All published articles of this journal are available on ScienceDirect.
Combination of Metformin and Laser against Head and Neck Cancer Cells In Vitro
Abstract
Introduction:
Metformin is a common drug for the treatment of diabetic patients. This drug has been shown to have anti-cancer effects. Metformin acts through the AMPK/mTOR pathway against cancer cells. We hypothesized that metformin, in combination with laser as photodynamic therapy (PDT), may increase cytotoxicity for cancer treatment. This study aimed to evaluate the anti-cancer effect and mechanism of metformin combined with laser irradiation on head and neck cancer cells.
Methods:
The anti-cancer effect of metformin was evaluated using head and neck cancer cells (HN5 cells) and oral cavity normal cells (HUGU) cells in vitro. Cell viability and phototoxicity were assessed with the MTT assay. Evaluation of protein expression of p-mTOR and mTOR genes was evaluated with the western blot method.
Results:
The results showed that the toxic effect of metformin on HN5 cells was dose- and time-dependent. Metformin had significantly more cytotoxic effects on cancer cells than HUGU normal cells. The laser increased the cytotoxic effect of metformin in HUGU and HN5 cells significantly (p<0.05). Metformin down-regulated the level of p-mTOR/mTOR protein, and despite the decreased ratio of p-mTOR/mTOR, the laser has affected the performance of metformin on p-mTOR/mTOR.
Discussion:
In this study, we used the laser without using a photosensitizer, but the simultaneous use of laser light and metformin increased the lethality of metformin on cells, which requires further research to determine whether metformin itself is a functional photosensitizer.
Conclusion:
: This study introduces the combination of metformin and laser as a new anti-tumor treatment that needs further studies.
1. INTRODUCTION
Cancer is a common disease in which uncontrolled and abnormal proliferation of cells occurs that can involve other nearby tissues [1-3]. Conventional cancer treatment is chemotherapy, which has many adverse side effects [4-6]. Photodynamic therapy (PDT) is utilized to treat various types of cancer [7-9]. In PDT, a photosensitizer is administered that irradiates the tumor and produces reactive oxygen species (ROS), which eradicate cancer cells [10-13].
The tumor may recur after PDT treatment, so this method is not always completely effective [14]. For energy production, normal cells depend mainly on mitochondrial oxidative phosphorylation, but cancerous cells switch to enhanced aerobic glycolysis, which is known as the Warburg effect [15]. The Warburg effect can affect the response to anticancer drugs [16-18]. Pyruvate kinase M2 (PKM2) expression is strongly increased in cancer and promotes the survival of cells. Also, phosphoinositide 3-kinase signaling by protein kinase B (AKT) can regulate the expression of glucose transporter (GLUT1) and increase glucose capture [19, 20]. In the metabolic reprogramming of cancerous cells, the mammalian target of rapamycin (mTOR) has an important role that is activated in most cancers [21].
A possible appropriate solution to prevent cancer recurrence in PDT is to combine it with other treatment methods. Because alterations in cellular metabolism happen in cancerous cells, the usage of agents that control the metabolism of glucose can be a great choice to decrease recurrence. In this regard, metformin is considered a promising drug in the treatment of cancer, which acts by inhibiting the AMP-activated protein kinase (AMPK)/mTOR pathway [22, 23]. Metformin inhibits tumor growth in skin cancer in obese and overweight mice by activation of AMPK and weakened signaling via mTOR complex and p70S6K [24]. Another study has shown that metformin increases the toxicity of 5-aminolevulinic acid-mediated photodynamic therapy in lung cancer cells [25]. Also, metformin combined with 20-tetra-sulfophenyl-porphyrin-PDT inhibits inflammation and angiogenesis of tumors in carcinosarcoma in rats [26]. Considering that metformin indirectly prevents the proliferation of cancer cells and reduces the risk of cancer, therefore, the simultaneous use of laser and metformin on cancer cells may have a favorable effect in the treatment of cancer patients. The present study evaluates the effect of the combination of metformin and photodynamic therapy without photosensitizer against head and neck cancer cells in vitro.
2. MATERIALS AND METHODS
2.1. Cell Culture
The HN5 cells (head and neck cancer cells) and HUGU cells (oral cavity; gingiva normal cells) were purchased from the Pasteur Institute of Iran. They were cultured in a supplemented DMEM medium (Gibco) with 10% FBS (Gibco) at 37 °C in an incubator with 5% CO2.
2.2. Photodynamic Therapy
For PDT, cells were seeded in 96-well microplates (10,000 cells/well). After 24 h, the cells were exposed to several concentrations of metformin (0, 5, 10, 20, 40, 80, and 160 mM) for 4 hours at 37 °C, then the cells were irradiated with laser light (630 nm) once for 60 seconds. After irradiation, the microplates were incubated for 72 hours until further examination. In this study, we didn’t use a photosensitizer.
2.3. Cytotoxicity
The MTT (3-[4,5-dimethylthiazol-2-yl]-2,5- diphenyl tetrazolium bromide) test (Sigma-Aldrich) was used for the evaluation of cell viability. The fresh MTT solution was added to wells and incubated for 4 h. Then, well's contents were exchanged with DMSO and absorbance was read at 570 nm. Cytotoxicity was calculated by [A]test/[A] control ×100 [27]. The experiment was repeated three times.
2.4. Western Blots
Western blot analysis was performed 72 hours after treatment of cells with IC50 concentration of metformin. For western blotting, RIPA buffer with Triton (Bioworld) with pH 7.4 was used for the preparation of cellular extract. The concentration of protein was evaluated by Bradford Protein Assay. The cellular extract was heated at 98 °C for 5 min. Electrophoresis was performed via acrylamide/bisacrylamide gel in denatured condition (SDS-PAGE) and moved to the membrane of polyvinylidene difluoride (PVDF) (Bio-Rad), was done via a Transblot Turbo system (Bio-Rad). The skimmed milk in 0.1% TBS-Tween 20 was used for blocking membranes and then incubated with antibodies. Then, the membrane was incubated with an anti-p-mTOR antibody (Ser 2481) (sc-2930,89), anti-mTOR antibody (30) and anti-GAPDH antibody ((sc-365062) (all Santa Cruz Biotechnology, Santa Cruz, CA), at 4°C overnight. Then, a secondary antibody conjugated with peroxidase (Thermo Fisher) was used for washing and incubating. The visualization of protein bands was performed by chemiluminescence (ECL Plus Kit) by the high-resolution ChemiDoc XRS system (Bio-Rad). The bands corresponding to the different proteins were evaluated via Image Lab software (Bio-Rad).
2.5. Statistical Analysis
Two-way ANOVA was used for analyzing the MTT test results, and One-way ANOVA was used for evaluating the western blot results, and differences were considered significant at p<0.05.
3. RESULTS
In this study, the MTT method was used to investigate the cytotoxicity of metformin on HN5 oral cancer cells and HUGU normal and non-cancerous cells. In this test, HN5 cells were exposed to metformin for 24, 48, 72 hours and 5 days. The results displayed that the toxic effect of metformin on HN5 cells was dose- and time-dependent (Fig. 1). HUGU cells were treated with metformin for 72 hours and 5 days. The results of the toxic effect of metformin on these cells are shown in Fig. (2). The results showed that metformin had significantly more cytotoxic effects on cancer cells than normal cells.
To evaluate the effects of Metformin and laser combination by light radiation energy intensities, HUGU and HN5 cells were incubated with different concentrations of Metformin. Cells were exposed to laser for 1 min. Cell survival was examined via an MTT test 72 hours after irradiation. The obtained results showed that with the increase in metformin concentration, the rate of cell survival gradually decreases (Figs. 3 and 4). The laser increased the cytotoxic effect of metformin in HUGU and HN5 cells significantly (p<0.05).
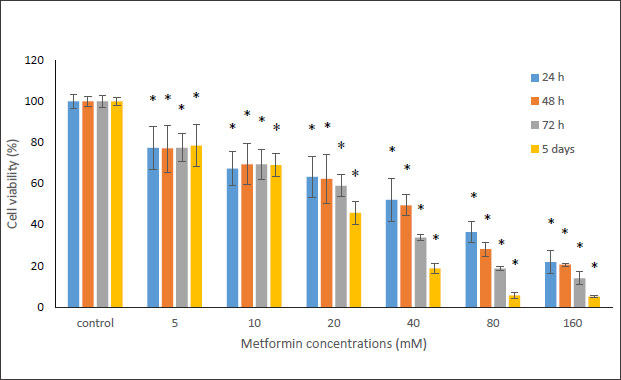
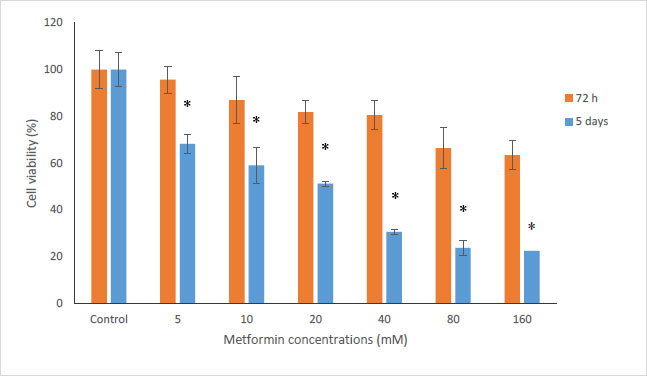
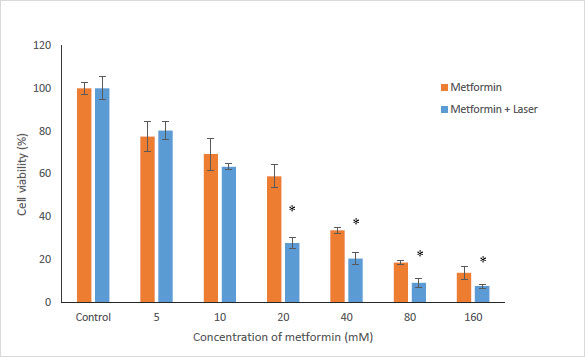
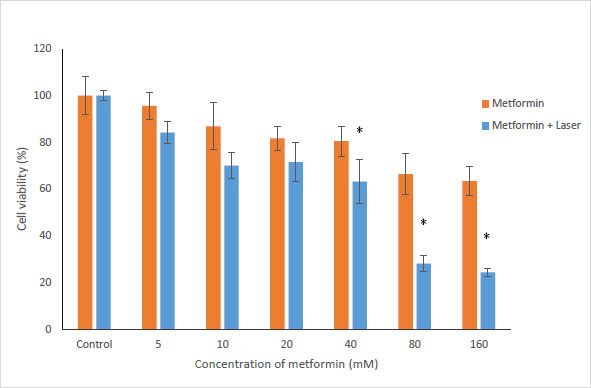
3.1. Effect of Metformin on AMPK/ mTOR Pathway in HN5 Cell Line
The anticancer property of metformin is multifunctional and it has the ability to control cancer through different pathways, one of which is the mTOR/AMPK pathway [28-30]. In this study, the expression of mTOR and p-mTOR protein after 48 hours of treatment on HN5 cells with MET, MET+ Laser was done by western blotting (Fig. 5a-d) shows that the level of p-mTOR protein compared to mTOR protein in the group treated with metformin showed a significant decrease (p <0.05), while in the group treated with metformin along with laser, the decrease observed is not significant compared to the control group.
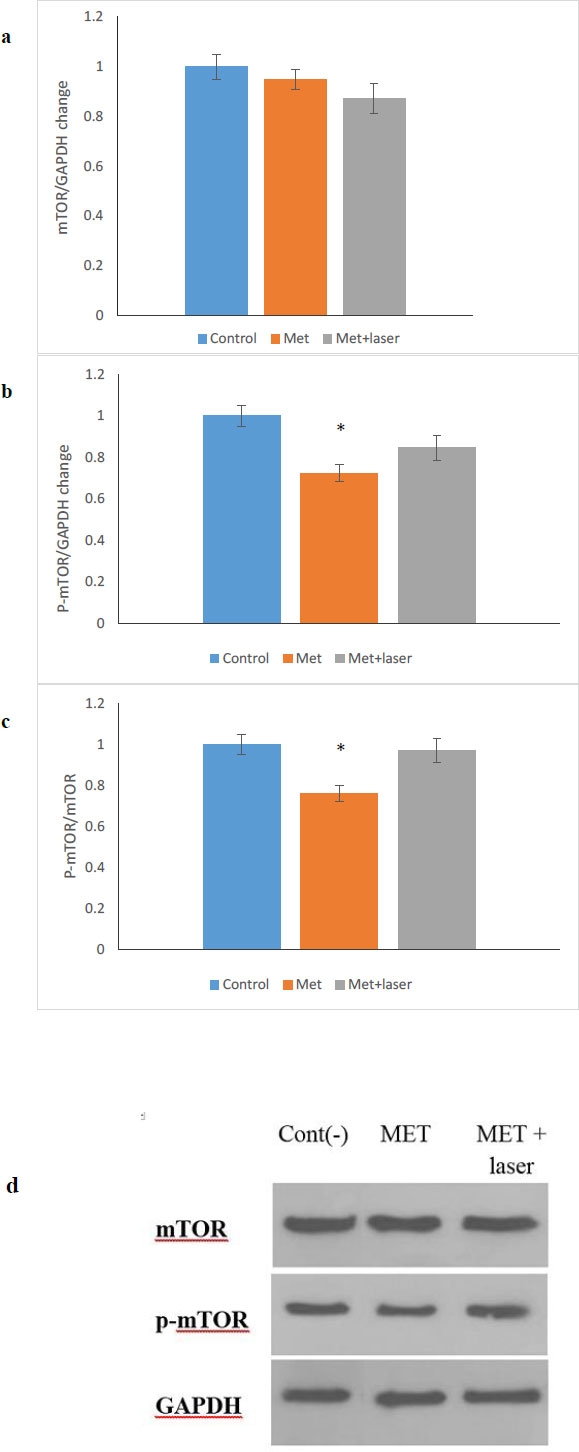
4. DISCUSSION
PDT is a new method of cancer treatment [31]. In PDT, photosensitizers can rapidly decompose intracellular oxygen under laser irradiation and keep cells in a hypoxic state [32]. Metformin is a classic hypoglycemic drug, and it also has an anticancer effect through the AMPK signaling pathway [33]. However, the anticancer effect of metformin is very low in conventional therapeutic doses. Studies have confirmed that metformin concentrations in the millimolar range in preclinical models have the greatest anticancer potential [34], which is more than that of serum metformin with conventional antidiabetic doses. Therefore, metformin combination therapy with PDT for PDT-resistant tumors can be utilized for maximum therapeutic efficacy [35].
In the present study, we showed the simultaneous use of laser and metformin significantly increased the death of cancer cells, which means that these two had a synergistic effect. This effect was observed in high concentrations of metformin (20-160 mM). Also, the simultaneous use of laser and metformin increased cell death in normal HUGU cells, but the effect was greater on HN5 cancer cells. In this study, we used laser without using photosensitizer, but the simultaneous use of laser light and metformin increased the lethality of metformin, which requires further research to determine whether metformin itself is a functional photosensitizer.
Several previous studies have elucidated the mechanism of metformin's anti-tumor effects. Metformin can decrease cancer cell proliferation by altering numerous signaling pathways, the most well-studied of which is the mTOR/AMPK signaling system [30, 36]. Metformin, as an anti-diabetic biguanide is presumed to inhibit mTOR via AMPK stimulation of TSC1/2-dependent or -independent mechanisms by increasing the cellular AMP/ATP ratio [37]. In this investigation, we found that metformin activated the mTOR/AMPK signaling pathway in HN5 cells consistent with previous studies [38].
CONCLUSION
It was proven that mTOR signaling regulates cell growth, proliferation, and metabolism, which has been linked to a number of human pathologies [39-41]. Also, mTOR signaling has a vital role in autophagy that is controlled by numerous factors such as mitogens, nutrients, and energy [42-45]. Our findings showed that metformin down-regulated the level of p-mTOR/mTOR protein, which was consistent with the results of other studies related to oral cancer [46-49] and other cancers [50]. According to previous studies, cells exposed to the laser can induce an increase in mTOR signaling [51]. As a result, in our study, despite the decreased ratio of p-mTOR/mTOR, the laser has affected the performance of metformin on p-mTOR/mTOR. Thus, this decrease was not significant in comparison to the control group. This study introduces the combination of metformin and laser as a new anti-tumor treatment, but it requires extensive studies for their anti-cancer effects in various types of cancer cells and in vivo studies.
LIST OF ABBREVIATIONS
PKM2 | = Pyruvate kinase M2 |
GLUT1 | = Glucose transporter |
mTOR | = Mammalian target of rapamycin |
PVDF | = Polyvinylidene difluoride |
ETHICS APPROVAL AND CONSENT TO PARTICIPATE
The ethics code was received from the Ethics Committee of Tabriz University of Medical Sciences, and all the steps were performed after obtaining the code (IR.TBZMED.VCR.REC.1400.436).
HUMAN AND ANIMAL RIGHTS
No humans/animals were used for studies that are the basis of this research.
DATA AVAILABILITY STATEMENT
The raw/processed data can be shared upon request from the corresponding author [S.S].
FUNDING
The Vice Chancellor for Research at Tabriz University of Medical Sciences provided financial support for this research that is greatly acknowledged (grant number 68889).
CONFLICT OF INTEREST
Dr. S.Simin Sharifi and Solmaz Maleki Diza are the Editorial Advisory Board Members of The Open Dentistry Journal.
ACKNOWLEDGEMENTS
Declared none.