Evaluation of Mechanical Properties and Antibacterial Activity of Nano Titania-Enriched Alkasite Restorative Material: An In Vitro Study
Abstract
Background:
A biocompatible additive with the ability to establish antibacterial action for restorative materials without sacrificing their physical properties is always in demand. Nano titania (TiO2 nanoparticles) was shown to have antimicrobial action against a wide range of microorganisms. Alkasite is a modern esthetic restorative material that has outstanding properties; however, it is deficient in antibacterial activity.
Objective:
TiO2 nanoparticles (NPs) were included in alkasite, and both mechanical properties and antibacterial activity of the new formulations were assessed.
Materials and Methods:
Nano titania powder was coupled to alkasite powder in proportions of 3 and 5% (w/w). There were 105 specimens made in total. Evaluation parameters were compressive strength, surface microhardness, surface roughness, water sorption and solubility, and antibacterial activity. One and two-way ANOVA were used for the statistical analysis, followed by Tukey′s test (p<0.05).
Results:
Both ratios of nano titania, 3 and 5% (w/w), significantly increased compressive strength, antibacterial activity against different pathogens, and decreased water solubility of alkasite (p<0.05). Only 5% (w/w) nano titania-modified alkasite exhibited significant decrease in water sorption (p<0.05). Conversely, an insignificant increase in microhardness and surface roughness was observed with both ratios, 3 and 5% (w/w) of nano titania (p˃0.05).
Conclusion:
Nano titania seems to be a very promising complementary additive to the alkasite restorative material, capable of generating considerable antibacterial effectiveness while also enhancing certain mechanical properties.
1. INTRODUCTION
Damaged teeth with impaired function and esthetic demand the selection of a restorative material that is capable of restoring these properties [1]. A variety of direct filling materials are available in the dental market; however, considering the properties of the material selected is a chief priority for best clinical performance and longevity of the restoration [2].
Dental amalgam has been used several years for its high mechanical properties, but due to its poor esthetic and mercury toxicity, priorities of selection have been directed to its alternatives [3]. Although glass ionomer cements (GICs) have a wide range of applications due to their favorable properties like good esthetic, fluoride release, and chemical bonding with tooth structure, their high solubility, low abrasion resistance, and low compressive strength are major shortcomings [3, 4]. Currently, Resin-Based Composite (RBC) restorative materials have been widely used and at least half of posterior restorations are made of these materials [5]. This popularity is highly relevant to their excellent esthetic, minimally invasive approach used and good retention. Despite these advantages, yet their polymerization shrinkage developed stresses and microleakage with the consequences of discoloration, recurrent caries, and pulpal pathology, might affect the longevity of composite resin. Consequently, different strategies have been developed to overcome these drawbacks [6-8].
Due to shortcomings of the most commonly used restorative materials for direct restorations, the demand for an alternative is highly considerable. Good esthetic, appropriate mechanical properties, fluoride release, simple application technique, and cost-effectiveness are the main objectives of the target product [9].
Alkasite (Cention N) is a recently introduced esthetic, resin-based, bulk filling material in retentive cavity preparations and it is an essential subgroup of the composite materials category. It is a self-cure restorative material with a light-curing option. The product has been offered in powder/liquid form and regarding its composition, it is UDMA-based resin. The material possesses certain advantages as radiopacity, the release of certain acid-neutralizing ions such as fluoride, calcium, and hydroxyl, high degree of polymerization, and cross-linking due to the inclusion of cross-linking methacrylate monomers combined with an efficient self-cure initiator. As well, the inclusion of stress-relieving isofillers in the recently developed bulk-fill material reduces the polymerization shrinkage stresses along with their consequences. As a cost-effective way to deliver a high-quality, predictable restoration with time-saving characteristics, alkasite could be a material of choice for posterior restoration [10, 11].
In addition to the potential to enhance the properties of current dental products, the application of nano-materials in the field of dentistry presents novel products with excellent properties. The extraordinary criteria of these nanomaterials are usually influenced by their nano-size. Different metallic nanoparticles have been investigated for their potential applications [12]. According to an increasing number of studies, nanomaterials have recently been proven to have novel dental caries prevention and therapeutic strategies, including the elimination and control of dental plaque biofilms, improving the antibacterial properties of dental products, and remineralization of initial dental carious lesions [13]. An example of these materials is TiO2 NPs that have been widely used in medicine and dentistry with different applications. Their ability to cause little pores in the bacterial cell wall, leading to an increase in permeability and cell death, has been reported [14]. Modification of composite resins by nanoparticles as a way for prevention of plaque accumulation and bacterial adhesion has been previously undertaken [15].
There is always a need for a biocompatible additive that has the potential to develop an antibacterial activity for restorative materials without compromising their mechanical properties. Based on the notable antimicrobial activity of nano titania and the recently introduced alkasite esthetic restorative material exhibiting excellent properties comparable to amalgam, but lacking antibacterial activity, this study aimed to evaluate the mechanical properties and antibacterial activity of nano titania-containing alkasite. The null hypothesis was that the incorporation of titania NPs into alkasite would neither change its mechanical properties nor induce antibacterial activity.
2. MATERIALS AND METHODS
An alkasite restorative material (Cention N, Ivoclar Vivadent AG, Liechtenstein, Lot number X46009) was blended with TiO2 NPs (Sigma-Aldrich, Germany, Lot number 718467, with average particle size 21 nm). Experimental powders were produced by mixing Cention N powder with TiO2 nanopowder in proportions of 3 and 5% (w/w). The powders were measured on a balance (TS4000, Ohaus, Pine Brook, NJ, USA) with a precision of 0.0001 g and mixed using a glass slab and plastic spatula. Unblended powder served as a control in all tests. A total number of 105 specimens were prepared for evaluation of compressive strength, surface hardness, surface roughness, water sorption and solubility, and antibacterial activity. Fifteen specimens were designated for each test and were classified into three groups (5 specimens each) as follows: I) control; II) 3% (w/w) nano titania-enriched alkasite; III) 5% (w/w) nano titania-enriched alkasite. Powder and liquid of each group were proportioned and mixed according to the manufacturer’s instructions and specimens were prepared in compliance with ISO standards for each test; ISO 604 for compressive strength, ISO 6507-1 for surface microhardness, ISO 4287 for surface roughness, and ISO 4049:2009 for water sorption and solubility.
2.1. Compressive Strength
Fifteen specimens were prepared using a cylindrical-shaped split Teflon mold with measurements of 4 mm diameter and 6 mm height. The compressive strength (CS), MPa, was measured using a universal testing machine (Model 3345, Instron Corporation, Canton, MA, USA) with a cross head speed of 0.5 mm/min and the equation:
CS = 4P/πD2
where, P is the maximum applied load at fracture (N) and D is the diameter of the specimen (mm).
2.2. Surface Microhardness
For evaluation of surface microhardness, 15 disc-shaped specimens were prepared in a split Teflon mold of 6 mm diameter and 3 mm thickness. Using a Vickers microhardness tester (HMV Microhardness Tester, Shimadzu, Japan), a load of 50 g was employed by the diamond indenter for a dwell time of 10 sec. For each specimen, five measurements were registered and the average value was recorded. The Vickers Hardness Number (VHN) for each specimen was determined and expressed in Kg/mm2.
2.3. Surface Roughness
In a split disc-shaped Teflon mold (8 mm in diameter and 2 mm in thickness), 15 specimens (5 specimens for each designated group) were prepared for surface roughness assessment using a surface profilometer (Surftest 211, Mitutoyo, Tokyo, Japan). Each specimen was scanned five times at distinctive locations. The stylus traversing length was 4 mm, and the surface roughness cut-off value was 0.8 mm. The measurement force and velocity were 4 mN (0.4 g) and 0.5 m s-1, respectively. The mean average roughness (Ra) was determined.
2.4. Water Sorption and Solubility
A split Teflon mold was used to make 15 disc-shaped specimens with a diameter of 10 mm and a thickness of 3 mm. At 23 ± 1°C, the prepared specimens were put in a desiccator with calcium sulphate. After each 24-hour cycle, the specimens were weighed using an electronic precise balance (TS4000, Ohaus, Pine Brook, NJ, USA). The calculations were repeated until the mass remained constant, and the results were reported (m1). Following that, all specimens were put in a glass vial containing 100 mL artificial saliva, prepared at the Faculty of Pharmacy, Mansoura University (Mansoura, Egypt) and composed of 0.75 g Sodium azide, 0.804 g potassium-monohydrate phosphate, 0.166 g calcium chloride, 0.059 g magnesium chloride, 1.02 g sodium chloride in 1 L of distilled water. The specimens were stored at 37°C in an incubator and weighed daily until a constant mass was reached and registered (m2). Before weighing, gentle drying with a filter paper was performed. Eventually, the specimens were redesiccated and reweighed one more time until they reached a steady mass (m3). The following formula was used to measure the water sorption (WSP) and solubility (WSL) (µg/mm3):
WSP= m2-m3/V
WSL= m1-m3/V
where, m1 is the specimen weight before immersion, m2 is the specimen weight after immersion, and m3 is the specimen weight after immersion and desiccation.
2.5. Antibacterial Activity
Using the agar diffusion test, 45 disc-shaped specimens were screened for antibacterial activity against Streptococcus mutans, Staphylococcus aureus, and Escherichia coli. In a Petri dish with a diameter of 100 mm, a 15 mL base layer of agar was combined with a 100 mL inoculum of each pathogen and stored at pH 7.5. Discs of the different groups were inserted in the plates. All plates were incubated at 37°C for 48 h. The diameters of the inhibition zones surrounding the specimens were measured in mm at three different points and the average value was considered to be the mean inhibition zone value (mm). Negative control specimens of sterile cellulose paper (8 mm) impregnated with Ampicillin (200 μg/disc) were used as a base line.
Finally, all specimens were stored in distilled water at 37°C for 24 h prior to testing. Data were analyzed by one-way ANOVA for all physical properties and two-way ANOVA for antibacterial activity with subsequent multi-comparison testing by Tukey (p<0.05). Statistical analysis software used was SPSS 12.0, SPSS, Chicago, Illinois.
3. RESULTS
3.1. Compressive Strength
Table 1 displays the mean and standard deviations (MPa) of the studied groups' compressive strength. A graphical representation of compressive strength results is shown in Fig. (1). The compressive strength of unmodified alkasite was the lowest (139.16±5.1), and when nano titania was added, the compressive strength was found to be proportional to the nano titania ratio, with the highest value (200.3±4.03) belonging to 5% (w/w) nano titania-enriched alkasite. ANOVA test found a significant difference (p<0.05) among the studied groups. Tukey′s test demonstrated that group III, 5% (w/w) nano titania-enriched alkasite, was significantly different from group II, i.e., 3% (w/w) nano titania-enriched alkasite. Moreover, a significant difference was observed between the control group, i.e., unmodified alkasite, and both group II, 3% (w/w) nano titania-enriched alkasite, and group III, 5% (w/w) nano titania-enriched alkasite (p< 0.05).
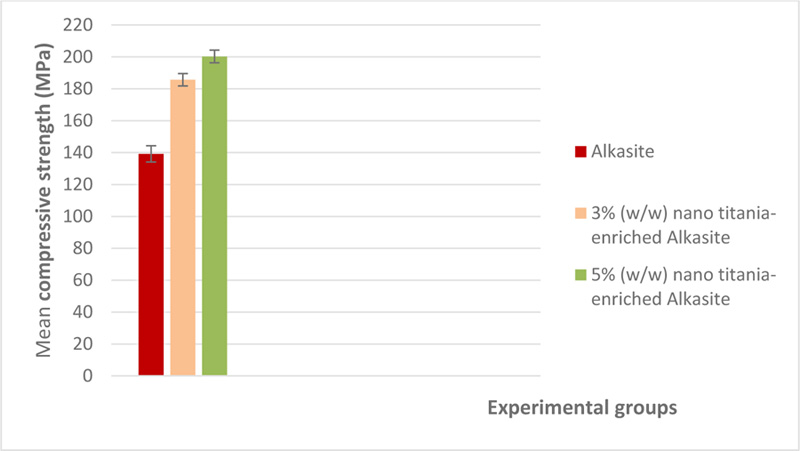
Group |
Compressive strength (MPa) |
Surface microhardness (Kg/mm2) |
Surface roughness (μm) |
Water sorption (μg/mm3) |
Water solubility (μg/mm3) |
I [Control; unmodified alkasite] | 139.16 + 5.1c | 49.92 + 1.46 | 0.051 + 0.003 | 8.76 + 0.7a | 73.7 + 2.18a |
II [3% (w/w) nano titania-enriched Alkasite] | 185.71 + 3.87b | 56.11 + 1.91 | 0.056 + 0.012 | 8.16 + 0.14a | 60.82 + 0.68b |
III [5% (w/w) nano titania-enriched Alkasite] | 200.3 + 4.03a | 63.37 + 1.27 | 0.060 + 0.007 | 7.4 + 0.4b | 47.67 +1.18c |
p value | <0.0001 | 0.52 | 0.27 | 0.0035 | <0.0001 |
3.2. Surface Microhardness
Mean surface microhardness and standard deviations (Kg/mm2) of the studied groups are shown in Table 1. A graphical representation of surface microhardness results is shown in Fig. (2). The control group, i.e., unmodified alkasite, exhibited the lowest value (49.92±1.46), while group III, 5% (w/w) nano titania-enriched alkasite, displayed the highest mean (63.37±1.27). One-way ANOVA revealed no statistically significant differences among the studied groups (p˃ 0.05).
3.3. Surface Roughness
Mean surface roughness values (μm) and standard deviations for the studied groups are shown in Table 1. Fig. (3) represents a graphical representation of surface roughness results. The results indicated that group III, 5% (w/w) nano titania-enriched alkasite, exhibited the highest mean value (0.060±0.007), while the unmodified alkasite displayed the lowest one (0.051±0.003). ANOVA test showed a non-significant difference among the three studied groups (p˃ 0.05).
3.4. Water Sorption and Solubility
The mean and standard deviation of both water sorption and solubility (μg/mm3) are shown in Table 1. A graphical representation of water sorption is displayed in Fig. (4). For water sorption, the unmodified alkasite exhibited the highest mean (8.76±0.7), while group III, i.e, 5% (w/w) nano titania-enriched alkasite, exhibited the lowest (7.4±0.4). ANOVA showed a significant difference among the studied groups (p< 0.05). Tukey test disclosed that both group I, i.e., unmodified alkasite, and II, i.e., 3% (w/w) nano titania-enriched alkasite, were significantly different from group III, i.e., 5% (w/w) nano titania-enriched alkasite. On the other hand, no significant difference was detected between group I, i.e., unmodified alkasite, and group II, i.e., 3% (w/w) nano titania-enriched alkasite. Regarding solubility, group III, i.e., 5% (w/w) nano titania-enriched alkasite, exhibited the lowest mean value (47.67±1.18), while the unmodified alkasite, exhibited the highest (73.7±2.18). ANOVA illustrated a significant difference among the studied groups (p< 0.05). Tukey's analysis showed that all the groups were significantly different from each other. The graphical representation of water solubility results is shown in Fig. (5).
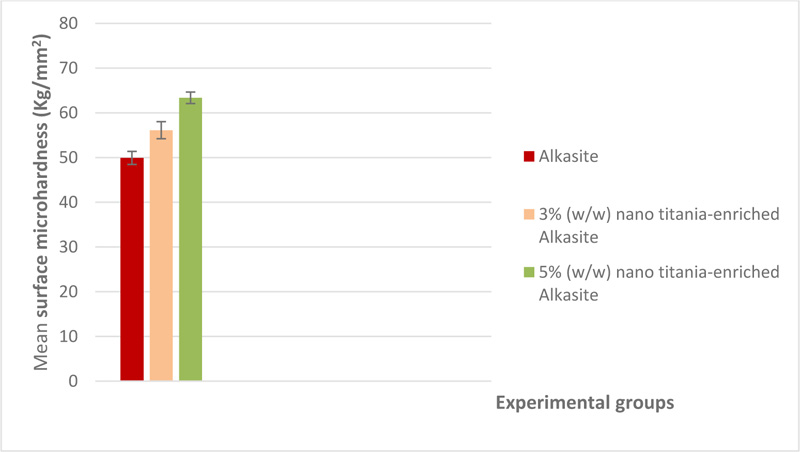
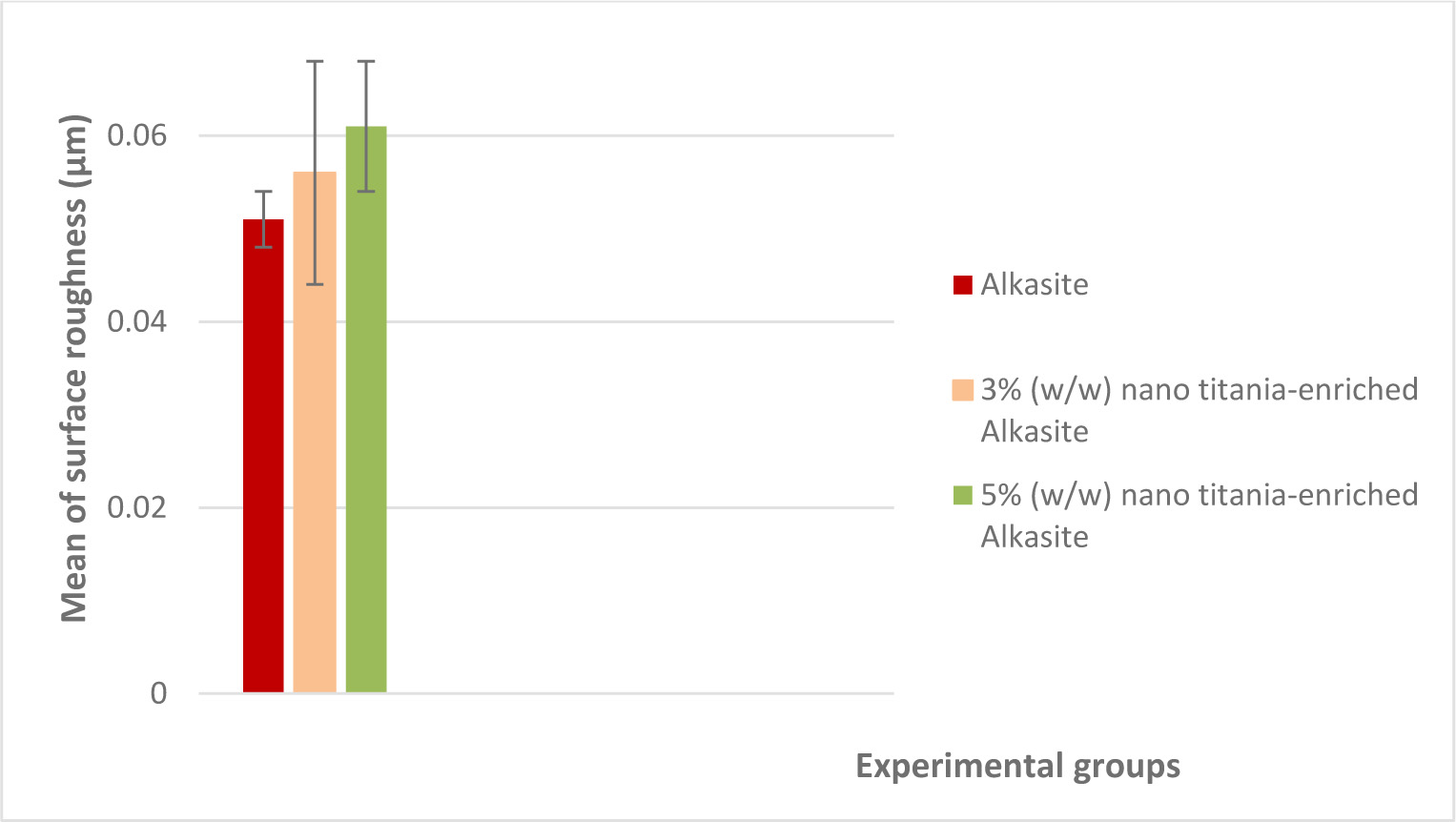
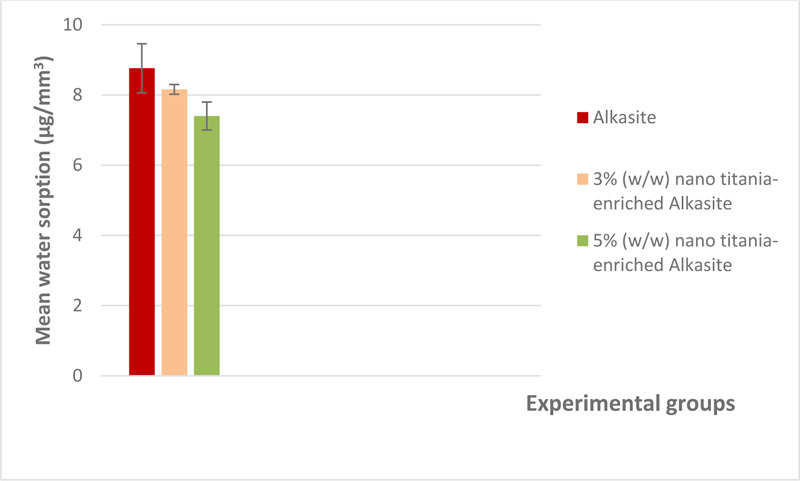
3.5. Antibacterial Activity
Table 2 presents the means and standard deviations of the inhibition zone (mm) of different groups. For all tested pathogens, group III, i.e., 5% (w/w) nanotitania-enriched alkasite, exhibited the highest mean (27.18±1.36, 6.6±0.55, 7.08±0.23 mm) against streptococcus mutans, staphylococcus aureus, and Escherichia coli, respectively, while the unmodified alkasite did not show any activity against the different pathogens. Two-way ANOVA indicated a significant difference among the groups (p< 0.05). Tukey's analysis showed that both group II, i.e., 3% (w/w) nano titania-enriched alkasite, and III, i.e., 5% (w/w) nano titania-enriched alkasite, were significantly different from the unmodified alkasite; also, there was a significant difference between group II, i.e., 3% (w/w) nano titania-enriched alkasite, and III, i.e., 5% (w/w) nano titania-enriched alkasite, for all tested pathogens (p< 0.05) (Figs. 3-4).
4. DISCUSSION
During the last decade, the demand for composite restorative material was attributed to its esthetic, however, the strength of such materials should also be considered during material selection to comply with the clinical scenario [16, 17]. Restorative materials should have properties comparable to those of the tooth structure to prevent either detrimental effects on the longevity of the tooth structure or premature failure of the restoration [18]. Compressive strength is one of the most important indicators for the material clinical performance under stresses during function and parafunction [2].
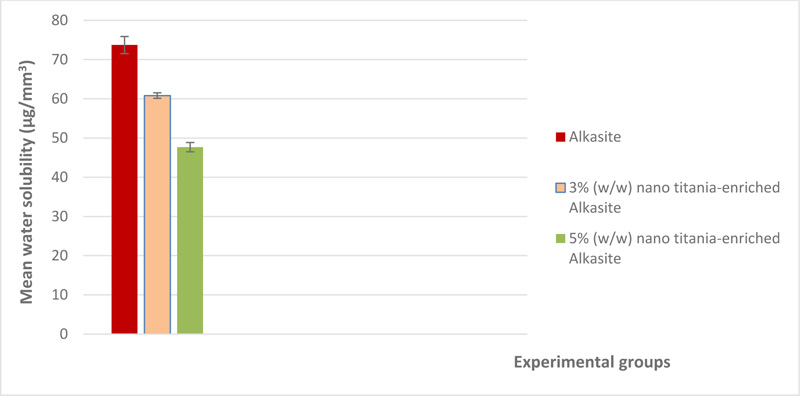
Group |
Streptococcus mutans mm) |
Satphylococcusaureus mm) |
E.coli ( mm) |
I [Control;unmodified alkasite] | 0c | 0c | 0c |
II [3% (w/w) nano titania-enriched alkasite] | 19.02 + 1.03b | 5.22 + 0.7b | 6.06 + 0.26b |
III [5% (w/w) nano titania-enriched alkasite] | 27.18 + 1.36 a | 6.6 + 0.55 a | 7.08 + 0.23 a |
p value | <0.0001 | <0.0001 | <0.0001 |
Difficulty to achieve complete sealing at the tooth restoration interface results in microgaps formation [19]. The restorative material should preferably exhibit antibacterial properties to prevent the consequence of the gap formation in terms of recurrent caries, postoperative sensitivity, and tooth discoloration [20]. On coupling an antimicrobial agent with the restorative material, its antimicrobial efficacy should be enhanced without having any adverse effects on its mechanical properties [21-24].
The use of nanoparticles in dentistry has recently sparked interest, owing to their ability to control the biofilm formation via exhibiting antibacterial, antiviral, anti-adhesive, and anti-inflammatory properties. In this perspective, the use of safe biocompatible nanoparticles may be of more noteworthy benefit. Among these metallic nanoparticles, TiO2 has been proved to have a potent antibacterial effect when added to a restorative material as glass ionomer cement with the fortification of its physicomechanical properties [25, 26].
In the current study, the incorporation of nano titania in different ratios into alkasite has significantly enhanced its compressive strength, with the most prominent effect detected with the ratio of 5% (w/w). This improvement may be attributed to a wider range of particle size distribution on adding TiO2 NPs, in which the small particles are liable to engage the small spaces between the polymer chains with more cross-linking. Another contributing factor that may support the compressive strength findings and explain the significant difference between 3 and 5% (w/w) is the higher density at the nanoparticles interfaces and the tendency of the nanomaterial to resist compression [27-29]. These results are fairly consistent with the findings of Elsaka et al., who concluded that both 3 and 5% (w/w) of TiO2 NPs were able to improve the compressive strength of glass ionomer cement [25].
Concerning surface microhardness, the addition of titania NPs exhibited an insignificant increase in the surface hardness of alkasite. This effect could be explained by the fact that TiO2 NPs are packed interstitially, resulting in denser surface quality with fewer voids, thereby generating more resistance to permanent indentation [25].
The increase in surface roughness observed on incorporating nano titania into alkasite was of no significance. The packing capacity of the TiO2 NPs into the microporosities at the alkasite surface seems to be controversial. Although the packing capacity was insufficient to reduce surface roughness, large agglomerations and distinct irregularities formation were still avoided. The wider range of nanoparticles size may be a sustaining factor for this judgment [27-29]. This outcome may be inconsistent with the finding of another study [30], which concluded that adding novel nano-hydroxyapatite-silica particles to glass ionomer reduced surface roughness substantially.
Considering water sorption and solubility, alkasite liquid contains urethane dimethacrylate (UDMA) resins with its ability to form a rigid network capable of absorbing less water and releasing more unreacted monomer [31]. It was recognized that nano titania addition decreased both water sorption and solubility on increasing the proportion of the nanoparticles, with the least sorption and solubility denoted by the ratio of 5% (w/w). Numerous factors are included in the validation of these results; one of them is that TiO2 nanofillers are water-insoluble, so they would decrease the solubility of the resin structure [32]. The reaction between the resin and the nanofillers would induce replacing the resin and diminishing water uptake through utilizing the most active sites, and accordingly, decreasing polarity. This influence is responsible for declining water diffusivity in the resin matrix of alkasite [33-35]. The result conforms to another investigation by Sideridou I et al., who noted that UDME-based resins were found to have less water sorption [31].
The antibacterial activity of nano titania had been investigated in several studies [36-38] and its potency has been shown against various pathogens. As a result, it has been merged with other materials. Nano titania is said to have the capacity to deactivate cellular enzymes and the microorganism’s DNA. Furthermore, it can cause pits in the bacterial cell wall, causing a decrease in the bacteria's resistance to it [39, 40]. The observations are compatible with the findings of Sabriye Pişkin et al., who concluded that the synthesized TiO2 nanoparticles exhibited antibacterial activity against E.coli, Staph.aureus, P.aeruginosa, C.albicans, and B.subtilis, and that TiO2 NPs could be considered as appropriate inorganic antimicrobial agents [41]. Another study confirmed the ability of MgO NPs to develop effective antibacterial and antibiofilm activity against cariogenic pathogens and to be very promising for dental applications; this finding may be in harmony with our outcome and may support the ability of different metallic nanoparticles to develop antimicrobial activity against different pathogens [42].
Finally, titanium dioxide as an inorganic additive has many promising properties as it is chemically stable, biocompatible and non-toxic [43, 44]. Despite the statement that TiO2 is allowed as an addition (E171) in food and pharmaceutical formulations, there is no strong evidence regarding its absorption, distribution, excretion, or toxicity when consumed. While it has a low acute toxicity profile for aquatic creatures, it has a variety of sub-lethal consequences on long-term exposure [45]. Accordingly, evaluating the immediate and long-term biocompatibility and cytotoxicity of the new formulation (nano titania-enriched alkasite) is of great importance and should be considered in further investigations.
CONCLUSION
Within the limitations of this analysis, it could be concluded that 5% (w/w) nano titania-enriched alkasite restorative material seems to be a very promising formulation. Nano titania supplement can generate considerable antibacterial activity against different pathogens. The modified alkasite exhibited an improved compressive strength and diminished water sorption and solubility while maintaining both surface microhardness and surface roughness. Further supporting studies are requested to assess the influence of this modification on other imperative properties, such as fluoride release, fracture toughness, and biocompatibility. Additionally, deeper analysis of the materials interactions that may influence the behavior of alkasite in service and the long term stability of the material after nano titania inclusion, and also the nature of ion release and its impact on long term antibacterial activity, should be conducted.
ETHICS APPROVAL AND CONSENT TO PARTICIPATE
This study was approved by the institutional research committee of Faculty of Dentistry, Mansoura University, Egypt ethical committee approval no. A02080120.
HUMAN AND ANIMAL RIGHTS
All procedures performed in the study were in accordance with the ethical standards of the institutional and/or national research committee.
CONSENT FOR PUBLICATION
Not applicable.
AVAILABILITY OF DATA AND MATERIALS
The data supporting findings of this study are present within the article.
FUNDING
None.
CONFLICT OF INTEREST
The authors declare no conflict of interest, financial or otherwise.
ACKNOWLEDGEMENTS
The authors are grateful to professor Dr. Nazem Abd El Rahman Shalaby (Faculty of Agriculture, Mansoura University, Egypt) for performing the statistical analysis.