All published articles of this journal are available on ScienceDirect.
Mechanisms Involved in Apice Closure of Pulpless Teeth – Literature Review
Abstract
Background:
Unfinished root formation has always offered challenges in endodontics due to technical difficulties and weakened teeth resistance during the lifetime of an individual. Pulp revascularization therapy appeared as a solution for apical closure and root maturation. The existence of oral stem cells involved in the process associated with traditional resident cells requires adequate blood supply given by induced controlled injury deliberately accomplished into the periapical zone.
Objective:
The aim of this work was to research, through literature review, the main mechanisms involved in the process of apical closure through the technique of pulp revascularization.
Conclusion:
Apice closure in pulpless teeth seems to happen as a result of professional intervention and biological activity. The success rate depends on the role of traditional local immune cells and stem cells associated with adequate blood supply to finish root formation.
1. INTRODUCTION
Unfinished open apices in immature teeth have always been a challenge for endodontics. Traditionally, the treatment of pulpless teeth with open apices can be summarized as a stimulated apexification accomplished with products based on calcium hydroxide and the subsequent filling with gutta-percha. Although this therapy has been used for years, in the near past, this particular procedure has been able to produce a mineralized barrier to obliterate the opening of the root apices. The root apices are left as a consequence of dentinal walls being weakened by thinness and square-shaped apices that demand a large number of gutta-percha cones, often jeopardizing the indication of intraradicular posts due to fracture risks. Inevitable as it was, thicker dentin walls had become the best outcome for teeth under those conditions.
Only in 2004 did Banchs and Trope [1] bring to the globalized world the Pulp Revascularization Therapy (PRT), with the premise of being biologically able to close open apices through the glaring possibility of revascularizing unfinished apices in pulpless teeth. Therefore, PRT has been recognized as a reality in modern times as a part of the therapeutic endodontic arsenal. Even though the mechanisms involved in hard tissue formation remain largely unknown, it is believed that PRT is a consequence of an intentionally injured apice, which causes bleeding that is responsible for triggering oral stem cells associated with local cells, such as fibroblasts and osteoblasts, located mainly in the periapical area.
Therefore, this work aimed to perform a literature review to bring to light the endodontic mechanisms of apical closure in teeth treated with PRT.
In order to facilitate the explanations of the steps to reach pulp revascularization, it is necessary to understand the immunological events demanded by the process and also comprehend the anatomy of the periapical area, which is able to offer a much effective immune response than the dental pulps when injured. The role of the oral stem cells present in the surrounding periapical tissues and within the dental pulps is also fundamental for the organism to mineralize the thin walls of the immature open apex, completing its anatomy. The substances used to induce pulp revascularization will also be introduced and illustrated so that practitioners can reproduce them.
2. LITERATURE REVIEW
2.1. Immunological and Histological Considerations
Injury in mammals is followed by inflammation and its vascular events necessary for the healing process. In this sense, tissue neo-formation and remodeling are necessary steps towards wound repair, requiring mainly blood flow to bring nutrients to the many kinds of cells involved in this process [2].
The damage caused by injury unleashes substances that act locally, such as platelets to halt hemorrhage and the clotting formation cascade, as well as cytokines, leading to the activation of both innate and adaptive immunities [3].
Afterward, competent immune local cells signalize the urgency for specialized cell recruitment and the inevitable need for specific substances with stimulatory or inhibitory effects. These substances are named cytokines whose family is vast, with contradictory and regulatory functions. It includes Interleukins (IL), Chemokines (CKs), Interferon (IFN), Tumor Necrosis Factor (TNF), Coloning-Stimulating Factor (CSF), and Growth Factor (GF) [4]. Cytokines then constitute an effective way of reaching healing due to their capability of promoting damage repair through cell recruitment through chemotaxis [5].
It has long been discussed in endodontics that the periapical tissues play important roles in halting bacterial invasion once the dental pulp is invaded and destroyed. However, bacterial penetration may occur before apical closure is finished, offering peculiar problems for the endodontist to obturate root canals in such situations. Regenerative Endodontic Procedures (REPs) were then developed, allowing mammalian organisms to complete their entire root formations through the stimulation of dentin-like tissue production, leaving at the end of the process a better-structured tooth with blood circulation within, where there was none before.
The procedure is accomplished with the aid of endodontic files intentionally introduced into the open apice, causing bleeding, usually induced with K-files. The absence of infection, induced controlled injury, and blood clot formation are the necessary basic events for inflammation and tissue repair. Once the file is inserted 2 mm into the open apice, bleeding will soon take place, followed by clot formation, whose aggregation time varies in humans from 4 to 8 minutes. Therefore, 8 minutes on average is the minimum period for blood clot formation. When bleeding is induced in teeth with periapical lesions, the very disarrangement will cause the influx of arterial blood full of nutrients and leucocytes right into the lesion, which will end up invading the root canal through the gap of the open apice [6].
The clot formed after the injury not only prevents blood from overflowing, it also works as a barrier against pathogens and foreign bodies while also providing a matrix for the deposition of different coming cells, which serves as growth stimulators in the following stages of healing. The repair process is trigged after injury, with the immediate release of cell mediators and low molecular compounds present in the disrupted blood vessels, along with the degranulation of the platelets, which will be the main cause responsible for the clot formation, basically composed of cross-linked fibrin along with extracellular matrix proteins such as fibronectin, thrombo- spondin and vitronectin [7, 8].
Current literature is full of examples of histological studies concerning regenerative endodontic procedures of immature teeth, the majority of them with necrotic pulp tissue and apical periodontitis. Many of such studies began with animal models, proceeding to human experiments. All of them have shown cementum-like and bone-like mineralized tissues in the canal space, as well as fibrous connective tissue similar to the periodontal ligament in the periapical area [9-12]. It is a fact that the approach of revascularization procedures causes an initial inflamed tissue, necessary for the cellular events coming forward.
After the initial inflammatory events, there is an invasion of reparative cells in the wounded tissues, mainly characterized by the arrival of neutrophils within minutes. These cells rapidly mount a non-specific response typical of innate immunity, attacking any sort of nonself substances and foreign bodies. Later, with the arrival of monocytes/macrophages and cells of other lymphocyte lineages (specific subsets of T cells and B cells), the integration of the innate immunity occurs with the adaptive one, characterized by the Antigen-presenting Cells (APCs) and lymphocyte lineages (specific subsets of T cells and B cells) to activate a more sophisticated immune response [13], also stimulating the production of protective and inflammatory molecules. The clinical response after induced controlled injury in the revascularization technique is somewhat painful and inflicts a sensation of dental extrusion due to the edema caused by inflammation.
When we observe the histological aspects of regenerative endodontic procedures concerning immature teeth that have been injured by pulp necrosis, what can be clearly observed is a reparative rather than a regenerative process [14, 15]. As a means to achieve repair, a new blood vessel formation will be necessary for blood supply in a region that needs cellular diversity, their integration, and above all, nutrients for their maintenance and survival, to rearrange the soft and hard tissues affected by the induced injury. The formation of new blood vessels involves two fundamental processes: vasculogenesis and angiogenesis [16].
Vasculogenesis is defined as the formation of blood vessels from angioblasts, which manifests only from the embryonic period [17], while angiogenesis is the formation of new blood vessels from pre-existing capillaries [18]. Vasculogenesis is expressed as a physiological process through which new blood vessels are formed from pre-existing functional vessels whenever it is necessary and when the organism can produce them [19, 20]. It is fundamental for wound healing and for the formation of granulation tissues, subdivided into two main types, namely: sprouting angiogenesis and intussusceptive angiogenesis. The former was the first identified form of angiogenesis, characterized mainly by growth factors produced to activate receptors on endothelial cells present in pre-existing vessels, followed by protease production, which degrades the basement membrane allowing endothelial cells to escape from the original vessel walls, originating new ones. The latter is considered a dynamic intravascular process that modifies the structure of microcirculation and pressure inside the vessels, spanning the lumen of the capillaries and modifying the branch angle, sometimes bifurcating them or duplicating the pre-existing less effective vessels, enhancing direct and collateral irrigations. The therapeutic potential of this intussusceptive angiogenesis is essential for the expansion of the blood net in areas that need increased blood flow for the intense metabolisms of the many cell types involved.
To enhance healing, the revascularization technique requires a defined sequence of events such as thrombus formation, inflammation, granulation tissue formation, fibroblast proliferation, matrix accumulation, and, finally, cell proliferation resulting in newly formed vessel formation consisting only of endothelial cells [21].
The strengthening of adhesions between endothelial cells and the recruitment of pericytic and smooth muscle cells are necessary to promote the stabilization and maturation of this newly formed vessel. This process is named arteriogenesis [22]. Angiogenesis, on the other hand, is regulated mainly by growth factors, enhanced by pro-angiogenic cytokines and neovascularization inhibitors [23].
The different cell functions that are thought to play important roles during angiogenesis may be categorized either in the activation phase or in the resolution one. The action phase comprehends initiation and progression, including increased vascular changes in permeability followed by extravascular fibrin deposition. As a consequence, the degradation of the basement membrane takes place, allowing cell migration and matrix invasion in the extracellular space, causing endothelial cell proliferation, enhancing the formation of the capillary lumen. The resolution phase is didactically characterized by the termination and maturation of the vessel. It also includes endothelial cell proliferation and diminished cell migration followed by membrane reconstitution, which finally enables the junctional complex maturation, forming a new blood vessel that originated from a thicker one, similar to a tree branch. This complex event is regulated simultaneously by stimulatory cells, most of which belong to the category of liberating growth factors since inhibitors can be divided into interferons, proteolytic fragments, interleukins, and protease inhibitors [24-26].
Inhibiting or stimulating blood vessel formation, the organism's urgency will define which of them is necessary for the moment, whether to form new blood vessels or to impede their formation. Therefore, cytokines are essential for “molecular communication” as they refer to the specific moment when it is necessary to stimulate angiogenesis or inhibit it. Positive regulation involves stimulation for angiogenesis, while negative regulations inhibit its formation. Examples of positive regulators are Epidermal Growth Factor/Transforming Growth Factor Alpha (EGF/TGF-α), Fibroblast Growth Factors (FGFs), Granulocyte-colony Stimulating Factor (G-CSF), Hyaluronan oligosaccharides, Interleukin-28 (IL-28), Platelet-derived Growth Factor-BB (PDGF-BB), Proliferin, Prostaglandins (PG), Tissue factor, Tumor Necrosis Factor-Alpha (TNF-α), Vascular Endothelial Growth Factor/Vascular Permeability Factor (VEGFs/VPF). Negative regulators are represented by Angiostatin, C-X-C chemokines, Endostatin, Hyaluronan, Interleukin-12 (IL-12), Interferons, Proliferin-related protein, Retinoids, Ribonuclease inhibitor and Transforming Growth Factor Beta (TGF-β) and Thrombospondin [27-30].
2.2. The Periapical Area
The periapical tissues surround the apical portion of the teeth, forming the periapical area. It contains a terminology named “critical zone” which comprises the apical root canal, the apical foramen, and its apical branches: apical deltas or foraminas, as well as accessory and secondary canals situated within 3-4 mm of the periapical zone or radicular apice. Its structural and anatomical components, the periodontal ligament and the apical alveolar bone provide the basic topographic and biological studies for the rational understanding of the subject [31].
The apical main foramen, larger than the foraminas, is usually located at the apical end of the root and may sometimes be situated laterally or even follow the curve of the canal in relation to the root curvature itself [32]. Different from the dental pulp, limited within rigid hard dentin walls, the periapical zone has ample possibilities of immune-modulated response, which needs an adequate blood supply to bring nutrients, immune-competent cells, and chemotactic substances such as cytokines and chemokines both responsible for the modulation of inhibitory or stimulatory effects. Therefore, an efficient new blood supply is necessary for healing.
Efferent blood vessels are veins carrying venous blood from the root canals, poor in oxygen and nutrients. In contrast, the afferent vessels that penetrate the canals are arteries demarcated from the main dental artery. After its penetration, the central arteriole goes towards the coronary portion and is divided into small branches (meta-arterioles and precapillaries) throughout the extension of the pulp. The smaller branches form the capillary network of the pulp, which ends in numerous venules, and all afferent vessels (except capillaries) have the smooth muscle to control their circulation. Together the afferent and the efferent vessels form the capillary bed responsible for the exchange of nutrients inside the pulp, constituting the capillary bed [33].
In normal conditions, the periapical zone hosts the normal resident cells expected to be found in its components: periodontal ligament fibroblasts, cementoblasts, osteocytes, and osteoblasts, circulating lymphocytes, monocytes, and few elements of the first line of immune defense, such as neutrophils. However, the apice is vulnerable to several stresses even in normal conditions in the absence of infection or inflammation due to the functional activity of mastication, thus causing the distribution of mechanical forces over the periodontium. When the infection is set, drastic changes occur, mainly represented by acute pulpitis, which, if untreated, may lead to a chronic pulpitis and finally to periapical lesions [34].
Cysts and granulomas are the main representative entities that result from the immune response of an individual to bacterial infection within the dental pulp, intending to contain the infection limited to the root canal. By scrutinizing the main resident cells in periapical lesions, a mixed infiltrate of T and B lymphocytes, along with polymorphonuclear leukocytes (PMNs), can be found effortlessly. Macrophages and natural killer (NK) cells are present, and also eosinophils and plasma cells have been identified [35-38]. As it can be observed by the different shapes and sizes of its resident cells, the periapical region contains elements from both sorts of immunities working in harmony with one intent: to protect the organism against microbial invasion. This immune response usually integrates four cell types, namely: T cells, B cells, NK cells, and Dendritic Cells (DC). Dendritic cells are also present and are known to be able to differentiate into osteoclasts when necessary [39-41].
The existence of infection can be more harmful when it affects open apice immature teeth simply because it causes dramatic changes capable of interrupting the normal biology of the cells responsible for finishing root formation. The consequence is usually teeth with open apices with or without periapical lesions. It is desirable, whenever it is possible, to offer an opportunity for biological apical closure, undoubtfully the most desirable outcome. Nevertheless, it is important to mention the role of the root canal decontamination before the other phases of root canal treatment or PRT procedures because if the necrotic material is pushed towards the periapical tissues, it might cause undesirable pathologies, such as apical pericementitis that may evolve into acute or chronic abscesses.
2.3. The Role of Oral Stem Cells in Apical Closure
The anatomy of the dental apice endows it with wide defensive biological tools ready to be activated whenever it is injured. It is an area of confluence and intense metabolism due to pulp and periodontal tissues, represented by the fibers of the Periodontal Ligament (PDL) with traditional dental pulp cells, alveolar bone and root cement, vascular components, and dental innervation.
Among the cells present in it, oral stem cells play a distinct role in producing hard tissue deposition, enough to finish apical closure. Therefore, three basic kinds of stem cells are pointed as the main responsible for helping apical closure, namely: Dental Pulp Stem Cells (DPSCs) [41, 42], Periodontal Ligament Stem Cells (PDLSCs)40 and Stem Cells from Apical Papilla (SCAPs) [43].
DPSCs were the first kind of oral stem cells found in the mouth. This is probably because dental pulps can be easily collected from extracted teeth, especially from those patients who undergo orthodontic therapy. These cells are more prone to originate neurons much more than other stem cells from the surrounding sites. Another characteristic is that it demands fewer ethical and much less polemic considerations than other stem cells do; and finally, they can be easily isolated and cultivated. All these facts gave rise to DPSCs to be exhaustively researched. These stem cells from the pulp can differentiate into odontoblasts, osteoblasts, adipocytes, and endothelial cells, under appropriate growth factors in vitro. On the other hand, in vivo, they seem to be able to build adult bone with Havers channels with appropriate vascularization and blood flux, they are also able to form dentin-like tissues [44]. DPSCs, in this sense, are imperative for the deposition of hard mineralized dentin-like structures responsible for the finishing of the apical third of the roots [45].
On the other hand, PDLSCs are also responsible for the biological frame of the dental apices and are maybe as important as DPSCs for the shaping and narrowing of the dental apice. Among other works which describe their capacity of diversification [46, 47], one specific study [48] strongly suggests that these cells can generate cementum/PDL-like tissue in vivo. They are multi-potential cells, and in vitro, they can differentiate into osteoblasts, cementoblasts, and adipocytes; while other in vivo studies show that these cells, after being transplanted into mice, differentiate into structures resembling bone-like tissue, cementum-like tissue, and even cartilaginous tissues [49].
Finally, ever since SCAPs have been identified as oral stem cells available, they have established a new protocol for their usage in endodontics, since, in vitro, they can differentiate osteogenically, odontogenically, and adipogenically. In contrast, in vivo, they do differentiate into odontoblasts and osteoblasts. Together, all these three kinds of oral stem cells reside in the periapical zone as a sort of cell storage, able to be activated to play their role in producing hard, functional tissues, provided that there is no infection impeding them [50].
2.4. Mineral Trioxide Aggregate (MTA) Considerations
Endodontics has a lot to thank MTA. Before its appearance, many of the complicated situations had much fewer possibilities to work out, a fact that caused lots of dental extractions all over the world. The alkalinity and the stimulus for the neoformation of osteoid tissue made MTA an interesting material capable of making a revolution in endodontic science. It appeared in dentistry in the early '90s, still as experimental material, developed by Torabinejad and his team. They had devised a suitable material to seal communications between the root canal system and the periodontium, more specifically, for perforations [51-53].
MTA has two basic clinical formulations: a white or gray powder composed of fine hydrophilic particles. Its main components are tricalcium silicate, tricalcium aluminate, tricalcium oxide, and silicate oxide. There are also small amounts of other mineral oxides responsible for the physical and chemical properties of the aggregate, such as bismuth oxide, responsible for its radiopacity [53].
Right after hydration with water, the powder forms a colloidal gel that solidifies in less than 3 hours [54], resulting in a hard structure composed of calcium oxide expressed in the form of discrete crystals, and calcium phosphate in an amorphous structure, with a granular appearance. After being approved by the “U.S. Food and Drugs Administration “, in 1999 [55], MTA started to be used for other clinical situations, expanding its indications to the following: direct pulp capping [56], pulpotomy [57, 58], filling in areas of internal and external resorption [59], cervical plug [60, 61], apical plug in teeth with incomplete rhizogenesis [62], canal filling [63] and then in 2006, as an intra-radicular reinforcement of teeth with incomplete rhizogenesis [64]. In most researches, MTA proved to be superior to other materials. Freshly prepared, MTA has a pH of 10.2, rising to 12.5 after 3 hours, and then remains constant. Literature shows initial and final setting times for the ProRoot MTA of 45 and 140 min, and 40 and 140 min [65]. Besides, it has a good sealing ability during in vitro studies. Such qualities made it an ideal material for inducing hard tissue formation due to its capacity to stimulate osteoblasts, as well as contamination control, due to its high pH. These properties have made MTA the most used material for pulp revascularization.
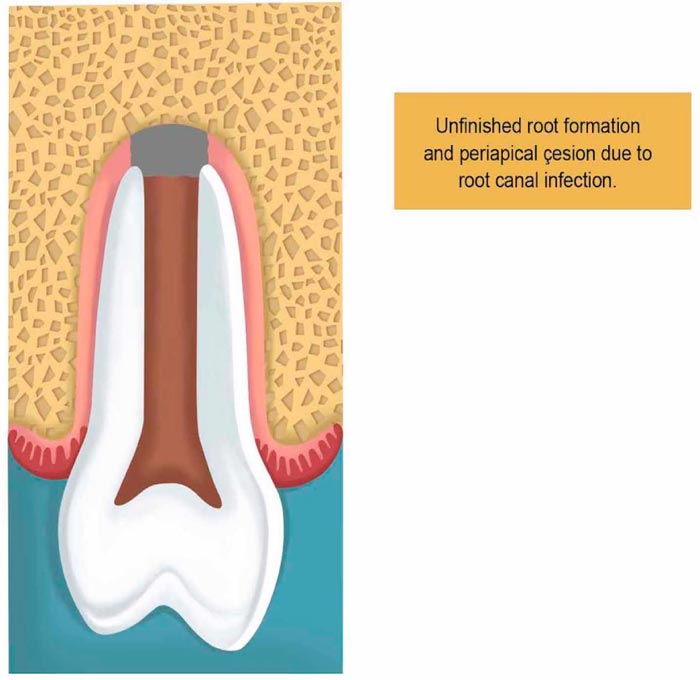
2.5. Pulp Revascularization Therapy
The traditional and practical indicated way to observe the evolution of root maturation of immature open apice teeth is the periapical radiograph, through which the dental professional can scrutinize the image in search of radiographic thickening signs in it. However, such signs should not be interpreted as regeneration of the so-called dentin-pulp complex. Histological confirmation is the only possible way to assure this interpretation. Nevertheless, the aim of the endodontic approach is the elimination of the infection present in the apical periodontitis. Therefore, the repair would be an adequate term. Infection control is accomplished mainly by irrigation, with the use of the three antibiotic pastes, and also by the placement of MTA into the cavity [49]. The alkalinity of MTA itself is a variable that impedes bacterial growth while stimulates hard tissue formation.
The procedure is indicated for teeth with unfinished root formation (Fig. 1). The clinical approach of PRT is made in two basic steps. The first includes local anesthesia followed by isolation of the tooth with a rubber dam to open the pulp chamber to find the canal entrance. Then the irrigation takes place with sodium hypochlorite (1.25% - 5.25%), and then it is removed with saline to allow minimum clinical aggression caused by chemical substances.
It is worth mentioning that no instrumentation is necessary. After irrigation, drying the root canal with paper cones is important for the insertion of the triple antibiotic paste, followed by the placement of a cotton ball obliterating the root canal entrance; and the sealing of the cavity with a temporary filling (Fig. 2).
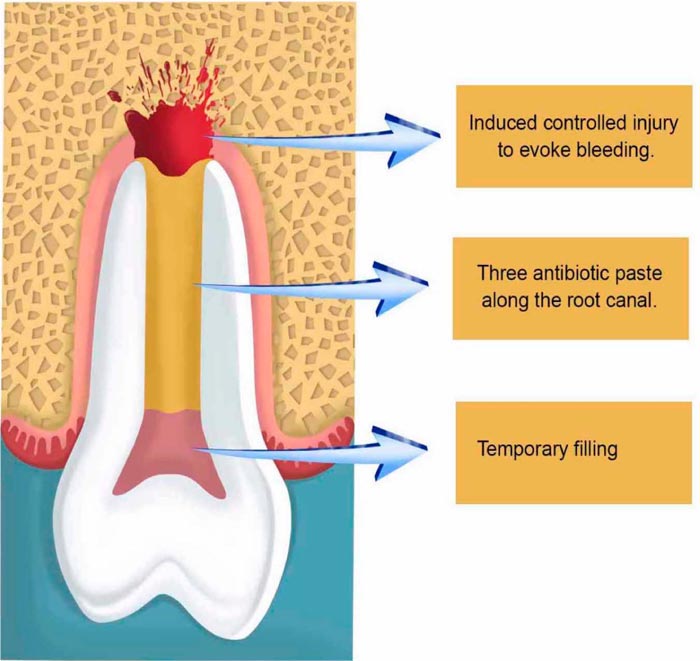
After two or three weeks, depending on the clinical situation including age and the width of the open apice, the second step takes place for the removal of the three antibiotic paste with sodium hypochlorite (1.25% -5.25%) and a final washing with saline to establish a free decontaminated way into the periapical zone, which will be injured with a k-file correspondent to the canal thickness to evoke apical bleeding, allowing new blood to flow as far as the cement-enamel junction. Afterward, the preparation of Mineral Trioxide Aggregate (MTA) takes place followed by its placement onto the blood clot to create a hermetic sealing within the root canal, followed by the obliteration of the cavity with a temporary filling (Fig. 3).
The tricalcium silicate, dicalcium silicate, tricalcium aluminate, tetracalciumaluminoferrite, calcium sulfate, and bismuth oxide offered by MTA enhance its radiopacity and allow better visualization of the biological events that will take place within. Over instrumentation to evoke bleeding is maybe the first essential step to induce apice closure in PRT [66]. This was made in the very first attempts to regenerate pulp tissues, followed by obturation with gutta-percha [67]. Nevertheless, the presence of necrosis has always been crucial for PTR for implying complex immune interactions, sometimes so intense as to cause acute abscesses that may put lives at risk. Infection decreases the possibility of healing in any part of the body. Considering that dental hard tissues have unique characteristics, such as not being replaceable in the majority of the situations, this constitutes a major issue. Therefore, the use of antibiotic pastes very short of the root apices not only allows direct action of the antibiotics involved locally, it also stimulates healing by disorganizing a microbiological ecosystem preestablished mechanically and chemically. Microorganisms within the root canals are diversified and vary due to many factors, including the oral microflora, which sometimes carries bacteria and fungi altogether. With this in mind, polyantibiotic pastes using a mixture of neomycin sulfate, polymyxin B sulfate, bacitracin, and nystatin began to be used, also associated with iodoform within the root canals, causing a thicker deposition of minerals and so enhancing apical barrier formation in open apices of pulpless teeth [68]. As time passed by, researchers used many kinds of substances to be in close contact with the periapical region. Some works used the host´s vascular cells, giving rise to two clinical modalities of approach: apexification and revascularization. Both therapies use the cells of the organisms already present in the periapical region, but with different outcomes: apexification uses calcium hydroxide, which in general takes a long time to form a rather squared mineral barrier leaving the root apice with thin, fragile walls. Revascularization therapy, on the other hand, promotes further apical development, which results in thicker reinforcement of the dentinal walls in the periapical zone due to the gradual deposition of mineralized tissues. Intracanal medication used in the different commercial pastes used in PTR varies in composition; calcium hydroxide pastes associated with metronidazole, ciprofloxacin, and minocycline have been used. Other authors have used Platelet Rich Plasma (PRP) associated with metronidazole, ciprofloxacin, and minocycline (Fig. 4).
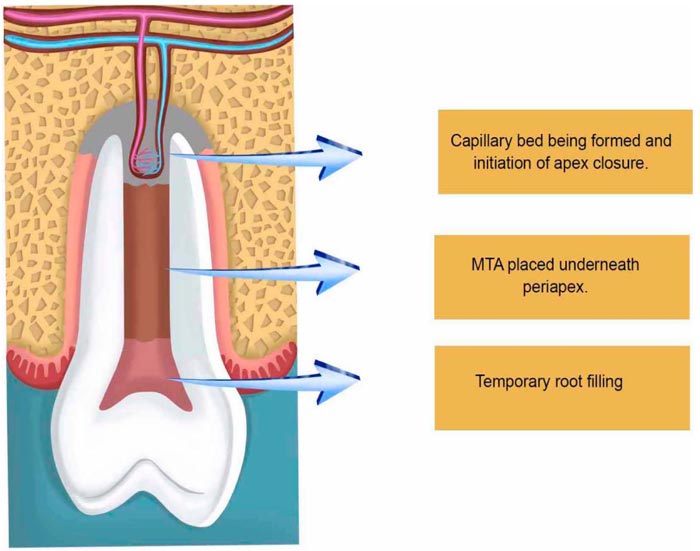
Some have used calcium hydroxide with IRM, while others have used the three mentioned antibiotics with only saline, while some other formulations included nystatin to combat fungi [69-72]. More important than placing antibiotic pastes is the control of contamination during the biopreparation of the root canals. Adequate cleaning and disinfection of the root canal space itself promote an ideal microenvironment for the placement of bioceramics to induce the production of mineralized tissue caused by the synchronized interactions of DPSCs, PDLSCs, and SCAPs [73-76]. The injury inflicted into the periapical tissues seems to allow the entrance of mesenchymal stem cells coming from the surrounding alveolar bone, flowing with the bloodstream into the canal space [77, 78], activating traditional resident cells such as fibroblasts and osteoblasts, to finish the conic shape of the dental apice [79, 80].
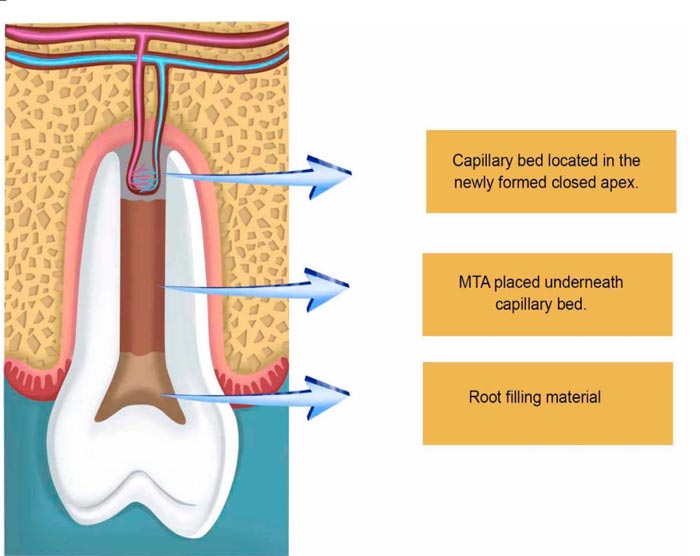
3. DISCUSSION
Immature nonvital teeth have presented a real challenge for endodontics because of their root canal walls diverging apically, making the preparation of an apical stop impossible to be accomplished and the result of the treatment unpredictable [81]. The need to solve this problem led to apexification therapies, whose aim was to create an apical stop that might be filled with gutta-percha in traditional endodontic obturation, or even better, with biological hard tissue formation in recent years. More recently yet, the rise of regenerative procedures in the health area has led these procedures to be practiced in graduation clinics enabling the newly formed dentists to accomplish them at the very beginning of their professional lives. In Brazil, this lone fact may save a great number of teeth that are removed unnecessarily every day in the poor peripheral population.
Three fundamental steps are necessary for the successful regeneration of the dental pulp: absence of infection in the root canal space; stimulation of the host dental stem cells; and finally, appropriate coronal seal. They constitute the basis for pulp revascularization and apical closure. They can be contemplated with traditional periapical radiographs, or with the aid of cone beam tomography for better visualization and assessment of the whole process until healing is obtained. Revascularization results in a more resistant, anatomically formed tooth that may support the stress of the mastication with fewer risks of root fracture, as well as fewer risks of bacterial invasion because of the biological mineralized barrier formed.
During the development of the regenerative procedures, two modalities of approaches have appeared to accomplish apical closure: apexification and apexogenesis. The difference between them lies in the vitality of the pulp affected. Apexogenesis happens when dental pulps are vital, while apexification is a treatment applied for necrotic pulps in order to induce apical closure [81]. The use of MTA, featured in this paper, was due to it being the first bioceramic to be used successfully to stimulate hard tissue formation in the apical area. However, nowadays, regeneration of immature necrotic permanent teeth seems to be reached by using other bioceramics under researches.
Contradictory as it may seem, the existence of open apices seems to enhance the success rate of pulp revascularization. They make it easier for blood supply and angiogenesis formation, offering the necessary physical and biological space for repair, mainly because of the interactions between different kinds of progenitor and traditional cell types mentioned above involved in the process.
Blood is an essential component when the periapical region is affected. Therefore, when it invades the unfinished root end, nutrients such as amino acids, vitamins, and minerals are offered for all cells present in the canal space and periapical tissues. It is a fact that almost all cells in the human body are close to a blood vessel. This vascular disposition is necessary for inflamed tissues to grow beyond 100-200 µm, known as the diffusion limit of oxygen [82]. Due to the high consumption of nutrients that the healing process demands, only cells close enough to a distance of nearly 200 µm seems to have access to sufficient nutritious particles, which are supplied by bloodstream by process of mass transport in mixtures that contain local concentration variations for cellular consumption [83]. Therefore, the induced control injury is maybe the most important step to reach pulp revascularization and root end formation.
With or without professional intervention, an adequate blood supply makes all the difference as to which way healing will evolve. However, pulp revascularization and root end formation in necrotic teeth are regenerative procedures that can not be reached without professional intervention. It is worth mentioning that the recruitment and differentiation of the mesenchymal stem cells from the periapical area depend essentially on the induced controlled injury, deliberately featured in Fig. (2). It will promote blood clots, enabling the stem cells to migrate into the root canal, promoting newly formed tissues in the canal space [84].
The contribution of the pastes applied into the root canal and short of the apice seems to play an important role, not only to combat infection but also to cause chemical and mechanical stimulation in the surroundings of the open apice, enhancing the apical closure, especially when calcium hydroxide was used in different formulations [74, 75]. Their obligatory use, however, has been questioned recently. Calcium hydroxide has been used for years in apexification procedures, with regular replacements, and is able as an alkaline substance to decrease osteoclastic activity while stimulating osteoblast formation. However, some new researchers are now focusing on one single session for revascularization therapies that might not use pastes between sessions.
The gradual closure of the apice will show a narrowed conic canal space that will lead to a closed foramen and the absence of periapical lesions, indicating the final healing process. This is probably reached by the orchestrated work of the DPSCs, responsible for the apical third of the newly formed canal space. PDLSCs are responsible for the establishment of the periodontal fibers that anchor the newly formed apice to the alveolar bone. In this way, the stress caused by mastication will stimulate the strength of the fibers, reinforcing the periodontal membrane in the regenerated part of the teeth. This is particularly important for prosthetic and functional reasons. It is not unusual that teeth undergoing revascularization procedures need reinforcement offered by intracanal posts, which would be too risky in teeth with open apices.
The finalization of the process is therefore orchestrated by SCAPs and bone marrow stem cells from the alveolar bone since the induced bleeding will transport these bone marrow stem cells directly from the injured bone into the open lumen of the unfinished root canal, originating bone or dentin-like structures [76, 77]. PDLSCs also share this specific microenvironment with other traditional immune cells with fibroblasts and epithelial cell rests of Malassez (ERM), and the constant stress may trigger both kinds of cells. Together PDLSCs and ERM cells also seem to be able to help tissue regeneration [78, 79].
As root formation continues, the insertion of new blood vessels originated from the blood clot will remain as the vessels responsible for the blood supply necessary for the intense metabolism therein. Depending on the size of their lumen, it will originate later on the arteries and veins present in the main canal (foramen) and accessory canal (foraminas), as hard tissue deposition will occur around them, forming the apical deltas [27, 32]. Natural as it seems, age plays the difference in the speed of root formation; therefore, teenagers generally respond faster due to being more biologically active.
CONCLUSION
Pulp revascularization is a regenerative procedure that depends on professional intervention to trigger biological activity in order to reach revascularization that will lead to apice closure. The teeth treated under this technique develop much more structured and resistant dentinal walls, enhancing long-term functional and anatomical characteristics. The success rate depends on the role of traditional local, immune, and stem cells, associated with adequate blood supply to finish root formation.
CONSENT FOR PUBLICATION
Not applicable.
FUNDING
None.
CONFLICT OF INTEREST
The authors declare no conflict of interest, financial or otherwise.
ACKNOWLEDGEMENTS
Declared none.