All published articles of this journal are available on ScienceDirect.
Physico-chemical and Mechanical Assessments of a New 3D Printed PMMA-Based Acrylic Denture Base Material
Abstract
Background
Three-dimensional (3D) printing is progressively being applied in the dental arena due to its time-saving potential and low cost, especially for the digital preparation of acrylic resin denture bases in the treatment of edentulism.
Objective
This study investigated the flexural strength, surface properties, water sorption, and solubility of a new poly (methyl methacrylate) (PMMA) resin denture base fabricated with a 3D printer (test group) and compared it with a conventional heat-cured resin (control group).
Materials and Methods
Sixty cuboid wax samples were prepared from two groups, with a thickness of 4 mm and dimensions of 10×80 mm. Then, half of the samples in each group were thermocycled to determine the strength, surface properties, water sorption, and solubility of the samples. Two-way ANOVA and independent t-test were used at a significance level of 0.05 after the normality of data in groups was tested with the Kolmogorov-Smirnov.
Results
Based on the obtained results, the flexural strength was higher in heat-cured resins than in the resin fabricated with the 3D printer. However, the flexural strength values in both resins were clinically acceptable (65 MPa) based on ISO standards. There was no significant difference in the mean flexural strength in the presence or absence of thermal stress for both groups. The layered structure was observed in the samples fabricated with two groups. However, the layering structure disappeared in all cases after polishing. The layering structure had no differences in the presence or absence of thermal stress. Mass change over time was observed for all groups. In the water sorption phase, the mass increased rapidly in the first 7 days, and then the increase rate decreased until it reached an equilibrium on day 21. In the desorption phase, the mass decreased steadily in the first 7 days, and then reduction was continued until equilibrium was obtained on day 21 of the dewatering process.
Conclusion
The 3D printer samples had greater water sorption, and no differences were found between the solubility of 3D printer samples and heat-cured samples. Heat-cured resin exhibited enhanced water sorption after thermal cycling, and 3D-printed materials displayed no significant change.
1. INTRODUCTION
Edentulism is a medical condition described as partial or complete toothlessness. Tooth loss usually occurs with symptoms, such as spontaneous pain or unexplained pain. This is followed by tooth sensitivity, which results in edentulism [1].
Prosthetic dentistry, prosthodontics, is a branch of dentistry that deals with providing substitutes for the missing parts of teeth, bones, gums, or facial structures. There are some choices accessible to replace missing teeth, including dental implants, dental bridges, and dentures [2].
Dentures are artificial teeth, removable or stable, applied as a partial or complete set to treat edentulism. Numerous different resins are applied in prosthetic dentistry based on their composition and processing techniques for the dentures. All types of resin materials have an acceptable aesthetic and functional effect. Removable dentures are extensively applied to replace missing teeth [1-3].
Polymethylmethacrylate (PMMA) has been used to fabricate heat-cured denture bases since the 1940s [1]. Its advantages include low cost, simple fabrication and repairability, low weight, acceptable aesthetics, biocompatibility, and ease of polishing [2]. However, some of its disadvantages include exposure to microbial occupation in the oral environment, high polymerization shrinkage, allergic reactions to residual monomer, mechanical degradation of its possessions over time, and low wear resistance to intraoral and extraoral stresses. New materials and techniques have been presented to solve these shortcomings [3, 4].
Indeed, dental acrylic resins are macromolecular composites. They can be from natural or synthetic sources that include monomer units. Monomers are single simple molecules that are linked together to form polymers. Macromolecules, such as polymers, are prepared by a polymerization process, which is allocated into two kinds of condensation and addition [4]. The possibility of using other materials with similar, perhaps better, mechanical features, used for similar purposes and which often appear to have similar characteristics should be assessed. For example, Reda et al. conducted a short review on the applications and clinical behavior of BioHPP in prosthetic dentistry [3].
Contemporary breakthroughs in digital dentistry have impacted the production of prostheses. Digital dentistry has developed many practical processes in some branches of dentistry since its introduction in the 1980s [5]. In 1994, the first attempt was made to create a fully removable dental prosthesis with the CAD/CAM technique [5, 6]. However, the digital fabrication of dentures was patented by Goodacre et al. in 2012 [7].
There are two digital methods to fabricate removable dentures: subtractive and additive [8]. The denture base is milled with a pre-polymerized resin using the subtractive method. Prefabricated or milled dentures are subsequently attached to the base, depending on the technique. The most important disadvantage of the subtractive method is that the bulk of the material remains unused and is discarded after fabrication [8].
The other treatment approaches are not possible owing to numerous contraindications. However, this type of material is still preferred by most patients and dental professionals for removable prosthetic restorations [9]. Modern additive manufacturing (AM) is currently improving clinical and laboratory procedures for fabricating removable prostheses [10].
In recent years, some studies have stated three-dimensional (3D) printing of PMMA denture base resins with a goal of attaining comparable mechanical possessions of conventional PMMA [11]. Numerous aspects associated with the resin composition, the kind of printer, the operating standards of the printer, and the post-curing process can affect the parts of the printed product [12]. The clinical routine of the prosthesis is limited by the mechanical properties of its materials. Prostheses are exposed to flexural pressures causing internal stresses by the masticatory system, which, in turn, results in periodic deformation of the polymer base, eventually leading to crack formation and fractures. In a study on the flexural strength and surface properties of CAD/CAM-based prepolymerized PMMA polymers used to fabricate 3D-printed full dentures, the flexural strength (FS) and hydrophobicity of CAD/CAM-based polymers were higher than conventional heat-polymerized PMMA while their surface roughness was similar [13]. Recent studies have reported similar findings on the high flexural strength of milled resins [14, 15].
In 3D printing, the fabrication direction (layer orientation) affects the mechanical properties of dental restorative materials since the nature of additive layers in additive manufacturing technology might initiate crack propagation, leading to the structural failure of printed materials [16].
An in vitro study reported that layer orientation affects the compressive strength of 3D-printed composite materials. By applying a force perpendicular to the direction of the layer, a vertically printed material exhibits higher compressive strength than a horizontal one [17]. In addition, it is worth realizing that the bond between layers is weaker than the bond within the layer because of residual stresses and accumulated porosity during UV polymerization and material shrinkage [18].
Ji Suk Shim et al. studied the effects of printing orientation on printing accuracy, surface features, flexural strength, and the microbial response of 3D-printed denture base resin in an in vitro study. The samples printed with a 0-degree orientation had higher flexural strength than those printed with 45 and 90 degrees [19].
This study investigated the flexural strength, surface layering, water sorption, and solubility of a poly (methyl methacrylate) (PMMA) resin denture base fabricated with a 3D printer and compared it with a conventional heat-cured resin.
2. MATERIALS AND METHODS
Thirty samples in each group [13], with dimensions of length: 80 mm, width: 10 mm, and height: 4 mm, were prepared according to ISO178 standard and assigned to two subgroups of control [n=15] and thermocycling [n=15] [20].
To prepare heat-cured polymethylmethacrylate samples, identical cuboid wax samples were made using a metal mold (Cavex Holland BV, RW Haarlem, Nether- lands). Then, heat-cured Meliodent acrylic resin samples (Kulzer, Germany) were made using the standard technology. Plaster was poured into a flask with a cuboid wax mold. After the plaster set, the flask was opened, and the wax was completely removed. Then, the denture base resin was placed inside the mold. Afterward, the flasks were placed under a 1250-kg pressure for 5 minutes, and by maintaining the pressure, the flasks were located in an appropriate polymerization unit. After polymerization, the flasks were cooled at room temperature, and finally, the cuboid acrylic blocks were meticulously removed from the flasks.
3D-printed cuboid samples, with dimensions similar to heat-cured ones, were designed by an open-source CAD software program (FreeCAD v. 0.15; FreeCAD Team) before being fabricated and then saved in an STL file (standard tessellation language) and fed into the 3D printing software program (PreForm Software 2.15.1; Formlabs). The samples were made using 200 grams of a 3D-printing fluid resin for denture fabrication (Detax, FreePrint 2.0) using a 3D printer (Luminous 4X, Tabriz, Iran) manufactured by Iranian Megatronics Company. The samples were fabricated with a zero-degree orientation, with a layer thickness of 100 µm.
The supporting components of samples were cut by a low-speed handpiece (Marathon Handy 700; Saeyang) and placed in a container with 99% isopropyl alcohol (FormWash, Formlabs, USA) for 2 minutes to remove the remaining surface monomers. Then, the samples were polymerized by UV light (FormCure, Formlabs, USA) according to the manufacturer's instructions. All the samples were polished with 500 - and 1200-grit silicon carbide paper [21]. The finishing process was performed by one operator.
The final dimensions of samples were measured with a digital caliper (IP54; Shan) with an accuracy of ±0.1 mm by one operator.
All the samples were immersed in 37°C water for 48±2 hours before thermocycling. Then, the thermocycling subgroup samples underwent a thermocycling protocol (5000 cycles, 5/55°C, each cycle = 5 minutes) in a thermocycler (MTE-101; MOD dental; Esetron Smart Robotecnologies, Ankara, Turkey).
2.1. The Flexural Strength
A three-point flexural strength test was conducted using a versatile testing machine (Static Universal-Testing Machine, SeriesLFM-L. walter+bag. Löningen, Switzer- land). The distance between the two supports of the machine was 53 millimeters, and the testing speed was set to 0.5 millimeters per minute based on the model's dimensions as per prior studies [21]. The load at the point of fracture was reported in Newtons, and the flexural strength was calculated in Megapascals (MPa) using the formula FS = 3PL/2 Wt2.
2.2. The Surface Morphology
The fractured surfaces of the samples from the flexural strength test were utilized to examine their surface morphology. Initially, the surface morphology of all groups was observed using an optical microscope (Olympus CX23, Japan) with a 10x magnification before and after polishing. Subsequently, a scanning electron microscope (SEM, TESCAN-USA) was employed for a more detailed morphology assessment. The samples were mounted on a stub and coated with a thin layer of gold. Images were captured at an acceleration voltage of 10.0 kV at a magnification of 10.0x.
2.3. Water Sorption and Solubility
Water sorption and solubility measurements were conducted following the ISO 20795-1:2013 standard for Denture Base Polymers. Prepared samples were placed in a desiccator containing fresh dry silica gel at 37 ± 2 °C for 1 day. Subsequently, the samples were transferred to another desiccator at room temperature, also filled with freshly dried silica gel, and weighed after 1 hour. The procedure was continued daily up to the alteration in weight between each weighing that did not exceed 0.2 mg, establishing the baseline mass (m1). The volume (V) of each sample was determined by measuring its diameter and thickness. Following this, the samples were kept in artificial saliva at 37 ± 2 °C. Each sample was dried after removal from the artificial saliva, and weighing continued until the change in weight did not exceed 0.2 mg, indicating a constant mass (m2). The samples were then overhauled by inserting them in a desiccator with fresh dry silica gel at 37 ± 2 °C for 1 day and then transferring them to another desiccator with freshly dried silica gel for 1 hour at room temperature. The desorption process was performed by repeated weighing until the change in weight between each weighing did not exceed 0.2 mg, achieving a constant mass (m3, reconditioned mass). Lastly, sorption and solubility in µg/mm3 were calculated using Eqs. (1 and 2), respectively [22].
Sorption= m2-m3/V | (1) |
Solubility= m1-m3/V | (2) |
2.4. Statistical Methods
Two-way ANOVA and independent t-tests were employed at a significance level of 0.05 after confirming the normality of the data in the groups using the Kolmogorov-Smirnov test.
Table 1.
Mean± SD | Thermal Stress | Resin Type |
---|---|---|
65.137±7.687 | Without thermocycling | 3D-printed |
67.468±7.089 | With thermocycling | |
88.002±10.723 | Without thermocycling | Heat-cured |
86.598±6.464 | With thermocycling |
3. RESULTS
3.1. Flexural Strength
Table 1 presents the means and standard deviations of flexural strengths in the two groups in MPa. The result shows no statistically significant difference between the mean flexural strength of the two resins in the presence or absence of thermal stress [P>0.05].
The results demonstrated that there was a statistically significant difference between the mean flexural strengths of the two resins, and the mean flexural strength of heat-cured resin was higher than the one fabricated with a 3D printer [P<0.001]. There was no statistically significant difference in the mean flexural strengths in the presence or absence of thermal stress [P>0.05]. Moreover, there was no reciprocal effect between the resin type and thermal stress. In other words, the effect of the presence or absence of thermal stress on the mean flexural strength was the same in both resin types [P>0.05].
The independent-sample t-test was applied to assess the influence of the presence or absence of thermal stress on the mean flexural strength in each resin at a significance level of 0.05. Independent-sample t-test showed that the mean flexural strength of heat-cured resin was higher than the one fabricated with a 3D printer resin [P=0.001].
3.2. The Surface Morphology
Based on images of the optical microscope and SEM, the layered structure was detected in the samples ready with all groups. However, after polishing, the layering disappeared in all cases. Figs. (1 and 2) present the results. It was observed that polishing had a better effect in disappearing a layered structure for a 3D printer resin. The layered structure had no differences in the presence or absence of thermal stress.
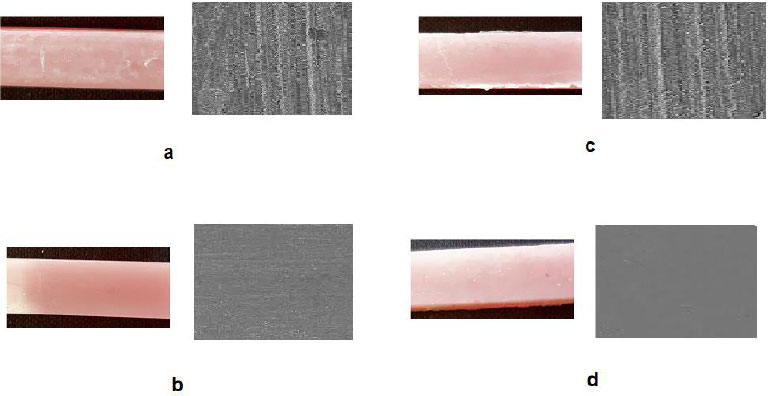
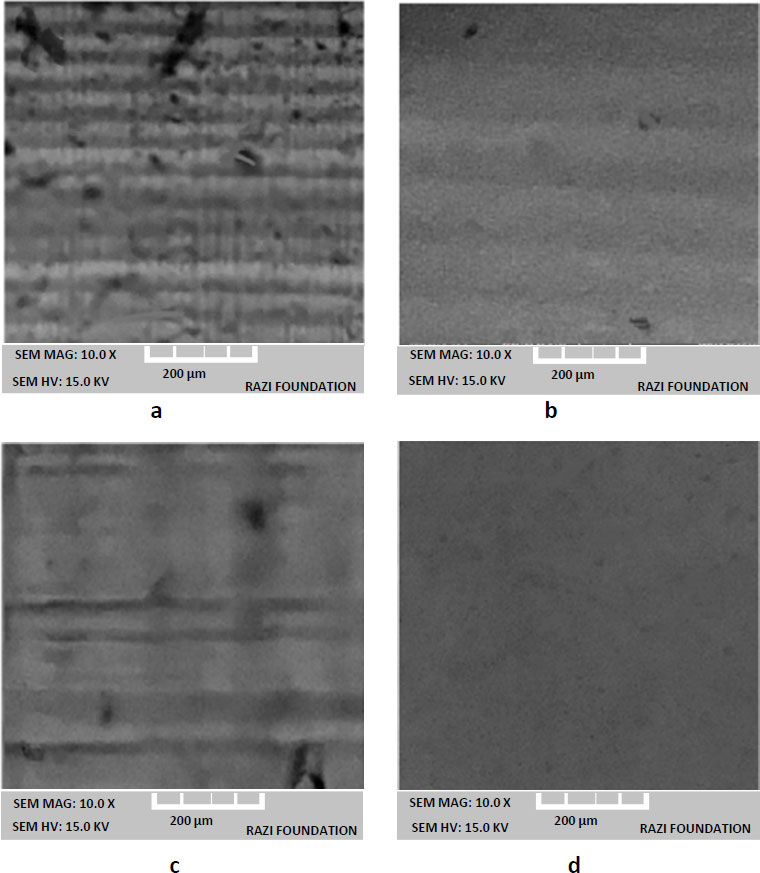
Groups | Mean Water Sorption in the Absence of Thermal Stress | Mean Solubility in the Absence of Thermal Stress | Mean Water Sorption in the Presence of Thermal Stress | Mean Solubility in the Presence of Thermal Stress |
---|---|---|---|---|
The 3D printer resin | 31.34 ± 1.34 µg/mm3 | 1.5 ± 0.08 µg/mm3 | 31.38 ± 0.79 µg/mm3 | 1.5 ± 0.12 µg/mm3 |
Heat-cured resin | 27.25 ± 0.48 μg/mm 3 | 1.5 ± 0.14 μg/mm 3 | 29.20 ± 0.57 μg/mm 3 | 1.6 ± 0.06 μg/mm 3 |
3.3. Water Sorption and Solubility
The mass change with time was obtained for all groups while being in artificial saliva. In the water sorption phase, the mass increased rapidly in the first 7 days, and then the increase rate decreased until it reached an equilibrium on day 21. In the desorption phase, the mass decreased steadily in the first 7 days, and then reduction was continued until equilibrium was reached on day 21 of the dewatering process.
The 3D printer samples had greater water sorption [P=0.001], and there were no differences between the solubility of 3D printer samples and heat-cured samples [P=0.12]. Heat-cured resin showed increased water sorption after thermal cycling [P=0.04], and 3D-printed materials exhibited the same behavior (but not significant, P=0.23]. Table 2 presents the results for the water sorption and solubility.
4. DISCUSSION
Despite their lower flexural strength than other materials, 3D-printed materials are an alternative option for fabricating dentures. Their advantages include easy fabrication and reduced treatment time in the clinic. Many studies are underway to improve the mechanical properties of denture base resins made with a 3D-printing machine, leading to similar results as conventional heat-cured acrylic resins.
A higher flexural strength of a resin is the main factor for the longevity of dentures and for preventing cracks under forces. Flexural strength is the cumulative measurement of compressive, tensile, and shear stresses of different materials.
Dentures undergo repeated masticatory forces during their lifespan. Since denture bases might break in real life for various reasons, their material must have high flexural strength.
The first hypothesis of the study was that the flexural strength of acrylic resins was not significantly different; however, it was rejected as this study reported that the mean flexural strengths of the two resins were significantly different. Moreover, the second hypothesis that thermocycling would not affect the flexural strength of any resins was accepted.
Similar research studies have indicated that 3D-printed resins exhibit lower flexural strength when compared to heat-cured acrylic resins [11, 23, 24]. Prpić et al. conducted a study to compare the flexural strength and surface hardness among three materials: a CAD/CAM-milled polymer, a 3D-printed polymer, and a polyamide denture base material. The 3D-printed resin demonstrated significantly lower flexural strength compared to the other materials, measuring between 60–85 MPa. However, it is worth noting that this strength still meets the clinical acceptability threshold of 65 MPa as per ISO standards. Furthermore, the 3D-printed resin exhibited lower surface hardness than the alternative materials. In conclusion, it was found that conventionally milled or heat-cured dentures currently outperform 3D-printed dentures [15].
Al-Qarni and Gad compared the flexural properties of three types of 3D-printed resins with a conventional heat-cured resin and concluded that samples fabricated with 3D printing using three types of resin had lower flexural strengths than heat-cured resin samples [24, 25].
Chhabra et al. reported that NextDent 3D+ denture samples had significantly lower flexural and impact strengths than DPI heat-cured samples [26].
In contrast, some studies reported that the flexural strength values of milled polymethylmethacrylate differed from CAD/CAM polymethylmethacrylate polymers.
Steinmassl et al. compared the fracture strength of heat-cured denture base resins with milled resins and reported similar, lower, or higher fracture strength values than the heat-cured control group [27]. Pacquet et al. reported higher flexural strength values for heat-polymerized PMMA than milled denture base materials [28].
Prosthetic material with high elastic modulus can be fabricated thinner without compromising the mechanical properties and would be comfortable for the patient. In addition, a denture resistant to deformation has a more stable occlusion. Manufacturing procedures might account for the difference in rigidity between conventional resins and CAD/CAM ones.
MOE can be related to the amount of residual monomer. It has been reported that a high amount of monomer can decrease the glass transition temperature and increase the elasticity of the resin. Polymerization with high temperature and pressure in CAD/CAM resins leads to higher amounts of monomer conversion and decreases the amount of residual monomer, consequently increasing resin rigidity. In addition, polymerization with high temperature and pressure allows different arrangements of polymer chains [29].
3D-printed acrylic resins used in removable denture bases have a lower double bond conversion, which can affect their mechanical properties. In contrast, in CAD/CAM resins, high pressure certainly helps develop longer polymer chains and can lead to higher monomer conversion. In addition, mineral fillers, as well as the high temperature used in the fabrication of the resins, improve the mechanical properties of PMMA, including its flexural strength [30].
Although 3D-printed materials (acrylic ester-based monomers) had the lowest flexural strength values compared to the heat-cured acrylic resins, they met the ISO requirements for flexural strength (65 MPa) [31]. 3D-printed materials for denture bases are definitely a novel choice for dentures despite having lower flexural values than other materials used in denture bases. The thermocycling method has been introduced as a technique of artificial aging, which mostly involves water immersion and temperature alteration under standardized in vitro situations [32]. In this study, thermocycling reduced the flexural strength of PMMA and increased the flexural strength of 3D-printed resin (although these changes were not significant). The current report is consistent with the results of other studies, which showed that thermocycling reduced some mechanical properties of PMMA resin [33, 34].
The increase in strength of 3D-printed resins due to thermocycling is related to multifactorial monomers, which can create cross-links with other monomers. In addition, 3D-printed resins have mineral fillers that prevent fine cracks [35].
It seems that polishing had a better effect in disappearing of layering structure for a 3D printer resin. Al-Dulaijan et al. reported that the common polishing procedures formed the smoothest surfaces in 3D-printed materials [36]. The surface roughness of heat-cured resins can be affected by some aspects, comprising the ratio for powder/liquid, appropriate treatment and mixing, and the material texture, while for a 3D-printed resin, the printing technology affects the surface properties of the resin [37]. This approves the possibility of the higher roughness amounts of unpolished 3D-printed products that can be attributed to the mechanism of fabrication [38].
In a study by Perea-Lowery, the samples for 3D printers also showed greater water sorption [23]. In another study, Berli et al. [39] also reported higher water sorption values for 3D-printed resins. The low water sorption for heat-cured resin is due to the complete polymerization compared to the 3D-printed resins. Indeed, in a 3D-printed method, the polymerization degrees are low and result in unreacted monomers [23]. Besides, the components, such as plasticizers, crosslinking agents, initiators, or other soluble materials, can lead to high water sorption in 3D-printed resins [22].
The results reported no differences between the solubility of 3D printer samples and heat-cured samples. Another study demonstrated that the solubility of 3D-printed acrylic resins increased in comparison to pressed resin [39]. The increased solubility may be due to the leaching out of the residual monomers [40].
For heat-cured resins, the water sorption increased after thermal cycling, while for 3D-printed resins, it had the same behavior (but not significant). The thermal cycling technique is used to mimic the clinical situation to be a standard for material actions in the oral area. Furthermore, increased temperature has been exposed to quicken the water uptake [40]. In a study by Lassila and Vallittu, it was reported that materials with high solubility will absorb more water [41]. Moreover, based on the reports, an increase in the solubility of denture base resin may result in a reduction in mechanical possessions, so the solubility must be low [42].
4.1. Clinical Significance
The 3D printed resins with improved properties can be appropriate for substituting conventional denture base manufacturing methods in prosthetic dentistry.
4.2. Limitations
First, this study was an in vitro study. Both resins should be verified in the clinical situation. In clinical studies, they may show different properties. Only common heat-cured and 3D-printed denture base resins were involved in this study. Resins fabricated by other techniques should also have been used for comparison. Besides, future studies should include many brands of resins in analyzing and comparing different properties.
4.3. Future Perspectives
In the future, the existing restrictions of the 3D printing method must be resolved for these materials in future. One of the most important advantages of using digital technologies is that these technologies greatly decrease the amount of treatment sessions required. Moreover, this process contributes to short-term clinical routines, rational financial costs, and optimistic patient-related outcomes. Clinical training is vital to logically confirm the demanded rewards of this new technology. High flexural is one of the desirable features for the denture base, so it is necessary to improve the 3D printed denture base resins in terms of this feature in order to take advantage of the 3D printing technique compared to conventional methods. Various features associated with resin composition and chemistry, the kind of printer and the operating standards of the printer, and the post-curing procedure should be improved in the future.
CONCLUSION
The methods based on 3D printing have entered the field of dentistry and play a vital role in esthetic dentistry. The most important advantage of this method compared to the common heat-cured polymerization procedure is the reduction of production time. Before and after thermocycling, the flexural strength of conventional heat-cured polymethylmethacrylate resin was higher than 3D-printed acrylic resins. Moreover, two types of resins met ISO requirements concerning tensile strength before and after thermocycling [≥65 MPa] despite having a statistically significant difference in flexural strength. It was found that thermocycling affected the flexural strength of none of the acrylic resins. Furthermore, polishing had a better effect in disappearing the layered structure for a 3D printer, which can be attributed to the mechanism of fabrication. The 3D printer samples had greater water sorption, and there were no differences between the solubility of 3D printer samples and heat-cured samples.
AUTHORS’ CONTRIBUTIONS
T.G. and E.M. conceived the idea. T.G., E.M., and K.A. proposed the overarching research goals. T.G., E.M., K.A., and S.M.D. wrote the initial draft. S.M.D. revised the version. T.G., E.M., and S.M.D performed the statistical analysis. All the authors were involved in study conception, data collection, data acquisition and analysis, data interpretation, manuscript writing, and manuscript revising. All authors have read and agreed to the published version of the manuscript.
LIST OF ABBREVIATIONS
PMMA | = Polymethylmethacrylate |
AM | = Additive Manufacturing |
MPa | = Megapascals |
SEM | = Scanning Electron Microscope |
ETHICAL STATEMENT
The ethics committee at Tabriz University of Medical Sciences provided the ethical code, and all procedures were carried out after getting the ethical code [IR.TBZMED.VCR.REC.1401.074].
AVAILABILITY OF DATA AND MATERIAL
The raw/processed data needed to reproduce these outcomes can be shared. Also, after publication, the data can be requested from the corresponding author [I.G] via email.