Preparation and Evaluation of Chitosan Nanoparticles containing Iranian Eschium Amoenum Extract and its Antimicrobial Effects on Common Oral Microorganisms
Abstract
Background and Aim:
Chitosan nanoparticles are one of the biocompatible and bioactive vectors in medicine contributing to the transfer and slow release of antimicrobial agents. The present study investigated loading Echium amoenum extract on chitosan nanoparticles and evaluated the antimicrobial effects of this composite on Streptococcus mutans, Candida albicans, and Escherichia coli.
Materials and Methods:
First, a chitosan solution was prepared in 1% acetic acid. Then, a tripolyphosphate (TPP) solution was added to it. The resulting mixture was centrifuged, and finally, its powder was dried. The E. amoenum extract was prepared and added to the chitosan powder. After mixing, the mixture was centrifuged, and the chitosan nanoparticle powder containing E. amoenum was separated and dried. The properties and characteristics of the nanoparticles were determined by the DLS test, and their stability was evaluated using the zeta potential. Finally, the antimicrobial effect of this product was evaluated on S. mutans, E. coli, and C. albicans using MIC and MBC through the microdilution method.
Results:
The sizes of chitosan nanoparticles and chitosan nanoparticles containing the plant extract were 98±1.24 and 108±1.54 nm and their zeta potentials were +17 and +10, respectively. The MIC for chitosan, the extract, and chitosan nanoparticles containing E. amoenum extract for E. coli were 170.67, 666.67, and 341.34 mg/mL; these values for S. mutans were 106.67, 416.67, and 170.67 mg/mL, with 426.67, 1000, and 341.34 mg/mL for C. albicans, respectively. The MBC for chitosan, the extract, and chitosan nanoparticles containing E. amoenum extract for E. coli were 426.67, 1666.67, and 853.34 mg/mL; these values for S. mutans were 426.67, 833.34, and 426.67 mg/mL, with 853.34, 1666.67, and 853.34 mg/mL for C. albicans, respectively.
Conclusion:
Chitosan nanoparticles are an efficient vector for E. amoenum extract and loaded chitosan nanoparticles can be used as a bioactive antibacterial agent against various oral microorganisms.
1. INTRODUCTION
The oral environment can be considered a haven for many pathogenic and opportunistic microorganisms due to its continuous relationship with the external environment. Recent investigations have identified >1000 microbial species in the oral cavity [1], colonization of which, increases the chances of their penetration into the other body tissues, resulting in the development of systemic diseases [2]. Streptococcus mutans is a facultative anaerobic gram-positive bacterial species and a member of the oral microbiome. Due to its acidogenic and aciduric properties, it is the most pathogenic bacteria for caries and root canal infections. This bacterial species can form biofilms, known as dental plaque [3].
Candida albicans is a fungal species commonly found in oral infections comprising up to 75% of fungal species in the oral cavity [4]. The virulence factors of C. albicans include the ability to change its morphology, strong adhesion to porous surfaces and cells, thigmotropism, and the ability to secrete hydrolytic enzymes, such as protease, phospholipases, and lipases [1, 5].
Escherichia coli is a facultative anaerobic gram-negative bacterial species primarily identified in the digestive tract. E. coli has also been identified in various oral and root canal infections [6].
The emergence of antibiotic-resistant bacteria has precipitated a growing health crisis which is compelling researchers towards alternative methods with both antibacterial activity and minimum side effects for human beings [7]. Many studies have evaluated the antibacterial properties of medicinal plants [8, 9]. An important medicinal plant in Iranian traditional medicine is the species of Echium, especially Echium amoenum (from the Boraginaceae family), due to its medicinal and nutritional properties [9]. This plant’s extract contains flavonoids, saponins, unsaturated terpenoids, and sterols. Phytochemical studies on E. amoenum have shown the presence of many chemical agents, such as RA, anthocyanidin, flavonoids, g-linolenic acid, and small amounts of alkaloids [8, 10]. The antioxidative and antimicrobial properties of flavonoids and RA are well-established [11].
Previous studies have evaluated the antimicrobial effects of E. amoenum against Staphylococcus aureus using agar diffusion and microdilution methods. The minimum concentration of E. amoenum that inhibits the growth of S. aureus (MIC) has been reported to be 6.2 µg/mL [12]. Another study evaluated the antimicrobial effect of E. amoenum seed oil against S. aureus, C. albicans, and Pseudomonas aeruginosa. The results showed that the seed oil of E. amoenum did not affect S. aureus; however, it significantly decreased C. albicans and P. aeruginosa [13].
Chitosan-based drug delivery systems are used to carry and deliver anti-inflammatory, antibacterial (different antibiotics), antioxidative and anti-cancer agents [14]. Nanoparticles (NPs] prepared from chitosan derivatives usually have a positive surface charge and mucous-adhesion properties, so they can adhere to mucous membranes and release their drug loads consistently [15]. Several studies have evaluated chitosan NPs to carry different antibiotics, polyphenol products, [16, 17] and plant extracts such as Mentha longifolia [18], Arrabidaea chica [19], Scutellariae baicalensis [20] and Ginkgo Biloba [21]. Producing chitosan NPs using TPP as a crosslinker is an efficient and safe method. Particles are made by electrostatic interactions between amine groups of chitosan and phosphate groups of TPP. It has been reported that addition of TPP results in lower particle size, improved loading efficiency, cellular uptake and better bioactivity [22].
Given the appearance of bacterial species resistant to antibiotics and the side effects of these compounds, attempts to achieve alternative materials using herbal compounds or natural polymers have increased. In addition, the next step to take is to load the target agent in bioactive nanocarriers to achieve a persistent slow release Although previous studies demonstrated the antibacterial activity of E. amoenum , the present study evaluated the effect of loading E. amoenum on chitosan NPs and its slow release to control oral microorganisms, so an alternative could be introduced for currently available mouthwashes and antimicrobial agents.
2. MATERIALS AND METHODS
In the present in vitro study, standard strains of S. mutans (ATCC 25175) and E. coli (ATCC 25922) from the microbial bank of Iran Pasteur Institute were prepared and cultured on a blood agar solid medium. In addition, C. albicans fungal species (ATCC 10231) was incubated in Sabouraud dextrose agar medium for 24 hours. MIC (minimum inhibitory concentration) test was performed using the microdilution method to evaluate the antimicrobial properties and minimum inhibitory concentration of the above-mentioned nanoparticles. To this end, the microorganisms were cultured in the tryptic soy broth (TBS) medium at 37ºC for 24 hours. The next day, McFarland’s 0.5 concentration, equal to 1.5×108 CFU/mL was prepared. Different concentrations of the chitosan NPs containing the extract were prepared to evaluate their effects. Different concentrations of the material were added to special microtiter plates. In addition, two wells were allocated to chitosan and E. amoenum extract alone. One well that contained the culture medium and the evaluated material (without bacteria) was considered the negative control. Then the microbial suspension was added to the wells and incubated in the presence of CO2 at 37ºC for 24 hours. MIC is the minimum concentration of the material that presents the formation of colonies on the culture medium surface.
To determine MBC (minimum bacterial concentration), 10 µL of the solution was removed from the wells with no microorganism growth and cultured in the agar medium. Finally, the plates were incubated, and the formed colonies were counted. MBC is the minimum concentration of the material that prevents colony formation on the culture medium surface [23, 24].
2.1. Preparation of Chitosan Nanoparticles
The ionic gelation method was used to prepare chitosan NPs, in which chitosan solution was prepared at a concentration of 1 mg/mL in 1 wt% of acetic acid. This solution was placed on a stirrer until a completely clear solution was achieved with no undissolved particles. Tripolyphosphate solution was prepared with 1 mg/mL concentration in distilled water and added to the chitosan solution. After completing the reaction, to separate the NPs, the suspension was centrifuged at 20000 rpm for 10 minutes. To eliminate impurities, the sample was rinsed with distilled water and centrifuged again. The achieved nanoparticle powder was dried and used [25].
2.2. Loading E. Amoenum Extract in Chitosan
E. amoenum extract was procured, and its aqueous solution [1 mg/mL] was prepared. Then this solution was mixed with 20 mg/mL of NPs. The mixing procedure continued on a stirrer for 24 hours. Then the NPs containing the extract were centrifuged at 10000 rpm. Then the NPs were rinsed with distilled water and centrifuged again. The resulting powder was dried and used.
2.3. Evaluation of the Properties and Characteristics of Chitosan NPs Containing E. Amoenum Extract
The particle size distribution graph was drawn using the laser diffraction method to compare the sizes of chitosan NPs prepared from the initial chitosan sample. The NP sizes were determined using the DLS [dynamic light scattering] method. In addition, the zeta potential was evaluated to determine the stability of NPs.
3. RESULTS
3.1. Characterization of Nanoparticles
According to the DLS test, the NP sizes of chitosan and chitosan containing E .amoenum extract were 98±1.24 and 108±1.54 nm, respectively (Figs. 1 and 2). The polydispersity index for chitosan NPs and chitosan NPs containing E. amoenum extract were 0.3 and 0.4, respectively. The zeta potential of chitosan NPs and chitosan NPs containing E. amoenum extract were +17 and +10 mV, respectively, according to the same test (Figs. 3 and 4).
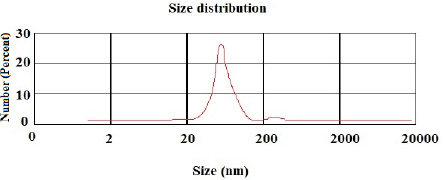
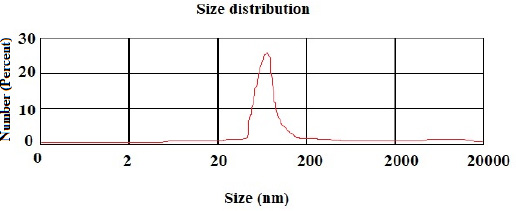
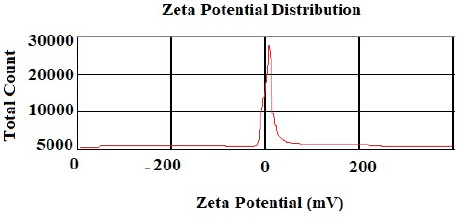
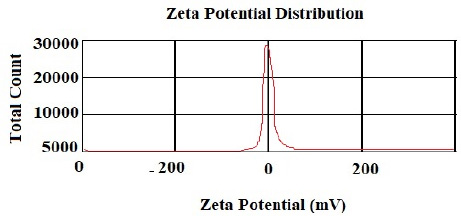
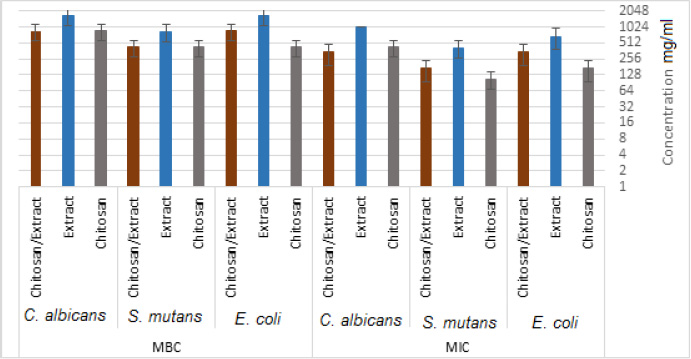
3.2. Antibacterial Activity
The MICs for chitosan, E. amoenum extract, and chitosan NPs containing E. amoenum extract against E. coli were 170.67, 666.67, and 341.34 mg/mL, respectively. These values for S. mutans were 106.67, 416.67, and 170.67 mg/mL, with 426.67, 1000, and 341.34 mg/mL for C. albicans, respectively (Fig. 5). The MBCs for chitosan, the extract, and chitosan nanoparticles containing E. amoenum extract for E. coli were 426.67, 1666.67, and 843.34 mg/mL, respectively. These values for S. mutans were 426.67, 833.34, and 426.67 mg/mL, with 853.34, 1666.67, and 853.34 mg/mL for C. albicans, respectively (Fig. 5).
4. DISCUSSION
The present study evaluated the antimicrobial properties of chitosan, E. amoenum extract, and chitosan nanoparticles containing E. amoenum extract against S. mutans, C. albicans, and E. coli as the most commonly found bacterial and fungal species in the oral cavity [1]. The results show that the MIC and MBC values of chitosan NPs containing E. amoenum extract are less than the MIC and MBC values of E. amoenum extract alone against all tested pathogens. The decrease in MIC and MBC against microorganisms of the E. amoenum extract after loading on chitosan NPs is due to the synergistic antimicrobial effect of chitosan. In addition, NPs have a larger surface area for contact with cells and bacteria due to their smaller size and larger total surface area, increasing the bioactivity of medications loaded on NPs [26]. A study by Farmoudeh et al. showed that loading ginger extract on chitosan NPs decreased MBC and MIC against different microorganisms [27]. Consistent with the present study, Bagheri et al. showed that loading nettle [urtica] extract on chitosan NPs produced using the ionic gelation method improved the antibacterial properties of this compound against E. coli and S. aureus [28].
Previous studies have attributed the antimicrobial properties of E. amoenum extract to its phenolic compounds. The antimicrobial effect of this extract is dose-dependent, and its effect increases with an increase in concentration and its release from the vector [29]. In the present study, the minimum concentration required for inhibiting E. coli was higher than that for S. mutans, which might be explained by differences in the bacterial wall between gram-negative [E. coli] and gram-positive [S. mutans] bacteria, making the antibacterial agents less effective on gram-negative bacteria [30].
Previous studies have evaluated the antibacterial effect of E. amoenum extract on S. aureus using agar diffusion and microdilution methods. A previous study reported an MIC of 6.2 mg/mL to inhibit S. aureus [12]. Bonjar et al. evaluated the antimicrobial effect of E. amoenum extract on Bordetella bronchiseptica and Klebsiella pneumoniae using the disc diffusion method and reported an MIC of 15 µg/mL for the methanolic extract of E. amoenum [31]. Another study showed that the seed oil of E. amoenum had no effect on S. aureus but significantly decreased C. albicans and P. aeruginosa counts [13]. All these studies have shown the antibacterial and antifungal activity of E. amoenum extract, consistent with the present study.
In the present study, the E. amoenum extract was loaded on chitosan NPs, and its properties were evaluated using the ionic gelation method, which can be implemented in an aqueous environment and does not lead to new toxic chemical bonds in the chitosan structure [32].
In the present study, the chitosan NP dimension was 98 nm. Previous studies have shown that 10‒100-nm NPs are suitable for carrying and delivering medicines because NPs with larger sizes might not penetrate the target cells and might accumulate in vital organs such as the liver and kidneys [26]. In addition, in the present study, the particle distribution index [PDI] was 0.3, indicating uniform NP sizes.
A previous study by Fan et al. (2012) showed that preparing chitosan NPs using the ionic gelatin method leads to the production of NPs with high stability with a diameter of about 138 nm, with the help of TPP as a cross-linking material [33]. In the present study, the chitosan NP size was 98 nm. In addition, the present study showed that with this method, the stability of chitosan NPs was favorable at room temperature for up to 20 days; i.e., the dimensions and distribution of NPs did not change significantly during this period.
Vaezifar et al. (2013), too, used the ionic gelation method and TPP anion to prepare chitosan NPs. They reported that the dimensions of the NPs produced using this method depended on the initial concentration of chitosan, the concentration of TPP, and the duration of the reaction. The optimal factors reported in this study were 1 mg/mL, 1 mg/mL, and 60 minutes, respectively [34]. Since the parameters of the ionic gelation method in the present study were consistent with those in the study by Vaezifar et al., the NP dimensions in the present study were consistent with that study, i.e., NP dimensions were <200 nm in both studies.
Neves et al., too, (2014) used this method to produce chitosan NPs and showed that the NPs’ zeta potential increased with an increase in chitosan concentration, which might be attributed to an increase in NH3+ groups [35]. The zeta potential shows the electrostatic resistance between the particles and is mostly used as an index to evaluate the distribution stability of the sample. A high zeta potential indicates that the suspension is in a favorable state regarding electrostatic stability. In addition, this parameter affects the amount and rate of medication release from the NPs and the interaction between the medication and the cell. The zeta potential of chitosan NPs in this study was +17 mV, indicating the stability of the compound. However, loading the extract decreased this stability to +10 mV [36]. The type of material loaded on chitosan NPs affects its stability and zeta potential. For example, Du et al. showed that loading metallic ions [with a positive charge] increased the zeta potential of chitosan NPs [37]. A decrease in zeta potential after loading E. amoenum extract was due to phenolic components in this extract. However, a positive zeta potential facilitates the interaction between the NPs and the cell membranes for the absorption and release of medications and with bacterial walls to destroy bacteria [38]. Loading the E. amoenum extract slightly increased NP sizes [108 nm], consistent with previous studies. Bagheri et al., too, (2021) produced chitosan NPs using the ionic gelatin method. The dimension of these NPs was 208 nm before loading, which increased to 369 nm after loading nettle oil [28]. In this line, Keawchaoon et al. (2011) loaded the phenolic compound carvacrol on chitosan NPs. An increase in carvacrol concentration increased the dimension of chitosan NPs and decreased the zeta potential [39]. In addition, loading clove extract on chitosan NPs in a study by Hadidi et al. (2020) increased the NP dimension from 223 to 444 nm and decreased the zeta potential [40].
In summary, chitosan NPs in the present study produced using the ionic gelatin method, presented acceptable characteristics and addition of E. amoenum extract to chitosan NPs demonstrated a synergistic antibacterial and antifungal effect against common oral microorganisms. However, wider studies on cytotoxicity and biofilm cultures are needed previous to bringing the produced compound to the clinical evaluations. One of the future aims of the present study is to use chitosan NPs containing E. amoenum extract to prepare mouthwashes against dental caries or fungal diseases or prepare intracanal medications for radicular infections or placement in periodontal pockets to treat periodontitis.
CONCLUSION
Chitosan NPs containing E. amoenum extract produced by ionic gelation method demonstrated a synergistic effect resulting in elimination of the common oral bacteria and fungi. Considering the structural properties of chitosan NPs as a natural polymer, they can be used as a proper and safe vector for medications and extracts with antibacterial and antifungal properties for oral microorganisms.
LIST OF ABBREVIATIONS
TPP | = Tripolyphosphate |
TBS | = Tryptic Soy Broth |
ETHICS APPROVAL AND CONSENT TO PARTICIPATE
The project was found to be in accordance with the ethical principles and the national norms and standards for conducting Medical Research with an Ethics code of IR.TBZMED. VCR.REC.1401.125 from the ethics committee of Tabriz University of Medical Sciences.
AVAILABILITY OF DATA AND MATERIALS
The raw/processed data required to reproduce these findings can be shared after publication by requesting from the corresponding author [S.D].
FUNDING
This study has been funded by the Vice-Chancellor for Research (VCR) of Tabriz University of Medical Sciences.
CONFLICT OF INTEREST
Dr. Solmaz Maleki Dizaj is on the Editorial Advisory Board of the journal The Open Dentistry Journal.
ACKNOWLEDGMENTS
This study was based on a thesis [No. 69924] registered at the Faculty of Dentistry, Tabriz University of Medical Sciences, Tabriz, Iran. It was financially supported by the Vice-Chancellor for Research at Tabriz University of Medical Sciences, Tabriz, Iran which is greatly acknowledged.