All published articles of this journal are available on ScienceDirect.
The Biomineralization Conundrum of Calcified Extraradicular Deposits on the Apical Root Surface of Teeth: A Case Report
Abstract
Background:
Calcified extraradicular deposits on the apical root surface of teeth (CEDARST) are rarely seen in the dental field, compared with dental calculus deposits, which are very frequent. However, the exact biomineralization process of this entity has remained unclear until now.
Objective:
To report a case of an exuberant calcified extraradicular deposit on the apical root surface of an anterior tooth with apical periodontitis and propose for the first time some potential mechanisms involved in this pathological mineralization, and thereby suggest directions for future research.
Case Presentation:
In brief, a root-canal-treated canine displaying a radiographic periapical radiolucency was treated, and the sinus tract persisted despite root canal retreatment in which high endodontic standards were maintained. Endodontic surgery was performed, and the curettage of the pathologic tissue revealed an exuberant calculus-like material attached to the outer root surface, around the exit of the apical foramen. Two-year follow-up radiography revealed complete bone healing, and the patient´s tooth was asymptomatic and functional.
Conclusion:
The development of CEDARST seems to depend on a fine synergic control exerted by specific microorganisms (mainly bacteria) on this pathological mineralization, as well as on the local chemical microenvironment of the apical lesion of the affected tooth. Microbial implications and inhibitors of mineralization seem to play a dominant role in CEDARST biomineralization.
1. INTRODUCTION
Root canal treatment (RCT) is a very common form of dental therapy; globally, the prevalence of people who have had at least one root canal treatment is 55.7% [1]. The ultimate goal of RCT is to prevent the development of apical periodontitis (AP) or, when the disease has already occurred, to create the right conditions for the healing of the periradicular tissues [2]. AP is a chronic inflammatory disease of the periradicular tissues caused by a microbial infection within the root canal system [3, 4]. It is one of the pathologies for which a tooth requires RCT, being almost always asymptomatic [2], and its prevalence is high, as evidenced by published studies [5-7]. AP pathobiology also includes neuro–immune–vascular interactions that eventually lead to the formation of a periapical lesion in the periradicular bone, as well as pathologic changes in the periodontal ligament and cementum [2].
Bacteria (sessile or planktonic) are the main etiological pathogens related to AP [2, 8]. However, the microbial nature of the latter is diverse; bacteria, fungi, archaea, and viruses have all been associated with this pathology [8-11]. In a seminal study of teeth with AP, the authors of [12] found that intraradicular biofilms are much more frequent than extraradicular biofilms, with prevalence rates of 77% and 6%, respectively. Overall, bacteria and AP establish acause–effect relationship [2], in which bacterial persistence after RCT can result in the eventual failure of the therapy, and the persistence or recurrence of AP. Extraradicular biofilms can cause post-treatment periodontitis and consequent RCT failure [13, 14] and their calcification has been reported in a few case reports in the endodontic field, being described as calcified-extraradicular deposits [15-21].
Biomineralization is a process inherent to all organisms on Earth. It is perhaps the most ancient chemical process linked to the origin, evolution, and conservation of life on our planet [22, 23] and represents a dynamic means by which various solid phases are deposited in biological molecules, cells, and tissues. This includes both normal and pathological biomineralization in humans [24]. Microbial-associated biomineralization is an important aspect of some infections in humans and in other animals [22, 25], including dental calculus [26, 27], urinary calculi [28-30), and salivary calculi [31-33]. Placental tissues can also be affected [34]. CEDARST are calculus-like deposits that have been rarely reported in the endodontic literature [15-21]; until now, no study has attempted to identify the potential mechanisms involved in the process of this pathological mineralization. This study presents a case report of a maxillary canine with CEDARST. An apical lesion with a sinus tract was present, the latter serving as the only communication with the oral cavity. Diagnosis and treatment planning were based on clinical and radiographic findings. The key aim of this review is to propose for the first time some potential mechanisms involved in this pathological mineralization, because the exact process of biomineralization of this entity has remained unclear until now.
2. CASE REPORT
A 55-year-old man attended the dental office of the author, complaining of swelling and bite tenderness in the left anterior maxilla, beginning two days previously. He had no history of trauma and declared that an RCT had been carried out on tooth 23 in a general dental office, months previously, and that he had been medicated many times with antibiotics. He also reported several episodes of a sinus tract before, during, and after RCT. His medical history was noncontributory. Intraoral examination revealed fairly good oral hygiene. A moderate swelling fluctuant to palpation was noted in the periapical area of tooth 23. Percussion and palpation gave positive responses. Periodontal probing did not reveal pockets exceeding 3 mm deep around the tooth’s root. No sinus tract could be seen buccally or lingually. Tooth 23 had a partial and poorly adapted restoration, was exposed to the oral environment, and a wire fragment could be seen inside the pulp chamber.
Conventional periapical radiography (CPR) was taken and showed a round radiolucent area surrounding the tooth apex. The outline of the root apex appeared radiopaque and irregular, suggesting a calculus-like deposit. Root-filling material of a “loose” appearance could be seen inside the main canal, which was not reaching the tooth apex, clearly indicating an improper RCT. In addition, a poorly adapted restoration was seen on the mesial side of the crown as well as a small and curved fragment of wire, just on the orifice of the main canal (Fig. 1A). Considering the clinical and CPR findings, a diagnosis of acute apical abscess was made. Root canal retreatment was planned in two sessions and the patient was warned that if the symptoms/and or the apical lesion did not resolve after the retreatment, he certainly would require endodontic surgery. Prior to the clinical procedures, informed consent was obtained from the patient.
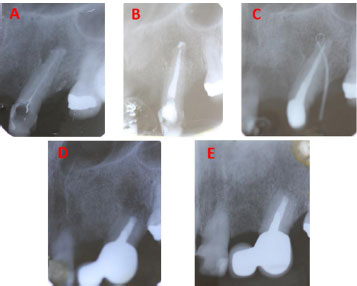
Following topical anesthesia with spray lidocaine (Roxicaine, Ropsohn Therapeutics, Colombia), local anesthesia was applied using the anterior middle superior alveolar nerve block, using 2% lidocaine with 1: 80,000 epinephrine (Xylestesin-A, 3M ESPE, Seefeld, Germany). Incision and drainage were established, through the most dependent site of the swelling, using a scalpel (Hu-Friedy Manufacturing Co, Chicago, IL, USA) equipped with a #15 blade; considerable pus drainage was obtained. Immediately, rubber dam isolation was placed, and the pulp chamber was accessed and gently flushed with 5,25% sodium hypochlorite solution (NaOCl), delivered with a 3 ml syringe using a 27-gauge Monoject (Kendall, COVIDien, Mansfield, MA, USA) needle. All the caries and the former partial composite coronal restoration material were removed; the access cavity was then refined with an Ultrasonic Retreatment Tip ET18D (Acteon Satelec, Merignac, France). The small fragment of wire was easily removed with an Ultrasonic Retreatment Tip ET25 (Acteon Satelec, Merignac, France), and a loose gutta-percha point under the former, using a #30 Hedström file (Dentsply Maillefer, Ballaigues, Switzerland). A size 20/0.06 taper stainless steel LA Axxess (SybronEndo, Orange, CA, USA) bur was used for preflaring the cervical and middle third of the canal. The canal was constantly irrigated with 5,25% sodium hypochlorite. The working length was determined with an electronic apex locator (Root ZX, J. Morita, Tokyo, Japan) and with a CPR. Biomechanical preparation was accomplished with Flexofiles and K-files (Dentsply Maillefer, Ballaigues, Switzerland), and a .02/70 K-file was finally obtained as the master apical file (MAF). Throughout all procedures, apical patency was maintained with a #10 K-file. At the completion of canal preparation, once the root canal was dried with sterile paper points, an intracanal exudate was still noticed, so the canal was filled with calcium hydroxide mixed with distilled water. A sterile cotton pellet was placed in the canal orifice, and the access was sealed with Cavit (3M ESPE, Seefeld, Germany). No antibiotics were prescribed to the patient, given that drainage was obtained and that no fever or malaise were exhibited.
At the next visit, one week later, infiltrative maxillary anesthesia was administered, rubber dam isolation was placed, and the canal was re-accessed using NaOCl, Monoject needle, and the MAF. Apical patency was maintained with a #10 K-file. A final ultrasonically activated irrigation protocol using 5,25% NaOCl and 17% EDTA solution was applied, assisted by a 25 mm long/size 25 Irrisafe file (Acteon Satelec, Merignac, France), driven with a P5 Newtron ultrasound device (Acteon Satelec, Merignac, France). Finally, the root canal was dried with sterile paper points and obturated with gutta-percha points and AH Plus sealer, using the cold lateral compaction technique. The access preparation was sealed with a light-cured glass ionomer and a post obturation CPR was taken (Fig. 1B).
The patient was referred to a prosthodontist dental office for placement of the final restoration. Ten days later, a cast-metal post was cemented in tooth 23, because a cantilever had already been planned to replace the previously lost tooth 22, taking into consideration patient needs and that cantilever showed excellent long-term clinical outcomes (especially in incisor teeth) compared with other fixed dental prostheses (35). Four weeks later, the patient attended the prosthodontist dental office to have the cantilever cemented. He declared that he had experienced no symptoms over the whole postoperative period. However, the dentist noticed a sinus tract buccally. Because of this, the cantilever was not cemented and the patient was referred back to the author´s dental office. There, the sinus tract was traced with a gutta-percha point and visualized with a CPR (Fig. 1C). The radiopaque point indicated tooth 23 as the origin of the sinus tract. Although the apical lesion was smaller in size, the patient was reminded of the necessity of future endodontic surgery, in light of these and previous dental findings. The patient decided to have the cantilever cemented before the apical surgery, because of aesthetic concerns. He was completely satisfied with the final esthetic results.
Two months later, the endodontic surgery procedure was performed. In brief, after infiltrative anesthesia, a full-thickness triangular flap was raised. After soft tissue enucleation of the apical lesion, apicoectomy was achieved using a high-speed handpiece along with a Zekrya bur (Dentsply Maillefer, Ballaigues, Switzerland). The resected tooth apex (Fig. 2) showed an exuberant black calculus-like deposit on its surface (CEDARST). For retrograde preparation, an AS3D Ultrasonic Tip (Acteon Satelec, Merignac, France) was used; afterward, the preparation was filled using mineral trioxide aggregate (ProRoot MTA, Dentsply Maillefer, Ballaigues, Switzerland). No complications were presented during the surgical procedure and post-surgery CPR was taken after suturing (Fig. 1D). Analgesics were prescribed and, after receiving postoperative care instructions, the patient was discharged. No antibiotics were prescribed. It is salient to point out that, considering the heavy biofilm aspect and calcified nature of the CEDARST, any antibiotic prescription would have been completely unhelpful, even in the previous endodontic treatment with the general dentist.
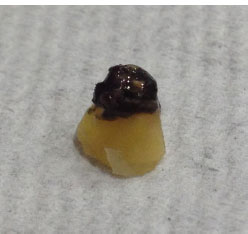
One week after surgery, the sinus tract associated with tooth 23 subsided, and the patient was subsequently symptom-free. Two years later, the patient presented for follow-up and declared that he had experienced no symptoms over the intervening period. A CPR was taken which showed complete resolution of the periapical lesion, i.e., the periodontal ligament space was completely continuous around the tooth’s root (Fig. 1E). Clinically, gingival tissues were healthy and no sinus tract was present; the cantilever was well-fitted. Tooth 23 was asymptomatic and functional.
A limitation of the present case report is that a histopathological analysis was not conducted; it cannot therefore be ascertained if the AP lesion was a granuloma or a cyst. However, after the endodontic surgery, the apical lesion was completely resolved, clearly indicating the above-mentioned bacteria and an APcause–effect relationship.
3. RESULTS AND DISCUSSION
This case report describes the surgical endodontic management of an exuberant calcified extraradicular deposit on the apical root surface of an anterior tooth with apical periodontitis. As mentioned above, such calculus-like deposits have been rarely reported in the endodontic literature [15-21]. However, up to now, no study has attempted to identify the potential mechanisms involved in the process of this pathological mineralization. In this regard, a key aim of this paper is to highlight for the first time some of these potential mechanisms, which are presented hereunder.
3.1. Microbial Implication in CEDARST Biomineralization
Microbe-mediated mineralization is a phenomenon ubiquitous in nature. All six taxonomic kingdoms contain members with the capability of forming minerals, including bacteria, fungi, viruses, and algae [22]. The mineralization processes in nature are calcification, silification, and iron mineralization [36-38], and the mechanisms involved can include extracellular and intracellular biomineralization [22].
Three types of bacterially mediated biomineralization processes have been described [39]: 1) biologically controlled mineralization, where specific cellular activity directs the nucleation, growth, morphology, and final location of minerals; 2) biologically induced biomineralization, where biological activity modifies chemical conditions in the environment, e.g., pH shifts or redox transformations; and 3) biologically influenced biomineralization, where passive mineral precipitation occurs in the presence of organic matter, such as extracellular polymeric substances (EPSs), which influence the crystal morphology and composition. Additionally, the term organo mineralization has been coined to define biologically influenced and biologically induced biomineralization [39]. Perhaps this latter definition is most suitable for CEDARST in teeth with AP, given the characteristics of this pathological mineralization, i.e., passive mineral precipitation by minerals coming from the saliva into the AP lesion, through a sinus tract (as most CEDARST case reports have been associated with sinus tracts) [15, 16]; as well as biological activity control from the bacteria established in the extraradicular biofilm, and, potentially, from the host. However, not every sinus tract leads to CEDARST, and other sources supplying the required minerals should be considered; specifically, inflammatory exudate from the periapical lesion, and periradicular tissue fluids saturated with minerals from bone solubilization, due to the AP inflammatory resorption process [15].
Although bacteria are believed to be only passively involved in the formation of dental calculus [27], it should be noted that some oral microorganisms have the ability to form intracellular and extracellular apatite crystals [40, 41] and so this kind of microbial calcification could eventually contribute to CEDARST formation. Neisseria flava, Aggregatibacter segnis, Streptococcus tigurinus, and Morococcus cerebrosus are bacteria that have been identified in vitro as contributors to the calcification of supragingival calculus [42]; it could be expected that these bacteria contribute in CEDARST formation, considering the saliva–sinus tract relationship. On the other hand, urease-producing bacteria, like Proteus spp., and the genera Providencia, Morganella, and Staphylococcus, have been associated with urinary calculi formation, through struvite biomineralization [25, 43]. Although struvite has not yet been described in CEDARST formation, there is a reasonable chance that this mineral contributes to this pathological mineralization, because Proteus spp [43], and the genera Providencia [45, 46], Morganella [47], and Staphylococcus [44] have all been identified in extraradicular biofilms, which are associated with cases of persistent AP.
Finally, microbial metabolism can provide a favorable environment for mineral precipitation [48]. Some researchers have demonstrated that microbial carbonic anhydrase significantly increases the rate of precipitation of carbonate minerals [49]. Additionally, on the host´s side, osteoclasts, the prime cells responsible for the periapical lesion formation, express carbonic anhydrase in the bone resorption process [50]. However, the carbonic anhydrase contribution in CEDARST has not yet been evaluated.
3.2. Role of EPSs in Biomineralization
Biofilms represent microbial communities that grow on many surfaces [51]. In such communities, microorganisms are encased in an organic matrix, in which the latter contains a variety of extracellular polymeric substances (EPSs) produced by the former, as well as other potential organic and inorganic compounds from the local environment [52]. Although the EPS composition varies with the structure and physiology of the component microorganisms, it may include a variety of capsule polysaccharides, extracellular DNA, secreted proteins, lipids, and—frequently—outer membrane vesicles (OMVs) [53].
Microorganisms can influence biomineralization by modifying milieu supersaturation levels through metabolic activity, as well as through the production of EPSs, which play an important role in the biomineralization process [39, 54]. EPSs can impact nucleation by stabilizing the first mineral seeds [39], possibly on account of their strong adsorption capacity (they are often anionic) for a large number of cations, and thereby act as a nucleation site in many environments [55]. It has also been evidenced that capsule polymers can adopt a secondary structure in the presence of some metal cations [52]; by such means, the former may influence mineral formation, considering their charge orientation and coordination chemistry [25]. Additionally, it has been stated that organic substances facilitate a decrease in the active energy of nucleation [56], so EPSs may also exhibit the same effect [57].
Bacteria have been detected as biofilm on the apical external root surface of teeth [12, 13, 45, 58, 59]; it can therefore be inferred that one or several components of EPSs in CEDARST are pivotal nucleation sites. It is also well established that bacterial biofilms are a common cause of persistent infections in the human body [51], as is the case in CEDARST.
Finally, it is important to note that, in general, fungi secrete large amounts of extracellular proteins with diverse functions including the reduction of soluble metal ions to their insoluble form and, eventually, to nanocrystals [60]. In this regard, as mentioned above, fungi are microorganisms that are potentially related to AP and might eventually contribute to the biomineralization role of EPSs in CEDARST. In addition, some filamentous fungi (Aspergillus niger, Aureobasidium pullulans, Cladosporium sphaerospermum and Exophiala jeanselmei) have been isolated from teeth with AP. These fungi are all able to produce melanin [10], which is a pigment and also a recognized virulence factor [61]. This melanin might contribute to the color appearance of CEDARST, which has been described as having a brownish-to-black color, as it was in the case described in this paper.
3.3. Roles of Nanobacteria and Nano-sized OMVs in Biomineralization
In 1998, a pioneering study described the presence of nanometer-scale objects—referred to as nanobacteria—and identified these structures as potential causative agents of urinary calculi [62]. However, there were some controversial issues with this work. First, the DNA isolated and sequenced in this study was identical to a common PCR contaminant [25]. Second, it was shown that aggregation of polysaccharides with Ca2+ could produce nanosized objects resembling nanobacteria [63]. Third, nanobacteria have been reported in environments in which normal-sized bacteria also reside [64]. In light of these concerns, it may be more likely that nanosized objects of < 250 nm diameter are microbial fragments, or small aggregates of minerals and organic matter, rather than independent lifeforms (nanobacteria) [65].
OMVs are nanosized (20–250 nm) spherical structures [66] that have been shown to be present in biofilms in both environmental [52] and medical [67] fields. They are produced by many Gram-negative bacteria and have been associated with microbial competition, predation, genetic exchange, quorum sensing, and interspecies communication [68]. OMVs contain peptides, nucleic acids, and, inherently, a surrounding membrane component consisting of lipopolysaccharides (LPSs) from the Gram-negative outer membrane [25, 67]. In morphology, they strongly resemble nanobacteria, which are locally influenced by mineral formation and solution chemistry [25]. Notably, some studies [25, 69, 70] have described a potential role of OMVs in the formation of urinary calculi. In this regard, it has been suggested that OMVs with crystal-promoting chemistry from some bacteria would enhance nucleation and growth, whereas OMVs from other bacteria, with crystal-inhibiting structures, would interfere with struvite nucleation [25], and that these opposing behaviors would be based on the LPS chemistry of OMVs and on other molecules from the local microenvironment [25]. This may suggest a potential mechanism in CEDARST formation, especially if we consider that Gram-negative bacteria have been observed predominantly among the extraradicular biofilm-forming bacteria associated with refractory AP [45]. However, further research is needed to confirm this hypothesis.
3.4. What Biominerals are Precipitated in CEDARST?
Although biomineralization of minerals induced by microorganisms has been widely investigated and reported [71, 72], its mechanisms and the characteristics of the biominerals precipitated in CEDARST need to be further explored. A recent CEDARST case report [16], using electron probe microanalysis, evidenced that calcium and phosphorus were the primary inorganic elements in the extraradicular deposit, showing that the molar Ca/P ratio of the deposit (1.25) was similar to that of dentine (1.32) and cementum (1.33), and confirming its calcified nature and similarity with the molar Ca/P ratio of dental calculus [73].
It has been evidenced that dental calculus involves four different crystals of calcium phosphate: brushite, octocalcium phosphate, whitlockite, and hydroxyapatite [74]. These minerals are initially formed as amorphous calcium phosphate, followed by intermediate calcium phosphate phases that finally transform to apatite [75]. Considering the calculus-like appearance of CEDARST, its location, and the fact that passive mineral precipitation is caused by minerals coming from the saliva into the apical lesion, as mentioned above, a similar crystal composition might be expected. However, until now, no study has confirmed the crystal composition of CEDARST. It should also be stressed that biomineralization is a process closely affected by such factors as pH, temperature, and ion concentration, as well as by enzymes that hydrolyze mineralization inhibitors [76]. All these determinants could influence the type of minerals precipitated in CEDARST.
Finally, it should be noted that minerals formed by biomineralization are not always strongly connected to cells, thus complicating the assessment of microbial-mediated minerals. In addition, the impact that biomineralization may have on microbial communities has yet to be addressed [77]. However, the rarity of CEDARST limits the potential for future studies of this issue.
3.5. Inhibitors of Mineralization
Although EPS, in the form of extraradicular biofilm, has been confirmed in teeth with AP by some authors [12, 13, 45, 58, 59], as mentioned above, clinically, not all teeth with AP develop CEDARST. For this reason, it could be speculated that inhibitors of mineralization from the host do not work efficiently and locally in some teeth affected by AP, thus contributing to CEDARST formation in some way. Calciprotein particles (CPPs) are dense membrane-less particles of mineral-binding proteins and calcium phosphate that are present in the blood and abundant in the extracellular fluids of mammals. An example is the hepatic glycoprotein fetuin-A, which forms nanoscale colloids with calcium phosphate [78, 79]. CPPs stabilize fluid phases and help maintain local mineral supersaturation during biomineral formation, solubilizing excess biominerals and thus preventing pathological mineral deposition in the extracellular space [79].
Mineral chaperones mediate transient solubility and stability of liquid mineral phases in situations of high supersaturation [80-83], complexing mineral ions and thus effectively lowering the availability of mineral solutes [78]. These issues are important, given the pivotal role of solubility phenomena in pathological mineralization processes [84]. For this reason, mineral chaperones have been cited as a prime explanation for pathological mineralization outside the skeleton, and designated as inhibitors of calcification [85]. Osteopontin [86] and fetuin-A [87] are examples of these chaperones, and these also play important roles in promoting bone destruction in AP [88] and periodontal disease [89], respectively. Because of this, it might be expected that when they do not work efficiently, they contribute to CEDARST generation.
Contrarily, bacterial destruction of saliva´s crystallization inhibitors (pyrophosphate, statherin, and proline-rich-peptides) has also been evidenced as a contributing factor in dental calculus formation, in light of their phosphatase and protease activities [75]. Some researchers have shown that bacterial pyrophosphatases contribute to dental calculus formation by hydrolyzing pyrophosphate [90, 91]; Corynebacterium matrucchoti, a bacteria found in dental plaque and calculus, has been proposed as one such contributor [90]. Additionally, it has been evidenced that bacterial protease, e.g., from Bacteroides loescheii, also contributes by degrading salivary protein inhibitors of calcium phosphate precipitation [92]. In this regard, it could be speculated that increased bacterial destruction of saliva´s crystallization inhibitors would contribute to CEDARST formation locally in the AP microenvironment. Finally, the above-mentioned potential inhibitory role of OMVs should also be recalled.
3.6. How Do Bacteria Circumvent the Immune System in CEDARST?
One of the puzzling issues raised by CEDARST is that, despite the plethora of innate and adaptive immune cells/molecules involved in AP [2], bacteria from this calcified biofilm can circumvent such immune mechanisms, so that CEDARST can persist even without treatment. For sure, bacteria have developed a variety of extremely sophisticated systems to evade host immune responses and establish infections in the body [93-95]. It can therefore be inferred that some of these systems/mechanisms are used by bacteria from CEDARST biofilm, especially given its calcified nature.
Notably, glycan-mediatedhost–pathogen interactions can benefit the pathogen, host, or both, given that glycans are highly diverse and ubiquitous in biological systems, being expressed on the surface of nearly all host and bacterial cells [96, 97]. Bacterial glycans play a vital role in bacterial colonization, invasion, and/or immune evasion. Some bacteria express cell-surface glycans that are pivotal for successful colonization on/in host cells; some express molecular mimicry of host glycans on their cell surfaces to subvert host immune surveillance; others cover their surface with layers of glycans to hamper host recognition of common pathogen-associated molecular patterns [96]. However, although host glycans and lectins play a crucial role in inducing bactericidal immune responses, many microorganisms have developed evasion mechanisms [97], and noncanonical variants of peptidoglycans [98, 99] or lipopolysaccharides [100, 101] can be expressed by some bacteria for immune evasion and/or successful colonization. Finally, many Gram-negative bacteria have evolved to express capsular polysaccharides for pathogen survival and/or immune evasion [102, 103].
For the purpose of immune system evasion, bacteria can also change their surface proteins or secrete proteases (intracellular and extracellular) that target pivotal host defenses, e.g., antibodies, complement factors, or antimicrobial peptides, thus allowing bacteria to remain undetected [104]. Furthermore, extracellular proteases are involved in biofilm adhesion, formation, modulation, and dispersion as well as in the breakdown of host extracellular matrix during colonization [105-107], and so these too could play a role in the CEDARST conundrum. However, the organic composition of the latter has not yet been characterized, and this is a study area worthy of further research.
CONCLUSION
The development of CEDARST seems to depend on a fine synergic control exerted by specific microorganisms (mainly bacteria) on this pathological mineralization, as well as on the local chemical microenvironment of the apical lesion of the tooth affected by AP. It is striking that this pathological mineralization develops in a specific tooth, in patients whose periodontal health is otherwise good, even with respect to the affected tooth itself (no deep periodontal pockets reaching the apex). Microbial implications and inhibitors of mineralization seem to play a dominant role in CEDARST biomineralization. Importantly, the clinical case here reported clearly shows that endodontic surgery represents an alternative in cases of endodontic failure, which are not treatable in a non-surgical way, e.g., when CEDARST is present.
Both local and environmental conditions, and the relationships between them, appear to control the nucleation, growth, and evolution of the pathological mineralization itself. In this regard, proteomic profiling could be a promising method of identifying CEDARST, because of the unique characteristics and performance of many of its protocols [108]. Additionally, cryogenic electron microscopy (cryo-EM) has contributed dramatically to bio-mineralization research, because it preserves materials in their native hydrated status and enables the direct observation of mineral precursors at different stages and with nanoscale spatial resolution [76], even at an atomic scale, if some adjuncts are incorporated [109]. Cryo-EM is, therefore, another potential tool for a better understanding of CEDARST, especially theorganic–inorganic phase interaction and the organic macromolecules regulating biomineralization, i.e., proteins and polysaccharides, even in their 3D structure [76].
A further approach might follow from the consideration that X-ray powder diffraction (XRD), scanning electron microscope (SEM), energy dispersive spectrometer (EDS), Fourier-transform infrared spectroscopy (FT-IR), and X-ray photoelectron spectroscopy (XPS) have all been used to determine hydrate phase, mineralogy, morphology, elemental composition and organic substances within minerals [57, 110]. Such techniques could provide important information concerning CEDARST, although the rarity of the latter might serve to limit the scope of future investigations. Because of this, animal models and in vitro studies might also contribute to our understanding of this pathological mineralization. In addition, deep learning models have been used in the prediction of crystal structures [111], and so the use of such models and other computational methods [112] might also add valuable data. Finally, a more thorough understanding of CEDARST formation could be attained as a result of multidisciplinary approaches. Indeed, the concerted contributions of mineralogists, endodontists, biologists, and other academic experts should surely be pursued in the future, to solve the biomineralization conundrum of this pathological mineralization, i.e., its nucleation, crystal growth, aggregation and binding, and retention on the tooth´s apical root surface.
LIST OF ABBREVIATIONS
CEDARST | = CAlcified Extraradicular Deposits on the Apical Root Surface of Teeth |
RCT | = Root Canal Treatment |
CPR | = Conventional Periapical Radiography |
AVAILABILITY OF DATA AND MATERIALS
The data and supportive information are available within the article.
ACKNOWLEDGEMENTS
Declared none.