All published articles of this journal are available on ScienceDirect.
A Novel Perspective on Tissue Engineering Potentials of Periodontal Ligament Stem Cells
Abstract
It is challenging to completely and predictably regenerate the missing periodontal tissues caused by the trauma or disease. To regenerate the periodontium, there is a need to consider several aspects that co-occur with periodontal development. This study provides an overview of the most up-to-date investigations on the characteristics and immunomodulatory features of Periodontal Ligament Stem Cells (PDLSCs) and the recent interventions performed using these cells, focusing on cell survival, proliferation, and differentiation. Keeping in mind the relationship between age and potency of PDLSCs, this work also demonstrates the necessity of establishing dental-derived stem cell banks for tissue regeneration applications. The data were collected from Pubmed and Google Scholar databases with the keywords of periodontal ligament stem cells, tissue engineering, characteristics, and stem cell therapy. The results showed the presence of wide-ranging research reports supporting the usability of PDLSCs for periodontal reconstruction. However, a better understanding of self-restoration for adequate regulation of adult stem cell growth is needed for various applied purposes.
1. INTRODUCTION
The gingiva generally shows the first signs of inflammation through disease processes in the oral cavity. Therefore, knowledge about the structure of periodontium is necessary for understanding the effects of any disease process. Periodontium is a complicated structure in the oral cavity that contains soft (gingiva and periodontal ligament) and hard (cementum and bone) tissues [1]. Prominent functions of Periodontal Ligament (PDL) include supporting the tooth structure and protecting nerves and blood vessels within it from injury through mechanical loading [2]. It is acknowledged that PDL has significant roles in homeostasis, sensation, nutrition of teeth, protection against oral cavities' pathogens [3, 4], and renewal of periodontal tissue by providing the surrounding tissues with stem/progenitor cells [5].
Periodontal disease includes a variety of diseases that are caused by inflammation. Periodontal diseases have distinctive features, such as destroying the supporting tissues of the teeth and chronic inflammation commenced with the infection caused by bacteria [6]. If this inflammation is not treated and eliminated, it can cause attachment loss, bone resorption, and eventually tooth extraction [7]. A decline in individual living standards through significant aesthetic problems and dysfunctions is caused by tooth loss [8]. Scaling, root planning, and open-flap debridement are examples of conventional therapies that dentists achieved to decelerate periodontitis progression. Other periodontal regenerative therapies, including Guided Tissue Regeneration (GTR) and bone grafting, have commonly been used in clinics [9]. However, the results of these treatments have been limited since they have not reached a complete restoration of the periodontium [10]. Tissue Engineering (TE) is an alternative method that can ease the regeneration process of periodontal tissues [11]. TE is an advanced field of regenerative medicine that denotes the practice of combining scaffolds, cells, and biologically active molecules into functional tissues. The aim of TE is to gather functional constructs that restore, maintain, or recover damaged tissues or whole organs [12].
Stem cells and their derived products are great promises for novel medicinal management. Stem cell therapy, also recognized as regenerative medicine, endorses the repair response of diseased, dysfunctional or injured tissue using stem cells or their derivatives. Up to now, the various stem cells have extensive use in tissue engineering, including Mesenchymal Stem Cells (MSCs), Adipose tissue-derived Stem Cells (ADSCs), and other tissue-specific somatic stem cells [13]. MSCs, as multipotent cells, have the capability of differentiation into multiple lineages depending on the signals received from encircling the microenvironment [14].
Five disparate MSC sources have been detected in human postnatal dental tissue, including Periodontal Ligament Stem Cells (PDLSCs), Stem cells from Human Exfoliated Deciduous teeth (SHED), Dental Pulp Stem Cells (DPSCs), Dental Follicle Precursor Cells (DFPCs), and Stem Cells from the Apical Papilla (SCAP) [15, 16]. Fig. (1) shows the process for dental stem cell-based tissue engineering.
Although the number of PDLSCs that can be obtained by a single sample is limited [3], these stem cells can be easily isolated and expanded in vitro [17] Fig. (2). PDLSCs are mainly derived from the mid-third section of the root surface after permanent tooth extraction. Harvesting PDLSCs is not so difficult since they can be derived from both the surface of the root and the alveolar bone [18]. PDLSCs isolated from the remnants of PDL on the alveolar bone surface of extraction sockets have revealed a more promising osteogenic/adipogenic differentiation capability than those from the root surface [18, 19].
A prominent source of MSCs is provided by PDL to their approachability and obtainability for autologous transplantation [18]. This type of MSCs is capable of multipotent differentiation, including osteogenic, adipogenic, myogenic, and chondrogenic commitment. Besides, these mesenchymal lineages, through the ectomesenchymal origin of PDLSCs, can differentiate into neuronal phenotypes [20-22]. It has been substantiated that various paracrine factors with different functions, such as immunomodulation, anti-apoptosis, and anti-inflammation, are secreted by PDLSCs, and the secreted factors of surviving cells in the transplanted site show positive effects on periodontal wound healing [23, 24]. Some problems have been discovered associated with the use of PDLSCs, such as limitations in the number of stem cells obtained by a single sample [3], sensitive condition of stem cells according to donor quality [25], and tumorigenesis [5].
The current study investigated the PDLSCs from multiple viewpoints, including markers, immunomodulation, differentiation, aging, and tissue engineering.
2. MARKERS FOR PDLSCs
Markers that have been reported in numerous studies are Cluster of Differentiation (CD)105 [15, 16, 18, 22, 23, 26-38], CD90 [15, 16, 18, 22, 23, 26, 28, 29, 31-34, 36-40], CD73 [15, 22, 27-29, 31, 34, 39], Stromal Precursor Antigen-1 (STRO-1) [16, 30, 31, 35, 37, 38, 41, 42], CD146 [16, 30-33, 35, 37, 38, 41, 42], CD29 [16, 31, 33, 35, 38, 41, 42], CD13 [16, 31, 33, 36], CD44 [15, 16, 30, 31, 33, 34, 41], CD166 [16, 22, 31, 33] but lack expression of CD45 [15, 22, 28, 29, 32, 34, 36, 38, 40, 42], CD34 [15, 22, 28, 29, 32, 34, 37, 42], CD14 [22, 28, 29, 38], CD19 [28, 30, 34], CD31 [22, 37, 38, 40] (Fig. 3).
In some studies, PDLSCs are positive for CD9 [33], CD10 [31, 33], CD49d [33], STRO-4 [41], STRO-3 [31], CD106 [31], CD349 [31], CD26 [31], CD71 [31], and Mesenchymal Stromal Cell Antigen-1 (MSCA-1)/Tissue Nonspecific Alkaline Phosphatase (TNAP) [31] but negative for CD11b [28, 34], Human Leukocyte Antigen (HLA)-DR [22, 34], HLA Cl II [28], CD79a [22, 28], CD40 [16, 22], CD80 [16, 22], CD86 [16, 22], CD54 [22], and CD20 [29].
3. PDLSCs AND IMMUNOMODULATION
Immunomodulation is a favorable characteristic of PDLSCs. Studies have shown that the expression of immune co-stimulating factors is absent in PDLSCs. Furthermore, PDLSCs could inhibit immune cell proliferation [43, 44]. This valuable property might be beneficial for stem cell therapies in periodontal tissue regeneration since the inflammatory environment in periodontitis suppresses the physiological repair procedures, including stem and mature cells [45]. Besides cell regeneration, PDLSCs display immunomodulatory features, which are evaluable through immunomodulatory function expressing HLAE, HLAG and indoleamine-pyrrole 2,3-dioxygenase 1 (IDO1), IDO2 [46].
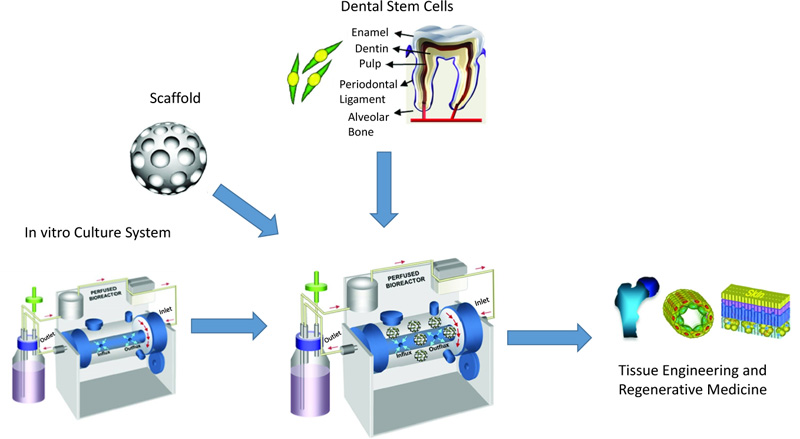
Reprinted from Tissue Engineering Part B: Reviews, 18, Kim BC, Bae H, Kwon IK, Osteoblastic/cementoblastic and neural differentiation of dental stem cells and their applications to tissue engineering and regenerative medicine, 235-544, 2012, with permission (Under the terms of the Creative Commons Attribution 4.0 International License (Creative Commons Public Domain Mark 1.0), which permits unrestricted use.)
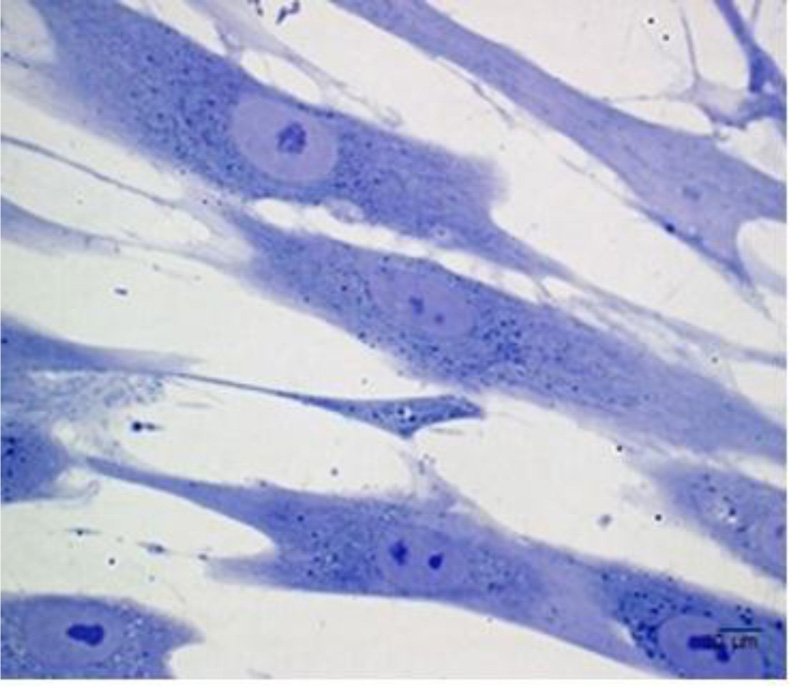
Reprinted from European Cells and Materials, 32, Diomede F, Zini N, Gatta V, Human periodontal ligament stem cells cultured onto cortico-cancellous scaffold drive bone regenerative process, 181-201. 2016, with permission (under the terms of the Creative Commons Attribution 4.0 International License (http://creativecommons.org/licenses/by/4.0/), which permits unrestricted use.)
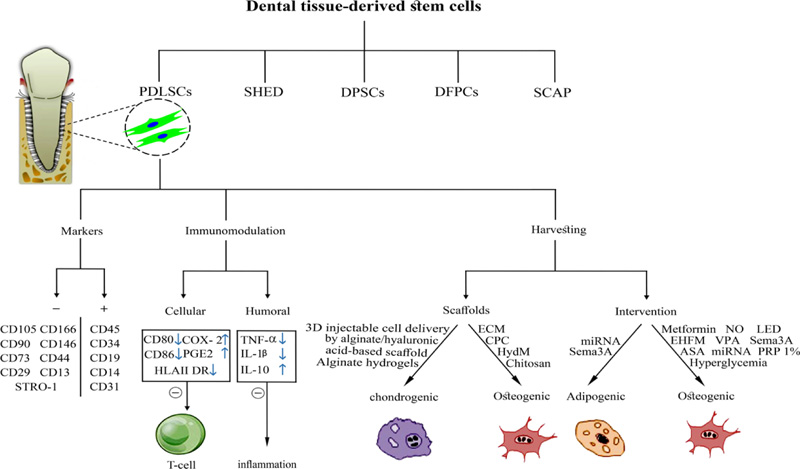
Besides their immunogenicity, an immunosuppressive possession with action on B and T cells should facilitate the use of allogeneic PDLSCs for periodontal regeneration [47]. Firstly, owing to co-stimulatory molecules of T cells (CD80 and CD86) and HLAII DR absence, the immunogenicity of PDLSCs is low. Secondly, due to the up-regulation of prostaglandin E2 (PGE2) and cyclooxygenase-2 (COX-2), PDLSCs restrain allogeneic T cell proliferation [48]. PDLSCs enhance the production of regulatory T cells (T-regs) in healthy tissue. In inflamed periodontium, PDLSCs have a low potential for induction of T-regs. Some studies also demonstrated reduced inhibitory effects on T cell proliferation [49]. Furthermore, PDLSCs blocked the activation of B cells by programmed death-1 [50].
Wang et al. proved that PDLSCs might decrease the apoptosis of neutrophils via interleukin-6 (IL-6) [51]. PDLSCs can be a valuable source for reducing the autoimmune disease destruction in Type 1 Diabetes (T1D) while they have an immunosuppressive impact on monocyte-derived dendritic cells (mDCs) in T1D patients [52]. Furthermore, PDLSCs may induce macrophage polarization to the M2 phenotype. This shift to M2 macrophages in the first stages of tissue regeneration is contributed to the promoted periodontal tissue regeneration [53, 54]. Collectively, signals from the PDLSCs could modify the immune-related niche to promote periodontal tissue regeneration. The reports proved the immunomodulatory impact of PDLSCs on both cellular and humoral immunity. It seems that this property is critical for periodontal tissue regeneration in inflamed environments and increases the success rate of cell transplantation.
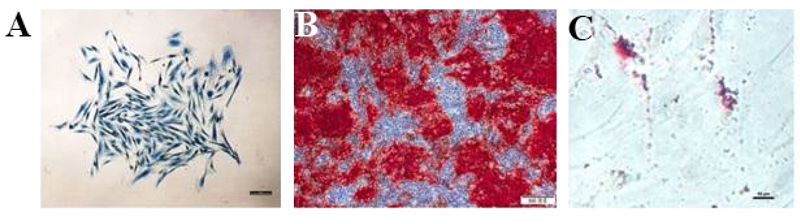
Reprinted from Stem Cell Research & Therapy, 10, Liu J, Chen B, Bao J, Zhang Y, Lei L, Yan F, Macrophage polarization in periodontal ligament stem cells enhanced periodontal regeneration, 1-11, 2019, with permission (under the terms of the Creative Commons Attribution 4.0 International License (http://creativecommons.org/licenses/by/4.0/), which permits unrestricted use.)
4. DIFFERENTIATION OF PDLSCs
Moreover, it has been reported that PDLSCs and DPSCs own the potential for osteogenic differentiation [22, 55]. PDLSCs favorably differentiate into osteoblasts and adipocytes [34, 56]. Fig. (4) shows the identification of PDLSCs. Within an osteogenic differentiation growth medium, osteoblasts formation occurs, and mineralization nodules are developed [15]. The capability of adipogenic differentiation is also demonstrated in several PDLSC differentiation studies [22, 34, 55, 57]. Moreover, it has been observed that due to exposure to a specific differentiation medium, PDLSCs would differentiate into chondrocytes [34, 55, 58].
PDL and dental follicles possess multipotent stem cells with differentiation capacity, especially neural cells [15]. The work from Bahrami et al. [15] revealed that the neurogenic differentiation potential of PDLSCs was more than DFPCs. The gene expression level and neural cell protein in PDLSCs were more than DFPCs of third molar [15]. Meanwhile, by plating on cytokine-free laminin-coated plates, impulsive differentiation into neuronal lineages was detected [59]. Also, another study showed that PDLSCs could augment Retinal Ganglion Cell (RGC) viability and axon regeneration [60]. Additionally, Li et al. confirmed odontogenic features of PDLSCs beyond their neuronal differentiation potential [61]. Furthermore, Pelaez et al. [62] illustrated that exposing PDLSCs to short-term mechanical strain induces them to differentiate into cardiac myocytes. PDLSCs can differentiate into endothelial cells that would provide capillary-like sprouts containing lumens in vitro [63]. Additionally, they possess the potential for differentiation into various PDL cells, such as fibroblasts, osteoblasts, endothelial cells, cementoblasts, and neural cells [64, 65].
PDLSCs have similarities with Bone Marrow-derived Mesenchymal Stem Cells (BMSCs) in some features, such as tri-linage differentiation potential and immunomodulatory and anti-apoptotic function [21, 23]. PDLSCs possess specific additional functions, such as cementum-PDL complex fabricating ability and a tooth-specific structure [22, 24]. Lee et al. [39] suggested that PDLSCs derived from the PDL of supernumerary teeth showed higher colony-forming efficiency than BMSCs and that they could differentiate in both adipocytes and osteoblasts. It has been found that PDLSCs have a remarkable potential to differentiate into PDL, alveolar bone, and cementum compared to BMSCs under regenerative conditions [21]. As reported, PDLSCs are multipotent stem cells. They have osteogenic, adipogenic, chondrogenic, neurogenic, and odontogenic differentiation potential. Additionally, PDLSCs could generate cardiac myocytes, endothelial cells, and cementoblasts. Hence, PDLSCs are appropriate cells in regenerative dentistry that can be used in periodontal disease. Furthermore, they could be used in other body parts for therapeutic goals.
5. EFFECT OF AGING ON PDLSCs
In clinical fields, the importance of PDL in auto-transplantation is known due to the potency of the donor’s PDL to cause the renewal of periodontium components, such as PDL, bone, and gingiva within the recipient site [8]. When the donor age increases, the functional and regeneration potential of stem cells will decrease [13, 66, 67] (Fig. 5). For example, Wu et al. [40] revealed that donor age has a negative impact on the osteogenic potential of PDLSCs. It was found that PDLSCs derived from aged donors had less regenerative potential than those from young donors [25]. Comparing biological characteristics of PDLSCs derived from donors at different ages and according to the results of various studies, proliferation [25, 68-70], migration ability [68], differentiation ability [25, 68, 70], and immunosuppression ability [70] of PDLSCs have a negative correlation with donor’s age.
Consequently, there is a need to design refined protocols for the successful proliferation and differentiation of PDLSCs derived from old individuals as a requirement for autologous cell-based strategies to treat periodontal diseases. Allogenic PDLSCs may be promising in the future by establishing cell banks. Cells from young donors may be beneficial for periodontal regeneration therapy because of their matrix protein production, osteogenic potential, and functional features following sheet fabrication.
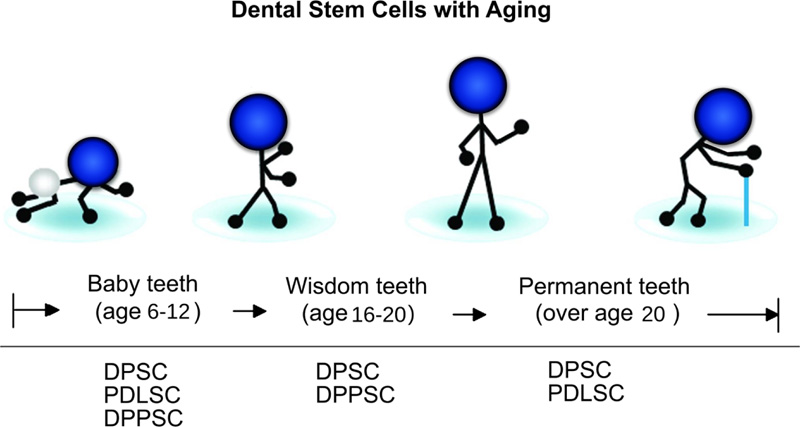
Reprinted from Tissue Engineering Part B: Reviews, 18, Kim BC, Bae H, Kwon IK, Osteoblastic/cementoblastic and neural differentiation of dental stem cells and their applications to tissue engineering and regenerative medicine, 235-544, 2012, with permission (Under the terms of the Creative Commons Attribution 4.0 International License (Creative Commons Public Domain Mark 1.0), which permits unrestricted use.)
6. TISSUE ENGINEERING WITH PDLSCs
Tissue engineering is an interdisciplinary field that includes the basics of engineering and biology to generate biological substitutes [71]. Tissue engineering in dentistry has two goals: first, to create therapeutic approaches to regenerate dental and craniofacial structures, and the second goal is to repair their natural functions [72]. Several types of cell-based regenerative therapies are currently being applied, including scaffold engineering and cell-sheet tissue engineering.
6.1. Scaffolds
The scaffold is an artificial extracellular matrix (ECM) and is a model for cell growth and tissue engineering. Scaffolds should have some vital characteristics, including biocompatibility, biodegradability, adequate mechanical and physical stability, and mimicking the niche to ease cell adhesion, proliferation, differentiation, and tissue regeneration [73-75].
Scaffolds are vital elements for periodontal tissue regeneration to hold cells and contain many bioactive materials [76]. Herein, we reviewed the different scaffolds and cell sheets applied for PDLSCs tissue engineering.
Chitosan is material extracted from invertebrates like crab, and lobster, which is also known as chitin. Chitosan has been used as bone graft material and has characteristics like high viscosity, water-binding properties, biocompatibility, biodegradability, and low cytotoxicity [77]. Ge et al. demonstrated that seeded PDLSCs on a nanohydroxyapatite-coated genipin-chitosan conjunction scaffold showed significantly superior viability and alkaline phosphatase (ALP) activity and up-regulated the bone-related markers to a more extent compared to PDLSCs seeded on the genipin-chitosan framework [78].
Alginate scaffolds are excellent for TE in biological systems since they have natural biocompatibility and easily adjustable immunosuppression and degradation features [79]. Alginate can ease the spatial arrangement of the encapsulated MSCs within its three-dimensional (3-D) structure, causing the creation of a structural organization with similarity to the native in vivo microenvironment [80]. Moshaverinia et al. [81] suggested that chondrogenic differentiation and viability of PDLSCs encapsulated within Arg-Gly-Asp (RGD)-coupled alginate-hydrogel scaffold have been enhanced both in vitro and in vivo. In an injectable approach, encapsulated MSCs demonstrated high levels of proliferation, viability, and chondrogenic differentiation ability. Moreover, PDLSCs with transforming growth factor beta (TGF) b3-loaded RGD-modified alginate microspheres are favorable alternatives for tendon regeneration [17]. In another study, PDLSCs encapsulated in RGD-coupled alginate microspheres presented a moderate ability for osteodifferentiation [82].
HydroMatrix (HydM) is an advanced injectable peptide nanofiber hydrogel presented recently for cell culture. PDLSCs can adhere, survive, migrate, and proliferate on HydM, and this gel also enhances their osteogenic differentiation [83].
Collagen is one of the most broadly used natural scaffolds. It has been observed that the combination of collagen scaffolds with platelet growth factors and PDLSCs could not significantly improve osteogenic regeneration [84]. Due to their anti-inflammatory and regenerative qualities, natural ECM scaffolds are considered superior alternatives to type-I collagen (COLI) membranes [85, 86]. ECM scaffolds are derived from the dermis, small intestine, and pericardium. ECM molecular composition comprises various structural proteins, including COLI, proteoglycans, glycosaminoglycans, glycoproteins, cytokines, and growth factors that are expressed as guidance of cell behavior [87, 88]. An increase in viability, proliferation, and reduction in apoptosis compared to PDLSCs treated with COLI are caused by incubation with ECM. Additionally, co-culture with ECM membrane enhances PDLSC migration and bio-attachment. The ECM membrane could be used as an appropriate scaffold in the GTR application for periodontal disease treatment [36].
The amniotic membrane is a biological membrane that encircles the amniotic sac [89]. The amniotic membrane has several biological features appropriate for periodontal regeneration, including low immunogenicity, anti-fibrosis, anti-inflammation, and being rich in ECM constituents. Furthermore, this membrane can maintain close contact with the transplanted area due to its flexible nature [90, 91]. Amniotic membranes can be used as scaffolds and can serve as a substantial material in TE [92].
Calcium Phosphate Cement (CPC) can be used as an excellent scaffold material for dental and craniofacial treatments [93]. CPC has potent biocompatibility, osteoconductivity, osteoinductivity, and bioactivity [94, 95]. In a study, PDLSCs were cultured on a Biphasic Calcium Phosphate (BCP) scaffold, and the results exhibited that the PDLSC-seeded BCP enhanced periodontal tissue regeneration. Moreover, new bone formation and collagen fibers were also observed [96].
6.2. Cell Sheet Engineering
Cell sheet engineering is a modern method in TE; it can be used in various fields like corneal surface reconstruction and non-invasive endoscopic transplantation [97, 98]. Proteolytic enzymes are one of the privileges of cell sheets concerning the unfavorable shortage of degrading cell adhesion molecules and the deposited ECM [99]. Therefore, compared to scaffold-based tissue engineering, a cell sheet can bring healthy cells connected by intact cell-cell interactions and ECM proteins. It is also proved in some investigations that they have an important role in periodontal tissue regeneration [35, 100]. Iwata et al. confirmed that the autologous PDL-derived cell sheets are safe and efficient in severe periodontal defects. Besides, the stability of these sheets has been shown during long-term follow-up [101]. A nanopatterned substratum activated with thermos-responsive polymers eased the reproducible and robust production of patterned cell sheets utilizing PDLSCs. The PDLSCs showed accelerated impetuous monolayer creation, as well as enhanced gene expression patterns related to PDL regeneration compared to the control group [30].
Platelet-rich plasma (PRP) is a common autologous platelet concentration with different growth factors. It contains growth factors, such as platelet-derived growth factor (PDGF), TGF-β, vascular endothelial growth factor (VEGF), fibroblast growth factor-2 (FGF), and insulin-like growth factor I (IGF). These types of growth factors can increase the aggregation of cells in a periodontal defect [35]. PDLSCs treated with 1% PRP exhibited the highest osteogenic differentiation potential and superior periodontal tissue regeneration capability [35]. PRP may increase the proliferation and ECM release of PDLSCs in the process of cell sheet formation [35]. Platelet-rich fibrin (PRF) is known as the second generation of platelet concentrate. It has superior characteristics compared to conventional PRP, including simplicity of preparation and a lack of the biochemical handling of blood [102]. Notably, the 3D structure of this newly developed material is ideal for directing therapeutic stem cells to a particular site of destruction [103]. In a study, the authors claimed that the novel cell transplant approach using PDLSCs/PRF, containing cell sheet particles of PDLSCs and PRF granules, may stimulate periodontal defect healing and PDL regeneration [104].
Based on the reviewed literature in this section, the various existing scaffolds have shown great potential for periodontal regeneration. The above-reviewed scaffolds are mostly biodegradable biomaterials. They degrade in the body once the new tissue is formed. Besides, most of the polymers mentioned above display a sustained degradation process with some degree of controllability [105, 106]. The characteristics of biomaterial scaffolds have mainly been characterized in vitro, providing good fundamental information for future in vivo tests [106].
6.3. Chemical Interventions
The fabrication of functional organs with TE is very challenging. Such barricades consist of inadequate cell migration into and retaining within scaffolds, host inflammatory reactions, inadequate abilities in generating microscale vascularization for mass transfer, various rates of cell multiplication in comparison to scaffold destruction, and the incapability of generating functional tissues with the structural intricacy of native tissues due to scaffold-dependent techniques [107]. Some studies focus on improving the osteogenic potentiality of PDLSCs and modulating the expression profile of growth factor-linked genes [108-110].
Acetyl Salicylic Acid (ASA), known as aspirin, is a commonly used drug globally and is generally useful for the secondary prevention of cardiovascular disease [111]. The observations in the previous studies showed that ASA made a positive impact on reducing the loss of human alveolar bone and enhancing periodontal tissue health [112]. Aspirin can enhance the proliferation of PDLSCs and their osteogenic differentiation potential and can ameliorate periodontal health by stimulating growth factor-associated genes in PDLSCs and improving their osteogenic potential [29].
Vitamin C (Vc) is a water-soluble vitamin essential for immune system functions. It is critical in human health and is profoundly involved in numerous metabolic and signaling routes. It has an antioxidant role with the capability to less Reactive Oxygen Species (ROS). In Vc provided culture medium, Vc is a growth factor to enhance cell proliferation and DNA synthesis [113]. Moreover, in periodontal disease, Vc diminishes the development of the damage process, stimulates the differentiation of PDLSCs [114], and augments the viability of these cells [115]. Wei et al. suggested that Vc enhanced the proliferation ability and osteogenic differentiation of PDLSCs, engaging telomerase activity in PDLSCs, thus indicating the superior potential for regeneration and differentiation [116]. Another study recognized that Vc-treated PDLSCs in a long-term culture preserved a slender morphology, greater growth rate and migration ability, stemness, and osteogenic differentiation potential [117]. The development of a valid approach based on Vc treatment eased the construction of highly practical and functional PDLSC sheets and the subsequent regeneration of periodontal tissues [116].
Metformin (1,1-dimethylbiguanide hydrochloride) is a hypoglycemic medicine vastly consumed by type 2 diabetes mellitus patients [118]. Metformin can be applied for vast purposes, like antitumor, immunoregulatory, and anti-inflammatory functions [119]. It has been used as an additive material to scaling and root planning in the treatment of periodontal tissue in dentistry [120]. A review study concluded that metformin has anti-aging and anti-oxidative effects on PDLSCs [121]. Low amounts of metformin did not affect cell proliferation but hindered adipogenic differentiation and enhanced PDLSCs’ osteogenic differentiation. The results of this study showed that metformin both increases osteogenic differentiation of PDLSCs and protects PDLSCs against oxidative stress-produced harm, indicating that metformin can be greatly useful in increasing bone regeneration in the therapy of periodontitis by PDLSCs [34]. Yang et al. demonstrated that metformin could relieve oxidative stress-induced senescence by autophagy and could, to a degree, recover the osteogenic potential of PDLSCs [122]. The results of Zhang et al. [123] revealed that metformin generates a suitable niche for periodontal tissue regeneration via stimulating the proliferation and migration of PDLSCs.
MicroRNAs (miRNAs) are a new type of posttranscriptional regulators. They are critical elements in regulating cell differentiation [124]. Inflammatory cytokines can control miRNAs and cause some inflammatory diseases [125]. Several types of miRNAs have been investigated in previous studies. MiR-21 may take part in the promotion of the osteogenic differentiation of PDLSCs [126]. Another study demonstrated that miR-21 is a mechano-sensitive gene that has an indispensable role in the osteogenic differentiation of PDLSCs [127]. Yan et al. proved that miR-22 enhanced PDLSC osteogenic differentiation by suppressing histone deacetylases (HDAC) expression [128]. HDAC regulates gene expression by removing negatively-charged acetyl groups from the positively-charged lysine on histone tails, tightening the interaction between histones and DNA and suppressing its access to transcription [129]. HDAC diminished the osteogenic differentiation potential of the PDLSCs under inflammatory conditions. In conclusion, HDAC inhibitors increased the osteogenesis of PDLSCs in vitro and in vivo [32]. On the other hand, some microRNAs showed adverse effects on periodontal tissue regeneration. For instance, miR‐146a exacerbates periodontitis by downregulating IL-17 and IL-35 expressions and proliferates PDLSCs [130]. Besides, miR-132 could hinder PDLSC osteogenesis [131].
PDLSCs constitutively express the chemokine stromal cell-derived factor-1 (SDF-1) that plays a crucial role in promoting cellular viability [132], migration [133] and homing of stem cells through signaling with its cognate receptor, C-X-C chemokine receptor type 4 (CXCR4) [134]. According to Feng et al. [135], SDF-1 plays a role in the protection of PDLSCs against apoptosis caused by oxidative stress by activating extracellular signal-regulated kinase (ERK) signaling. Based on the results of this study, it is suggested that SDF-1 therapy is a promising approach to promote PDLSC survival, which may assist in dental tissue regeneration [135]. In another study, the parathyroid hormone was used in combination with SDF-1. This co-therapy enhanced proliferation, migration and osteogenic differentiation of PDLSCs. Furthermore, it seems that co-therapy has the potential to promote periodontal tissue regeneration since it facilitates the chemotaxis of PDLSCs to the damaged site [136]. Additionally, SDF-1/Exendin 4 co-therapy was reported to have some other positive effects on PDLSC, including increasing ALP activity, mineral deposition and osteogenesis-related gene expression [137]. In conclusion, SDF-1 may promote periodontal tissue regeneration by the means of directing PDLSCs to injured periodontal tissue. SDF-1 enhances the activation and proliferation of PDLSCs and also induces the differentiation of these cells.
Semaphorin 3A (Sema 3A), one of the members of the semaphoring family secreted protein, has essential roles in developing different tissues, such as blood vessels, peripheral nerves, and skeletal tissues [138]. Additionally, Sema 3A functions as an osteoprotective factor by increasing bone production and preventing bone resorption [139]. It plays a vital role in maintaining the stem cell characteristics of PDLSCs. The expression level of Sema 3A was higher in multipotent human PDL cell lines compared to low-differentiation potential lines, and Sema 3A-overexpressing low-differentiation potential PDL clones promoted MSC-related marker expression and improved capacity to differentiate into osteoblasts and adipocytes [140].
Hypoxia has several effects on PDLSCs mineralization, osteogenic potency, and paracrine secretion. In hypoxic conditions, an increase in ALP activity and Runt-related protein 2 (RUNX2), Sp7, osteocalcin and osteopontin (OPN) expression was observed in PDLSCs [141, 142]. Moreover, hypoxia could regulate the expression of RUNX2 in PDLSCs via hypoxia-inducible factor-1α (HIF-1α) and play an effective role in the primary stage of osteogenesis of PDLSCs [143]. Zhang et al. observed an increased 2% O2 PDLSC cell count and osteogenic potential [144]. Hypoxia had a stimulatory effect on PDLSC proliferation, osteogenic differentiation, and migration [145].
Nitric oxide (NO) is a small, diffusible, diatomic, reactive element with various cellular functions in human cells [146]. NO plays a key role in the proliferation and differentiation of stem cells. It has been proved that NO is a negative modulator of stem cell proliferation in physiological concentrations and initiates cell differentiation [147]. Orciani et al. demonstrated that the upregulation in NO production was related to increased alkaline phosphatase activity. This study showed that NO had a key role in the osteogenesis of stem cells, especially PDLSCs [148].
Some researchers developed a new effective mineralization-inducing medium by adding KH2PO4 to the solution [149]. Cell Counting Kit-8 (CCK-8) assay suggested that 1.8 mmol/L KH2PO4 can increase the PDLSCs proliferation in the logarithmic phase of growth [150].
Some studies showed that tuning the settings of light-emitting diode (LED) irradiation, such as wavelength and dose, can significantly impact the proliferation rate and undifferentiated cells’ osteogenic differentiation. The advantages of irradiation could be used to regenerate PDL utilizing LED as a light source of antimicrobial photodynamic therapy (aPDT) [151, 152]. High-power, red LED irradiation increases the proliferation and eventually improves the osteogenic differentiation, mineralization, and ATP levels of PDLSCs [153]. A study by Chaweewannakorn et al. demonstrated that the PDLSCs responded to different LED wavelengths in various ways. 830-nm irradiation demonstrated better results in improving proliferation. Moreover, 630 and 680 nm wavelengths stimulated osteoblastic differentiation [154]. In another study, the results revealed that red LED enhanced osteogenic differentiation of the PDLSCs. The real-time polymerase chain reaction (RT-PCR) results showed upregulation of the expression of osteogenic genes, such as Bone sialoprotein (BSP), OPN, Osteonectin (OCN), and RUNX2, by the red LED. Moreover, red LED at 1, 3, and 5 J/cm2 stimulates proliferation and osteogenic differentiation of PDLSCs [155].
Improving the osteogenic potentiality of PDLSCs and modulating the expression outline of growth factor-linked genes are important stages in tissue engineering processes based on PDLSCs. For example, using continual cell sheets by preserving cellular junctions, endogenous ECM, and imitating cellular microenvironments with regard to different mechanical, chemical, and biological features may be advantageous for cell transplantation.
CONCLUSION AND FUTURE OUTLOOKS
Numerous factors need to be considered for the regeneration of periodontium. So far, there is no clinical evidence for the application of PDLSCs for immunomodulatory periodontology/medicine. Evidence indicates that the periodontal ligament is a replete resource of MSCs. Despite the apparent high regenerating potentiality of this tissue, harnessing and utilizing this capability are not easy for clinical applications. Up to now, oral and dental-tissue-derived stem/progenitor cells have been utilized to study tissue engineering in small and large animal models to evaluate their capability in preclinical utilization. This study provides an overview of the most up-to-date investigations on the characteristics and immunomodulatory features of PDLSCs and the recent interventions performed using these cells, focusing on cell survival, proliferation, and differentiation. Keeping in mind the relationship between age and potency of PDLSCs, this work also demonstrates the necessity of establishing dental-derived stem cell banks for tissue regeneration applications. For regeneration of periodontium, better understanding of the modes of self-restoration action is necessary for sufficient regulation of adult stem cell growth in vitro to produce cells necessary for various applied purposes and regulate stem cells for differentiating and maturating tissue-specific cell types, as well as wound remedy. In addition, the interplay between stem cells and the immune system is necessary, particularly in allogeneic cell populations.
AUTHORS’ CONTRIBUTION
All authors contributed to the drafting and scientific revision of the manuscript. All authors read and approved the final manuscript.
LIST OF ABBREVIATIONS
PDL | = Periodontal Ligament |
TE | = Tissue Engineering |
MSCs | = Mesenchymal Stem Cells |
ADSCs | = Adipose Tissue-Derived Stem Cells |
PDLSCs | = Periodontal Ligament Stem Cells |
SHED | = Stem Cells from Human Exfoliated Deciduous Teeth |
DPSCs | = Dental Pulp Stem Cells |
DFPCs | = Dental Follicle Precursor Cells |
ECM | = Extracellular Matrix |
FGF | = Fibroblast growth factor-2 |
VEGF | = Vascular Endothelial Growth Factor |
IGF | = Insulin-Like Growth Factor I |
SCAP | = Stem Cells from the Apical Papilla |
T1D | = Type 1 Diabetes |
ASA | = Acetyl Salicylic Acid |
miRNAs | = MicroRNAs |
HDAC | = Histone Deacetylases |
CXCR4 | = C-X-C Chemokine Receptor Type 4 |
NO | = Nitric Oxide |
CONSENT FOR PUBLICATION
Not applicable.
FUNDING
The vice chancellor for research at the Tabriz University of Medical Sciences provided financial support for this research that is greatly acknowledged.
CONFLICT OF INTEREST
Dr. Solmaz Maleki Dizajand and Dr. Simin Sharifi are editorial advisory board members of The Open Dentistry Journal.
ACKNOWLEDGEMENTS
Declared none.