All published articles of this journal are available on ScienceDirect.
Comparison of Biocompatibility of Experimental Tricalcium Phosphate Cement versus Biodentin and Mineral Trioxide Aggregate used for Furcation Perforation Repair (in vivo study)
Abstract
Background:
The interaction between the root canal system and the oral cavity caused by iatrogenic perforations significantly affects the treatment outcome and tooth survival.
Objectives:
This study was directed to compare the biocompatibility of an experimental tricalcium phosphate cement versus biodentine and mineral Trioxide aggregate used for furcation perforation repair in dogs.
Methods:
Perforations were done in 60 teeth (premolars and molars) of six adult dogs. Animals were divided randomly into 3 equal groups of 2 animals each according to the post-operative evaluation period of 1 week, 1 month, and 3-months. Each group was further subdivided into 4 subgroups according to either being repaired with the experimental tricalcium phosphate cement (n=6), or Biodentine (n=6), Mineral Trioxide Aggregate (MTA) (n=6), and positive control(n=2). After evaluation periods, tissue blocks were harvested and histologically examined.
Results:
No statistically significant difference was found regarding bone deposition scores and inflammatory reaction in the three groups after 1 week, 1 month, or 3 months. All three groups showed a statistically significant difference between all three time periods. Regardless of the repair material used, inflammation scores at 1-week showed the highest scores, decreasing over time except for the control group.
Conclusion:
The experimental material could be considered as a successful treatment option for repairing furcation perforation.
1. INTRODUCTION
Perforation is an atypical pathway between the root canal system and the surrounding tissues and supporting structures, which are either created by a clinician during the initial penetration into the root canal system, cavity preparation, or by a biological event such as resorption or caries [1]. Root perforation is one of the most common mishaps of endodontic treatment that a general practitioner or even a trained endodontist could encounter. It occurs in approximately 2–12% of endodontically treated teeth [2]. When furcal perforation occurs in the furcation areas of posterior teeth, it can compromise the attachment apparatus and can have an adverse effect on the overall tooth prognosis [3]. When MTA was popularized in 1990, it was the material of choice for all dentinal defects due to its biocompatibility and the ability to induce calcium-phosphate precipitate at the tooth tissue interface. However, this material has some drawbacks, such as slow setting, complicated handling properties [4], and tooth discoloration. MTA angelus is a new formulation of traditional Pro-Root MTA that overcomes its slow setting time, and there is a reduction in setting time from 3 hours for Pro-Root MTA to 10 minutes for MTA angelus, according to the manufacturer [5].
Biodentine, also referred to as ‘dentine in a capsule,’ is a bioactive dentine substitute that has mechanical properties similar to dentin. It is biocompatible and has beneficial effects on living cells [6]. To simplify clinical procedures, biodentine uses modified powder components by adding setting accelerators, softeners, and a pre-dosed capsule formulation in a mixing device, with higher physical properties and a more user-friendly approach [7]. Numerous studies have compared sealing ability and healing after perforation repair using MTA and Biodentine [8]. Calcium silicate cement has become the preferred material for repairing dentin defects where the root canal system interacts with the periodontal ligament because of its ability to induce calcium-phosphate precipitate at the interface with the periodontal tissue and biocompatibility, which leads to bone tissue repair. The material-dentin interface gets better with time which guarantees long-term clinical success with the reduction of risks of marginal percolation [9].
Tricalcium phosphate comprises a biodegradable ceramic, and it was very promising in periodontal therapy due to its compatibility with periodontal tissues [10]. In 2016, Radwan et al. formulated a root-end filling material based on Tri calcium-silicate and Tetra calcium-phosphate, which were prepared from ultra-pure chemical compounds via solid-state reaction at 1450°C and 1500°C, respectively. In their study, they concluded that the experimental material had good physical and mechanical properties as a root-end filling material [11]. Later the same group examined both the biocompatibility by implantation subcutaneously in seven rats and cytotoxicity on Baby Hamster Kidney cells using the MTT assay, and they found no statistically significant difference between the new material and MTA regarding edema formation, inflammation, capsule formation, and calcification. Furthermore, it was considered to be less cytotoxic than MTA [12].
Thus, the objective of this study was to examine the biocompatibility of an experimental tri calcium phosphate cement, which provides a new low-cost option for perforation repair, which is a frustrating problem to dentists in developing countries, and compare it to biodentine and mineral trioxide aggregate. The null hypothesis was that there would be no significant differences in the histological findings among the three materials used.
2. MATERIALS AND METHODS
2.1. Composition of the Experimental Tricalcium Phosphate Cement
Table 1 shows the composition of the experimental tricalcium phosphate cement powder, which was mixed with a liquid (Di-sodium hydrogen phosphate1%). One well-packed scoop of the powder of weight equal to 0.1068 gm was dispensed on a clean, smooth glass slab. One drop weight equal to 0.0227 of the liquid was added to the powder and mixed together until the desired consistency for a repair material was reached.
Constituents | Weight % /gram |
---|---|
Tri-Calcium Silicate | 30 |
Di-calcium silicate. | 30 |
Tri-calcium phosphate. | 35 |
Bismuth oxide. | 5 |
2.2. Sample Selection
The protocol was approved by the medical research ethical committee, National research center (Registration number 17-77). For the study, six male dogs (free-ranging urban dogs) [13, 14] aged 18-24 months and weighing between 10 and 20 kilograms each were obtained from the animal house of Faculty of Medicine, Cairo University and selected as experimental animals, where all captured dogs had veterinary health care. They received all needed medication and were kept in separate cages. Dogs were allowed free access to water and were fed ad libitum. All guidelines regarding the care of animal research subjects were strictly followed in this study.
2.3. Animal Preparation
All procedures were carried out under general anesthesia. Anesthesia was administrated by a veterinarian via intramuscular injection with a mixture of Xylazine (Xylaject50 ml) (Adwia co. S.A.E.10th of Ramadan city, Egypt) and Ketamine (Keiran HCL 500 mg; KUP, Austria, packed by Pharco, Alexandria, Egypt) in a ratio of 2:1. Anesthesia of surgical depth was assessed by loss of the pedal and corneal reflexes and the presence of deep regular breathing. Maintenance doses were given whenever needed.
2.4. Operative Procedures
Antisepsis of the oral cavity was performed with the use of chlorhexidine 2% swapping [15]. The mouth was kept open with the aid of a holding device. After rubber dam application, a coronal access cavity was prepared for each experimental tooth with a sterile round bur (ISO size 012) under water coolant. The pulp chamber was then irrigated with 5.25% sodium hypochlorite solution (NaOCl; Clorox, HC Egyptian company, Cairo, Egypt), and the coronal pulp was completely removed using a spoon excavator (Dentsply/Maillefer Corporation, Zurich, Switzerland). Once the bleeding was controlled, the root canals were explored with a no. 20 K-file (Mani Inc, Tochigi-Ken, Japan] and pulp extirpation was done using no. 30K-files. Coronal flaring of the canals was done using Gates Glidden drills# 2, 3, and 4 (Maillefer Corporation, Zurich, Switzerland). Mechanical preparation of the apical 1/3 of the canals was done by K-Files using the step-back technique. The master apical file sizes ranged from 40-70 according to the size of the initial file. The root canals were dried with paper points (Meta Biomed Co, Korea), and obturation was done with gutta-percha and adseal sealer (Meta Biomed Co, Korea) using lateral condensation technique.
2.5. Furcation Perforation Procedures
A sterile No. 4 round bur (Mani Inc, Tochigi-Ken, Japan) was used to create a perforation of diameter 1.4 mm. The depth of perforation was limited to 2 mm using a stopper fixed to the bur. The hemorrhage at the perforation site was controlled by irrigation with saline and then dried by the use of sterilized cotton pellets.
2.6. Teeth Grouping
A total of 60 perforations were divided into 3 equal groups of 2 animals each according to the post-operative evaluation period into 1 week, 1 month, and 3 months. Each group was further subdivided into 4 subgroups according to either being repaired with the experimental tricalcium phosphate cement (n=6), or biodentine (n=6), or mineral trioxide aggregate (MTA) (n=6), or positive control (n=2). In each dog, 10 teeth were used; 3 teeth for each material and 1 for the control group.
2.7. Perforation Repair
Biodentine (Septodont, Saint Maur des Fosse's, France) and MTA (Angelus, Londrina, PR, Brazil) were mixed according to the manufacturer’s instructions, while for the experimental cement (ECSP), one well-packed scoop of the powder of weight equal to 0.1068 gm was dispensed on a clean, smooth glass slab. One drop weight equal to 0.0227 of the liquid was added to the powder and mixed until the proper consistency was reached.
The materials were lodged in the perforation site using an amalgam carrier and were compacted by a suitable size plugger (Fig. 1). The coronal accesses were sealed using amalgam. A post-operative periapical radiograph was taken for each tooth immediately after perforation was repaired with materials used in the study (Fig. 2). For the control group, the perforation site and the access cavity were left open to the oral environment. A single experienced endodontist (M.B) performed all access cavities preparation, perforations, and perforations repair to avoid inter-operator variability; all steps were done under magnification (2.5X magnification EyMag Smart Loupes; Carl Zeiss Meditec; Jena, Germany).
2.8. Postoperative Care
After the operation, each dog was kept within confinement and watched carefully till recovery, then returned to its cage. To reduce postoperative pain, all dogs received Meloxicam (Metacam 2.5 mg chewable tablets, Boehringer Ingelheim, New Zealand) at a dose of 0.2 mg/kg body weight once daily for 5 days. Dogs were fed a soft diet for 3 to 4 days, followed by a regular diet. They were periodically examined during the evaluation period.
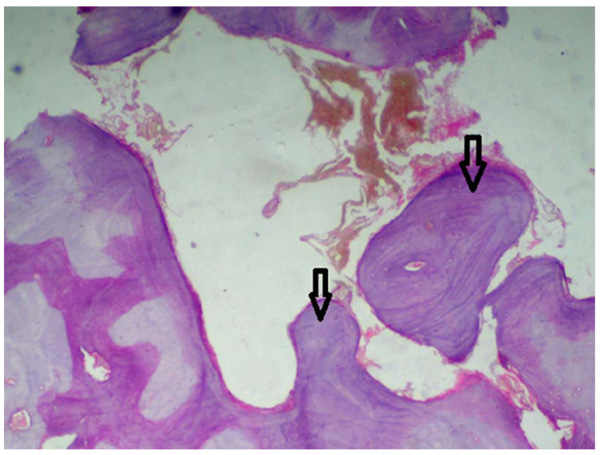
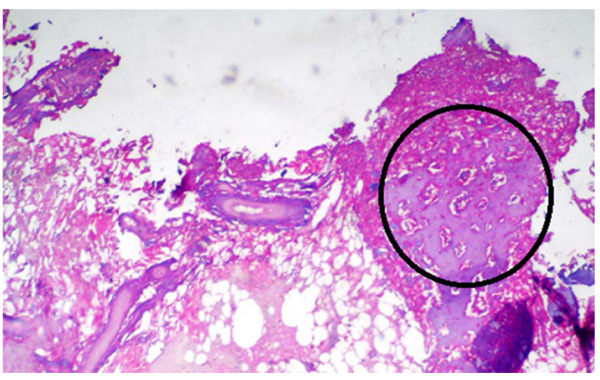
2.9. Histological Preparation
Animal euthanization was essential for histological examination. After the experimental period, animals were sacrificed by anesthetic overdose. Each experimental tooth with the surrounding tissues was cut in a block section. For the preparation of histological sections, the cutting–grinding technique was used [16]. The sectioned specimens were immediately immersed in 10% buffered formalin for fixation for 2 weeks in containers marked for the dog number and the tooth number. Specimens were then removed from the formalin solution and rinsed for four hours under running tap water. Specimens were then decalcified in a solution of 10% formic acid buffered with sodium citrate [17] and then dehydrated in ascending grades of alcohol (50%,75%, and 100%), cleared in Xylene, and were then embedded in paraffin [18]. The paraffin-embedded specimens were serially cut in a buccolingual plane into sections of six-micron thickness and mounted on glass slides. The sections were deparaffinized, hydrated, and stained with haematoxylin and eosin. The stained histological sections were then examined under a light microscope by a pathologist (R.S.E), who was blinded to the study groups to interpret any histopathological changes found in the furcation area.
2.10. Criteria for Evaluation
The histological sections were evaluated for bone apposition and inflammatory reaction and graded according to Al-Hadainy et al.’s scoring system [19].
2.10.1. The Scoring System used for Testing Bone Apposition
Following is the scoring system used for testing bone apposition:
0 = None
1 = Osteoblastic rimming with no osteoid tissue formed.
2 = Heavy osteoblastic rimming with some osteoid tissue.
3 = Heavy osteoblastic rimming with abundant osteoid tissue.
2.10.2. The Scoring System used for Testing Inflammation
Following is the scoring system used for testing inflammation:
0 = None
1 = Small area of minimal inflammation
2 = Small area of inflammation, mainly chronic and slightly of higher intensity
3= Large area of infiltrated cells of moderate-intensity
4 = Large area of acute or chronic inflammatory cells of severe intensity or abscess formation
2.11. Statistical Analysis
The mean and standard deviation values were analyzed for each group in each test. Data were inspected for being normal by Kolmogorov-Smirnov and Shapiro-Wilk tests, and parametric (not-normal) distribution was found. Kruskal Wallis test was used to compare more than two groups in non-related samples. Mann Whitney test was used for comparing two groups in non-related samples. Friedman test was used to compare more than two groups in related samples. Wilcoxon test is used to compare two groups in related samples. The significance level was set at P ≤ 0.05. Statistical analysis was performed with IBM® SPSS® Statistics Version 20 for Windows.
3. RESULTS
A statistically significant difference was seen among the control group and the other groups being tested (MTA, Biodentine, and ECSP) for bone deposition where p<0.001 (Table 2). No significant difference was seen in bone deposition scores of the three groups after 1 week, 1 month, or 3 months. All three groups showed a statistically significant difference between all three time periods. Within MTA, biodentine, and ECSP groups, bone deposition scores at 3 months evaluation period showed statistically significant highest scores. The statistically significant lowest score was found at 1-week then 1-month evaluation period (Figs. 3 and 4).
Table 2.
Variables | Bone deposition | ||||||
---|---|---|---|---|---|---|---|
1w | 1m | 3m | p-value | ||||
Mean | SD | Mean | SD | Mean | SD | ||
Control | 0.00 | 0.00 | 0.00 | 0.00 | 0.00 | 0.00 | 1ns |
MTA | 0.00 | 0.00 | 1.17 | 0.41 | 2.00 | 0.50 | 0.001* |
Bio-Dentine | 0.00 | 0.00 | 0.83 | 0.41 | 1.50 | 0.55 | 0.001* |
Experimental Material | 0.00 | 0.00 | 0.50 | 0.55 | 1.17 | 0.41 | 0.003* |
p-value | 1ns | <0.001* | <0.001* | - |
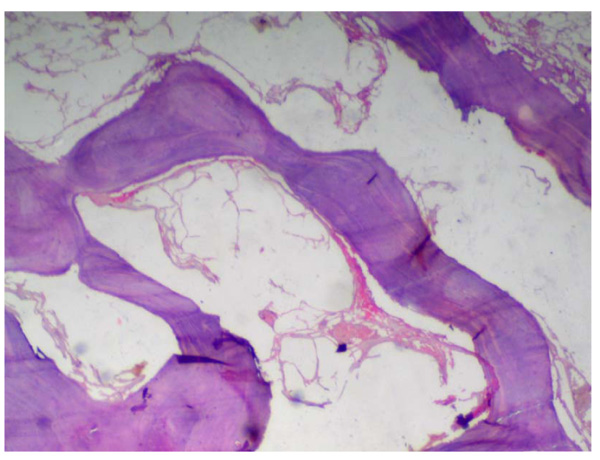
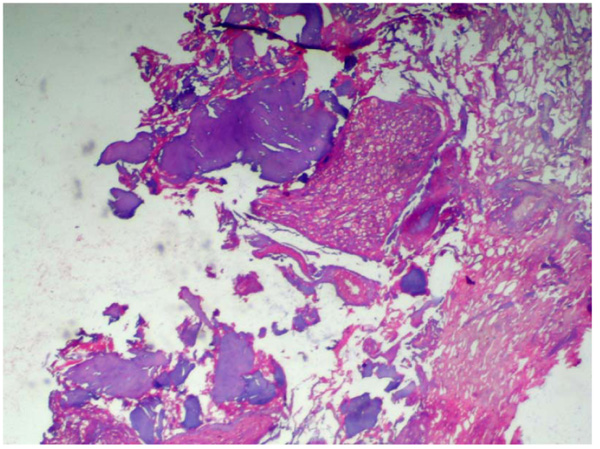
As for the inflammatory response, we found no significant difference in the scores between the 3-groups after 1-week, 1-month, or 3-months. Inflammation scores between all periods in the three groups did not show any significant difference. Within MTA, biodentine, and experimental material groups, inflammation scores at 1-week evaluation period showed the highest scores, and it decreased between 1 and 3-months; the lowest score was found at 3-months period (Table 3, Figs. 5-7).
Variables | Inflammation | ||||||
---|---|---|---|---|---|---|---|
1w | 1m | 3m | p-value | ||||
Mean | SD | Mean | SD | Mean | SD | ||
Control | 2.50 | 0.71 | 3.50 | 0.71 | 4.00 | 0.00 | 0.167ns |
MTA | 1.33 | 0.52 | 1.17 | 0.41 | 1.00 | 0.00 | 0.322ns |
Bio-Dentine | 1.17 | 0.41 | 0.83 | 0.41 | 0.81 | 0.41 | 0.284ns |
Experimental Material | 1.00 | 0.00 | 1.00 | 0.00 | 0.83 | 0.41 | 0.368ns |
p-value | <0.001* | <0.001* | <0.001* |
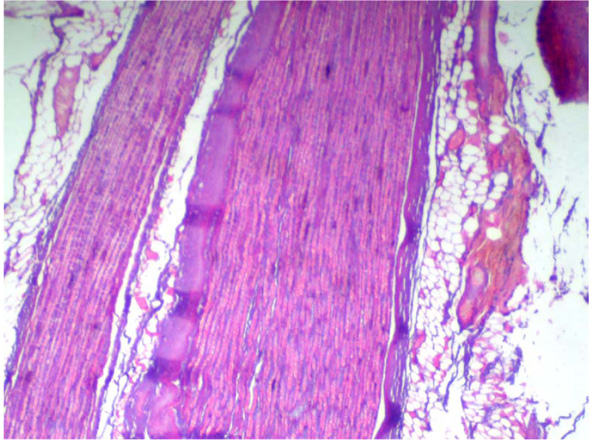
4. DISCUSSION
Furcation perforations have a significant impact on the prognosis of endodontic treatment. They dispose teeth to the periradicular breakdown with subsequent loss of periodontal attachment. This may be difficult to fix in most cases and will eventually lead to tooth loss [20]. Furcal perforation was chosen in this study because of its adverse effect on prognosis [21]. On the other hand, the placement of repairing material can be well controlled by the dentist. Nonetheless, it is difficult to control material extrusion into the periodontium. This can be controlled by placing a bio-inert matrix beneath the repairing material; however, this is a complicated method that does not ensure success [22]. A bio-inert matrix was not used in this study to be able to evaluate the effect of the tested material solely without other variables being involved, besides Al-Daafas and Al-Nazhan claimed that placing MTA only when repairing a perforated furcation without the internal matrix (barrier) under it will result in better healing [23].
An animal model was adopted in this study, as it assists examiners in producing and regulating operative scenarios that are not attainable in clinical setups, such as inducing perforations and providing the benefit of obtaining histologic data that could not be obtained clinically, thus helping in understanding the underlying tissue reaction. Dogs were selected as the animal model, as their teeth have well-developed roots, and the furcation affords proper accessibility and visibility. They have large teeth, which makes the study of tissue reaction easy, and they also provide enough area for perforation formation [24]. Yet, the morphology of the dog’s teeth differs from that of human’s, as they have 2 roots in mandibular premolars, with the furcation being 1 to 2 mm from the cement enamel junction [Furcation lies deeper within the alveolus in humans]. Therefore, any method that produces acceptable results in dogs will probably provide a better result in humans [25].
Alhadainy et al. [17] and Himel et al. [10] recorded a higher success rate in molars than premolars. However, Salman et al. [23] found no difference in success rate between molars and premolars. The findings of the current study showed that there is no relationship between tooth width and success rate.
For ethical reasons, we used the smallest number of dogs as possible, where premolars and molars of the same dog were used, and a negative control group was not assigned in the study design.
Perforations sizes were standardized at 1.4mm [26], and bur penetration depth was limited to 2 mm to initiate the inflammatory reaction. In the present study, the access cavity was sealed with amalgam in all of the experimental teeth due to its known strength and good sealing ability, in addition to its common use in previous studies [13, 18]. For histological evaluation, the teeth were demineralized and processed by standard paraffin embedding procedures and then stained by Haematoxylin and Eosin for examination by microscopy [27, 28].
MTA has been extensively used as perforation repair material due to its reported favorable sealing ability, biocompatibility, and dentinogenic activity [29]. Biodentine is a bioactive [30] dentine substitute specifically designed as a “dentine replacement” material. In biodentine, tricalcium silicate and dicalcium silicate of the powder are the main and second core materials, whereby zirconium oxide is the radio-opacifier. The liquid has calcium chloride as an accelerator and a hydro-soluble polymer that serves as a water-reducing agent [31]. The material is characterized by the release of calcium when in a solution like MTA [32]. The experimental material used in this study is mainly calcium silicate and calcium phosphate -based. Due to the earlier proven good physio-mechanical properties [11] as well as biocompatibility and cytotoxicity of both constituents [12], this study aimed to investigate and evaluate the suitability of this material used in repairing perforations. It showed better results compared to other already available materials.
The results showed that no statistically significant difference was found between bone deposition scores in the three groups after 1 week, 1 month or 3 months; thus null hypothesis was accepted. This is probably due to the high similarity in the chemical composition between the materials [33]. All three groups showed a statistically significant difference between all three time periods. Within MTA, biodentine, and experimental material groups, bone deposition scores at 3 months evaluation period showed the statistically significant highest scores, and this is in accordance with other studies, which observed the very high biocompatibility of MTA in their examinations [34, 35]. The statistically significant lowest score found at 1 week and then 1 month evaluation periods can be attributed to the higher inflammatory reaction during this period [36-38]. Previous investigations on the experimental material found mineral deposits to be related to both MTA and ECSP. The source of calcification may be the calcium ions released by both materials, which can react with phosphate ions from tissue fluids, leading to the formation of an amorphous calcium-phosphate layer, which is a precursor to calcification and the formation of hard tissue barriers [12, 39].
The inflammatory reaction scores were not statistically different between the three evaluation periods for all materials. For all materials, a higher inflammatory response was found in the initial period (1 week) and decreased gradually in the subsequent periods, which is in accordance with other studies [40-42]. It was found that the inflammatory cells form and release different factors and interleukins, such as interleukin-1 (IL-1), IL- 6, tumor necrosis factor A, and prostaglandins (PGE2). These cytokines stimulate osteoclast formation and activity [38, 43]. Therefore, the intense inflammatory process seen at 1 week may be responsible for the increase in the osteoclastic activity seen at this evaluation period. Though it is impossible to examine the tissue reaction produced due to the traumatic injury taking place while performing the perforations, the high number of inflammatory cells observed at 1 week could be, at least in part, a defense mechanism to this injury. In a study conducted on dogs, Holland et al. [40] discovered that MTA induced different tissue reactions after 30 days, while after 180 days, the periodontium was nearly free of inflammation. This study allowed the assumption that we can obtain improved results on long-term evaluations. It is well recognized that calcium phosphate-containing materials induce inflammatory reactions (necrosis) in different tissues at early stages, but there has not been a final assessment regarding how long this situation continues and when the healing phase begins [14]. Further studies are required to assess the effect of the size of the perforation and delayed time of repair on the tissue response.
CONCLUSION
The experimental tricalcium phosphate cement performed similarly to the other materials showed promising results with evidence of healing by new bone deposition and few inflammatory cells at the perforation site when used as a perforation repair material. It can be considered as a successful treatment option for repairing furcation perforations at a lower cost.
ETHICS APPROVAL AND CONSENT TO PARTICIPATE
The research protocol was approved by the medical research ethical committee, National Research Center, Egypt (Registration number 17-077).
HUMAN AND ANIMAL RIGHTS
All the experimental procedures were carried out under international guidelines for the care and use of animals.
CONSENT FOR PUBLICATION
Not applicable.
AVAILABILITY OF DATA AND MATERIALS
Not applicable.
FUNDING
None.
CONFLICT OF INTEREST
The authors declare that there is no conflict of interest, financial or otherwise.
ACKNOWLEDGEMENTS
Declared none.