The Effect of Curing Pressure on Shear Bond Strength of Zirconia to Resin Cement
Abstract
Background:
Nowadays, the esthetics demand is continuously increasing; therefore, metal-free materials are widely used, like a zirconia-based ceramic, which is conveniently fabricated via computer-aided design and computer-aided manufacturing (CAD/CAM) system for restorations from single to full mouth rehabilitation.
Objective:
This study evaluated the effect of pre-curing pressure on the shear bond strength of zirconia to the resin cement.
Methods:
A total of sixty-three sandblasted cylindrical zirconia mounted in autopolymerizing resin were randomly assigned to three groups; Group 1: no treatment (control), Group 2: negative pressure, and Group 3: positive pressure to resin cement after resin cement application and resin composite columns bonded to zirconia. Thirty-three of the samples were stored in distilled water at 37 °C for 24 hr before the shear bond strength test for thirty samples and three samples were cross-sectionally cut for interfacial observation with FESEM. Another thirty samples were thermocycled for 5,000 cycles in distilled water at 5°C to 55 °C before testing. The shear bond strength and failure mode were evaluated. Examination of the bonding interface was also done.
Results:
The results were analyzed using two-way ANOVA. The means of shear bond strength of non-thermocycle of the control group were 8.01 ±1.74 MPa, 9.10 ±1.90 MPa, and 9.14 ±2.58 MPa, whereas that of thermocycle group were 5.71 ±0.84 MPa, 5.53 ±0.68 MPa, and 5.68 ±0.77 MPa in zero pressure group, negative pressure group, and positive pressure group, respectively. It showed no statistically significant differences in shear bond strength in all pressure groups (p > 0.05). The pre-curing pressure did not influence the shear bond strength of the zirconia and resin cement.
Conclusion:
There was no difference in the shear bond strength between the pressure groups and the no treatment control group. The positive and negative pressure did not influence the shear bond strength of the zirconia and resin cement.
1. INTRODUCTION
The esthetics demand is continuously increasing; therefore, metal-free materials are widely used, like a zirconia-based ceramic, which is conveniently fabricated via computer-aided design and computer-aided manufacturing (CAD/CAM) system to restorations [1]. The technical complication of full coverage restoration is the loss of retention [2, 3] which is caused by improper tooth preparation, cement selection, and cementation [3].
The retention form, resistance form of a prepared tooth, chemical bond of cement, how capable filler particle penetrate to the rough surface, all of these are the factors affecting the bonding of fixed prosthetic restoration [4]. Particle abrasion is used to clean various surfaces, increase bonding, and wettability with surface roughness. The 10-MDP has been applied to make the chemical bond between the zirconium oxide layer and resin cement [1, 5, 6]. However, decreasing durability of bonding has been reported [5], but using 10-MDP cooperating with mechanical pretreatment can improve the durability of bonding [6-9]. According to a systematic review of applying air-abrasion with alumina particle on zirconia surface followed by applying primer containing MDP or resin cement containing MDP can reach the maximum bond strength and durability and this method is recommended to do before bonding the zirconia [9].
The rough surface of zirconia results from both addition and subtraction of surface by acid-etching, airborne particle abrasion, laser, and selective infiltration-etching. Airborne particle abrasion is the convenient and common method, which can lead to various characteristics surface such as flaws, pits, microcrack, melting of the surface, embedded aluminum oxide, grain pull-out, and plastic deformation. Sharp-edged, deep pits easily trap air due to the penetration limit of cement, mainly when viscous cement is applied, but the results may be better if there were shallow, wide, open pits which facilitate cement spreading. The result cannot be determined and hard to know exactly what surface characteristics will be after air-abrasion [10].
The air pressure has been used to improve many dental procedures for many decades, especially reducing voids in the polymer material, interface, and facilitating polymerization. Studies reported that pressure regulating triple syringe could help adhesive agents to spread a thinner uniform film to entire substrate surface irregularities [11]. Another study showed that the sub pressure (negative pressure) or vacuum exhaust gas in the dentinal tubule facilitate adhesive agents to penetrate the entire dentinal tubule [12]. Li et al. [13] suggested that the sub pressure infiltration technique (negative pressure) can result in improvement in the adhesion between zirconia and resin by eliminating voids and promoting intimate contact between resin cement and zirconia. The physical method tends to promote zirconia bonding. The use of these pressure before curing resin cement for reducing voids have not been established; therefore, positive, negative, and zero pressure were prepared jointly in this study. The aim of this study was to evaluate the bonding of zirconia and resin cement in terms of shear bond strength values and bonding interface observation in the different pressure conditions. We formulated the null hypothesis that there was no significant difference between the various pressure treatments on shear bond strength of zirconia to resin cement.
2. MATERIALS AND METHODS
2.1. Specimen Preparation
Specimen division is shown in Table 1. Thirty-three of the samples were stored in distilled water of 37 °C for 24 hr before the shear bond strength test for thirty samples and three samples were cross-sectionally cut for interfacial observation with FESEM. Another thirty of the samples were thermocycled for 5,000 cycles at distilled water of 5°C to 55 °C before testing.
Study Groups | Subgroups | Subtotal |
---|---|---|
Non-thermocycled groups | Zero pressure | 11 |
Negative pressure | 11 | |
Positive pressure | 11 | |
Thermocycled groups | Zero pressure | 10 |
Negative pressure | 10 | |
Positive pressure | 10 | |
Total | 63 |
A total of 63 zirconia discs were made from pre-sintered zirconia blocks, a 4 mol% yttria-stabilized tetragonal zirconia polycrystal (4Y-TZP), (Ceramill Zolid HT+, Amann Girrbach AG, Koblash, Austria) with milling machine (250i, imes-icore GmbH, Eiterfeld, German) and cutting equipment (IsoMet 1000, Buehler Ltd., Lake Bluff, Illinois, USA). The discs had a diameter of 14 mm, thickness of 1.5 mm. After fully sintered, the final dimension was approximately of 12 mm diameter, 1 mm thick, and was mounted in PVC tube (26 mm diameter, 15 mm height) with autopolymerizing acrylic resin. Air-abrasion was done 10 mm away from a vertical distance with 50 µm aluminum oxide particles for 10 sec with 2.5 bar pressure, and then, all specimens were cleaned ultrasonically for 3 min in distilled water. Then, a piece of adhesive tape (140 µm thickness) was attached to it, then a hole of a diameter of 5 mm was made on the zirconia to define the bonding area and control cement volume. The surface roughness of every bonding area was measured with a profilometer (Profilometer, Surfcorder, SE2300, Kosaka Laboratory Ltd, Tokyo, Japan) before the bonding procedure, and the standard deviation was less than 10% of the mean of average roughness.
The resin composite (Filtex Z350XT A3.0, 3M ESPE, USA) column was made with a stainless steel mold of 4 mm diameter and 6 mm height; then the mold was covered with transparent glass follow by light-curing (Bluephase® Style, Ivoclar Vivadent, Liechtenstein) High Power mode, the intensity of 1,100 mW/cm2 for 15 seconds at both sides. The bonding areas were polished with silicon carbide paper of 600 grit and cleaned ultrasonically.
2.2. Bonding
All the samples were assigned to three groups randomly; group 1: no treatment (control), 2: negative pressure (-0.08 MPa), and 3: positive pressure (+0.3 MPa). The methodology is followed from a study done by Li et al. [13]. The cement pastes A and B (PANAVIA F 2.0 Paste) were harmoniously mixed and applied to zirconia at the hole of adhesive tape, and the cement beyond the edge of the adhesive tape was wiped away for controlling cement volume. The pressure group zirconia specimens (group 2 and group 3) were brought to the center of the lightless pressure pot then the pot was covered with a lid to make a perfect seal. In the positive pressure group, the air was added to the pot until a positive pressure gauge displayed 0.3 MPa and in the negative pressure group, the air was pumped out of the pressure pot by vacuum pump until a negative gauge displayed -0.08 MPa then it had been continuously maintained for 1 minute before pot pressure was released (Fig. 1).
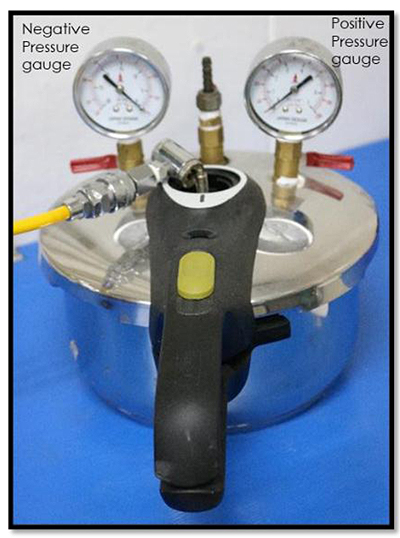
While primer A and primer B were mixed and then applied to the resin composite column according to the manufacturers’ instructions. The zirconia from the pot and the resin composite column were bonded together, then the weight of 1 kg was applied to the specimen, and excessive cement was removed with a disposable micro brush before light curing of 4 directions, each direction for 20 s. Control group specimens were bonded without an extra step in the pressure pot. Half of the samples in each group were tested for shear bond strength following storage in distilled water of 37 °C for 24 hr, and another half of the samples in each group were thermocycled for 5,000 cycles at distilled water of 5°C to 55 °C before testing. The dwell time in each water chamber and traveling time between the two chambers were 30 sec and 15 sec, respectively. Then the bond strength (shear) testing was performed.
2.3. Shear Bond Strength and Failure Mode Testing
Bond strength (shear) was performed with the universal testing machine (Lloyd Instruments, LRX-Plus, AMETEK Lloyd Instrument Ltd., Hampshire, UK). Each specimen was mounted in a shear test jig that custom-made from stainless steel and a blade was intimately positioned to the specimen, as shown in Fig. (2). Only a polyester film strip (0.125 mm) could pass the gap between them. The specimen was loaded at 0.5 mm/min crosshead speed until it broke the bond. The shear bond strength of specimens was calculated as the load at failure/the area of the bonding surface.
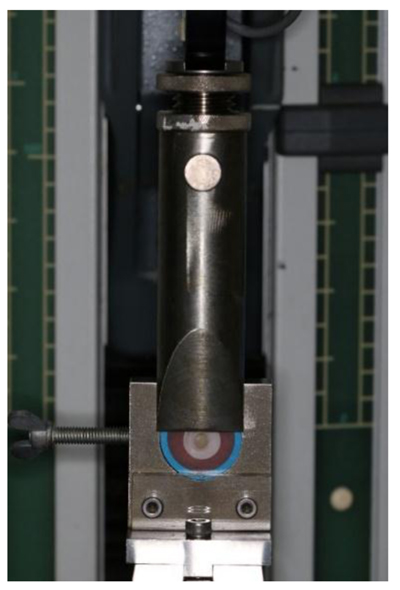
The failure mode of specimens was observed with a measuring microscope (MM-400, Nikon Corporation, Tokyo, Japan) for 50 magnification and categorized into three types as the following [14].
1. Cohesive failure within resin cement, resin composite, or zirconia
2. Adhesive failure at the interface between zirconia and resin cement when >75% of resin cement remained on resin composite or failure at resin cement-resin composite interface when >75% of resin cement remained on zirconia
3. Mixed: If less than 75% of resin cement remained on zirconia or resin composite and with or without cohesive failure. The failure was considered mixed.
2.4. Examination of Bonding Interface
One representative specimen was selected randomly in each group and embedded in autopolymerizing resin and cut perpendicular to the zirconia-resin composite interface with the cutting equipment (IsoMet 1000, Buehler Ltd., Lake Bluff, Illinois, USA). The bonding interface in each group was examined by Field Emission Scanning Electron Microscope (FESEM; Apreo, Thermo Fisher Scientific (Thailand) Co., LTD, Bangkok, Thailand).
2.5. Statistical Analysis
Results were analyzed using statistical software (SPSS version 20). Following the descriptive statistics, the differences in shear bond strength were calculated with Two-way ANOVA that shear bond strength as a dependent variable, thermocycled conditions and pressure conditions as independent factors, followed by post-hoc Tukey’s HSD. A value of p < 0.05 was considered statistical significance.
3. RESULTS
The average surface roughness of all the fully sintered zirconia was 0.45 µm. The mean shear bond strength tests and standard deviation of different pressure groups are shown in Table 2. The results of the shear bond strength are shown in Table 3. Two-way ANOVA indicated the pressure condition (p=0.50) and the interaction between two factors (p=0.38) had no statistically significant influence on the shear bond strength and a statistically significant difference was detected among non-thermocycled group and thermocycled group (p<0.05). The means of shear bond strength was higher in non-thermocycled group compared to thermocycled group.
Subgroups | Non-thermocycled (mean ±SD) | After 5,000x thermocycled (mean ±SD) |
---|---|---|
Zero pressure | 8.01 ±1.74 | 5.71 ±0.84 |
Negative pressure | 9.10 ±1.90 | 5.53 ±0.68 |
Positive pressure | 9.14 ±2.58 | 5.68 ±0.77 |
Source | SS | df | MS | F | p-value |
---|---|---|---|---|---|
Thermocycle | 145.299 | 1 | 145.299 | 57.839 | 0.000 |
Pressure | 3.529 | 2 | 1.764 | 0.702 | 0.500 |
Pressure x Thermocycle | 4.952 | 2 | 2.476 | 0.986 | 0.380 |
Residual | 3241.305 | 55 | 3108.162 | ||
Total | 3395.085 | 60 |
A summary of modes of failure of specimens in study groups is presented in Table 4. Microscope observation of the bonding area predominantly showed mixed failure in non-thermocycled and few cohesive failures within resin cement. There was a greater number of mixed modes of failure and a few interfacial failures between the resin cement-zirconia interface in thermocycled group.
Study groups | Subgroups | Cohesive failure | Interfacial failure | Mixed mode of failure |
---|---|---|---|---|
Non-thermocycled groups | Zero pressure | 100% (11) | ||
Negative pressure | 19% (2) | 81% (9) | ||
Positive pressure | 19% (2) | 81% (9) | ||
Thermocycled groups | Zero pressure | 20% (2) | 80% (8) | |
Negative pressure | 40% (4) | 60% (6) | ||
Positive pressure | 60% (6) | 40% (4) |
Fig. (3) shows the FESEM images of the bonding interface in the control group, negative group, and positive group. Three cross-section microscopy images showed two interfaces: resin composite-resin cement interface and resin cement-zirconia interface. The representative images showed a similar structure as the resin composite firmly adhered to the resin cement layer; neither gaps nor voids appeared at this interface. The zirconia bonding surfaces were treated with 50 µm aluminum oxide particles resulting in a little wave and some voids appeared at this interface. The middle layer was the resin cement layer with various sizes of porosity spreading all over the layer.
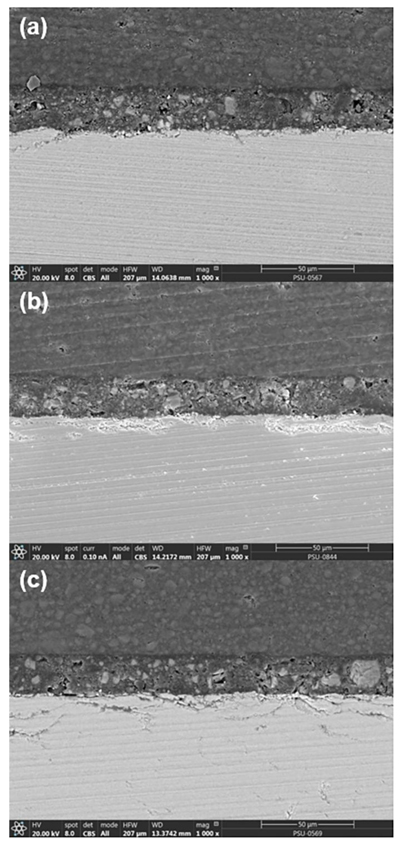
4. DISCUSSION
The bond strength and failure modes of various materials in dentistry can be measured using the shear bond test [15, 16]. In this study, the shear test measured the resin cement with MDP’s bond strength to zirconia in different conditions and the mean bond strength ranging from 8.01-9.14 MPa, which were lower than reported by a systematic review and meta-analysis (15 MPa) [15]. The different values of each study possibly occurred due to different testing conditions. Additionally, the different substrates of each experiment have been used to bond to zirconia surfaces, such as zirconia bond to each other, which has superior cohesive strength compared to other interfaces; therefore cohesive failure in zirconia is eliminated [17].
Zirconia bonded to dentin which can imitate clinical situations, but dentin microstructure has a great variation [18]. Zirconia bonded to cement, which has only one interface but cohesive failure in cement occurs easily due to cement weakness and zirconia bonded to composite block has weak bond at the interface and the zirconia/resin cement interface. Even though there were two different interfaces, but adhesive failure between the resin cement and composite block rarely took place in this study, and cross-section under SEM images demonstrated firm attachment and no gap was observed between them. A mixed-mode of failure was predominant in this study because there was a cohesive failure within resin cement combined with adhesive failure between the resin cement-zirconia interface. It was similar to the results obtained by the previous study [19, 20]. After the thermocycling, there was a significant reduction in the bond strength and an increase in the adhesive failure in all subgroups. There were no significant differences in bond strength among thermocycled groups, and this implies that the zirconia-resin cement bond strength can be weakened during the thermocycling process with time. An explanation can be that the hydrophilic resin at that interface can absorb water, which leads to hydrolysis. Hence, water-induced degradation is an influence on decreasing the bond durability and another reason is the different coefficient of thermal expansion of zirconia and resin cement, which is twofold higher for resin cement. Thus, thermocycling directly stresses the adhesive joint [21]. The 5,000 thermal cycles in this study would correspond to 6 months due to a suggestion of 10,000 cycles representing a service year [22].
The roughness of the substrate is one of the key factors to have a successful bonding. Hydrofluoric etching glass-ceramic surface is usually done for a rough surface. The glass-free zirconia surface can be etched in only appropriate conditions, such as 9.5% hydrofluoric acid (HF) at 25°C for 1 hr or 80°C for 1 min to produce a nano irregular pattern of zirconia surface. However, nano roughness is not enough for good bonding, and the high viscous cement cannot completely infiltrate into the nano pits of the etched-zirconia surface [23]. So air abrasion plays an important role in the zirconia bond despite the tetragonal-to-monoclinic phase transformation. And if the monoclinic concentration is between 12% - 54%, the flexural strength tends to decrease, but that can cope with the masticatory force [24]. In this study, negative and positive pressure groups required an increase of 60 seconds in working time, it was practicable due to the 3 min working time of Panavia F2.0, and cement paste would start to set when contacted with ED PRIMER II, which was applied on resin composite blocks.
Many studies reported polymerizing under positive pressure can reduce the flaws, especially voids within the material and improves its mechanical performance, such as increasing the degree of conversion, polymerization rate, flexural strength, and hardness [25-28], but some studies denied it [29, 30]. The use of positive pressure applying before cement polymerization for reducing flaws has not been established, but the SEM images and the bond strength found no differences between groups. Formation of voids in polymeric materials can occur due to air entrapment during mixing and monomer vaporization both prior to and during polymerization [26]. Generally, the pressure limit of the pressure pot must not exceed 0.4 MPa hence this study chose a pressure level as much as possible. Frangulyan et al. [31] studied the processes of phase transformations in zirconium ceramics under the action of mechanical treatment and thermal annealing. Hence, it is recommended that after air abrasion, the samples would have to be annealed to remove the induced monoclinic phase.
The sub pressure infiltration technique explains voids elimination by the gas that traps between the adhesive agent, and the substrate can be exhausted when sub pressure is applied due to the pressure gradient, when releasing the sub pressure, the atmosphere automatically instantly presses the adhesive agent replacing those voids. Interfacial voids are eliminated, and the adhesive agent can infiltrate into all the micro-pits that cause the adhesive agent to intimately contact the substrate as a consequence of the sub pressure infiltration technique [12]. On the contrary, our SEM images demonstrated voids within the resin cement layer, and some gaps between resin cement-zirconia and the bond strength were not different from other groups. A possible explanation for this could be that void elimination may be possible during the resin cement is being mixed without trapping the air because of an airless environment and only the surface bubbles that can be sucked out. But if mixing has already been done, only bubbles that move upward to the surface can be expelled, which is a small number of bubbles. According to Archimedes' principle and Newton's laws of motion, the bubble’s Buoyant force must more than the bubble’s weight combine with cement viscous force so the bubbles can move upward, or the bubbles can easily move up if specimens or the pressure pot were places on a laboratory vibrator. Therefore, many bubbles remain in resin cement and interface as the SEM images. In this study, Specimens were applied 0.08 MPa negative pressure for 1 min, double negative pressure but less time comparing to the previous study. The converse results might be due to different testing conditions.
Many dental offices certainly have their air pressure system, and some offices might have a vacuum mixing system for gypsum that be able to supply and vacuum pressure to the pot, so the clinical application would be possible. The only extra equipment will be the pressure pots that are available for sale. And the most important one is having enough working time for the resin cement.
CONCLUSION
In this study, there was no difference in the shear bond strength between the pressure groups and the control group (no treatment). Application of both positive and negative pressure to the resin cement before curing does not influence the bond of zirconia and resin cement.
ETHICS APPROVAL AND CONSENT TO PARTICIPATE
Not applicable
HUMAN AND ANIMAL RIGHTS
Not applicable.
CONSENT FOR PUBLICATION
Not applicable.
AVAILIBILITY OF DATA AND MATERIALS
Not applicable.
FUNDING
This research was supported by the Maxillofacial Prosthodontics and Dental Materials Research Unit, Faculty of Dentistry, Prince of Songkla University, Songkhla, Thailand.
CONFLICT OF INTEREST
The authors declare no conflict of interest, financial or otherwise.
ACKNOWLEDGEMENTS
Declared none.