All published articles of this journal are available on ScienceDirect.
The Comparison of Biofilm Formation, Mechanical and Chemical Properties between Glass Ionomer Cement and Giomer
Abstract
Aims:
The aim of this study was to compare compressive strength and its correlation with the surface morphology and chemical elements of GIC and Giomer, as well as to determine the fluoride amount effect on the bacterial biofilm formation of GIC and Giomer.
Background:
The liability of Glass Ionomer Cement (GIC) mechanical properties is overcome with better antibacterial properties among restorative materials. Another fluoride-releasing restorative material, such as Giomer, has been discovered and is expected to overcome the issues with GIC’s mechanical properties; however, no research has been conducted related to antibacterial properties in Giomer.
Objective:
To compare compressive strength and its correlation with the surface morphology and chemical elements, then determine the fluoride amount effect on the bacterial biofilm formation of GIC and Giomer.
Methods:
Sixteen specimens of GIC and Giomer were prepared for a compressive strength measurement with the Universal Testing Machine. Sixteen specimens of GIC and Giomer were incubated for three days with the Streptococcus mutans culture at 37°C. The bacterial colonization was calculated using the Colony Forming Unit (CFU) and bacterial adhesion was calculated using a Scanning Electron Microscope (SEM). The mechanical properties’ compressive strength measurement, surface morphology, and chemical elements analyses were performed using SEM-EDX.
Results:
The compressive strength of Giomer was higher than GIC (P=0.001). The higher compressive strength of Giomer was reflected by a predominant regular surface, fewer voids, smaller and denser particles, and a higher content of silica and carbon. The bacterial biofilm on the surface of Giomer was higher than GIC, although there was no significant difference. GIC and Giomer have identical chemical elements: C, O, F, Na, Al, Si, P, and Ca.
Conclusion:
The compressive strength of Giomer is better than GIC; however, the biofilm formation of Giomer is higher than GIC, whereas GIC has a higher fluoride content but inferior in surfaces morphology characteristic
1. INTRODUCTION
Glass Ionomer Cement (GIC) is a water-based dentistry material that is commonly used due to its advantages. It can bond with dentin and enamel chemically through ion exchange mechanisms, is biocompatible, and provides a long-term fluoride release [1, 2]. The weaknesses of GIC are its translucency, hardness, and strength, which make it vulnerable to fractures and less aesthetic [2, 3].
The development of dental cement continues to provide cement with excellent compatibility, remineralization, and good mechanical and physical properties. Several attempts in its development include the addition of tartaric acid to GIC to improve its mechanical properties [4] and the addition of carbon nanotubes to GIC to increase its mechanical strength and bioadaptation [5].
One of the most important biological properties considered in determining the use of dental materials is the antibacterial properties. Materials that have antibacterial properties can better prevent the development of biofilms. Antibacterial activity is also related to the release of fluoride ions or Ag, Cu, and Zn [6]. Fluoride on teeth reduces demineralization and increases remineralization. Fluoride ions also play a role in inhibiting pellicle and plaque formation and preventing microbial growth [7]. Among the various types of restorative materials on the market, GIC has the best antibacterial properties due to the ability to release fluoride. Other strengths of GIC are excellent adhesive properties and good thermal compatibility with tooth enamel [8]; however, GIC’s disadvantages include imperfect aesthetics and low tensile and compressive strength, so it is not recommended to be applied to posterior teeth [9, 10].
As a technological development, Shofu Inc. (Kyoto, Japan) introduced a new hybrid aesthetic restorative material called Giomer. Giomer is an adhesive material in dentistry made from resin that contains a Pre-Reacted Glass-ionomer (PRG) filler. The advantages of Giomer include the release and recharge of fluoride, resistance to acid, anti-plaque effect, remineralization of dentin, and acid buffering capacity [11]. Due to these advantages, Giomer is expected to be an alternative adhesive material in dentistry.
Both GIC and Giomer have a fluoride release ability as an anti-cariogenic agent. Giomer is expected to overcome GIC’s disadvantage in its mechanical properties. In this study, the mechanical property tested was compressive strength because the restoration material is subject to pressure generated by the mastication system [12]. The forces on the restoration material in the mastication process are a combination of compression, tension, and flexures, which are dominated by compression [12, 13].
A previous study on GIC added with Calcium Deficient Hydroxyapatite (nCDHA) nanocrystalline showed a correlation between the hardness value and microstructure of GIC. The denser the surface texture, less and smaller air cavity, the higher hardness of GIC [14]. Similar research on GIC given a milling treatment has shown that GIC has the longest milling time (80 minutes) and the highest compressive strength value with the least and smallest voids [15] Another study on fissure sealant types of GIC showed a significant increase in surface roughness after prophylactic treatment accompanied by a decrease in compressive strength [16]; however, the correlation between the surface morphology and compressive strength of GIC and Giomer remains unknown.
In addition to surface morphology, chemical elements may also affect mechanical properties. In human teeth, the decrease in the mechanical properties of hardness and the modulus of elasticity in dentin with caries positively correlates with the reduction in mineral content (r2 = 0.93-0.92) [17]. The hardness and elastic modulus of enamel showed a positive relationship with the calcium content [18]. In this correlation, the restoration material showed that there was a significant relationship between the decrease in silica content and the reduction of surface hardness in GIC that was stored for one year in water [19]; however, there is currently no research on the correlation between a restoration material’s chemical elements and compressive strength. Therefore, in this study, compressive strength was compared between GIC and Giomer. A correlation between surface morphology and chemical elements using a Scanning Electron Microscopy and Energy Dispersive X-Ray (SEM-EDX) was observed.
2. METHODS
In this study, 32 specimens of GIC (GC Fuji IX®, Tokyo, Japan) and 32 specimens of Giomer (Beautifil II SHOFU Inc., Kyoto, Japan) were prepared for compressive strength and biofilm formation. Data were analyzed by an Independent T-test. Another specimen of GIC and Giomer was prepared for a surface morphology and chemical elements analysis using SEM-EDX (Fig. 1).
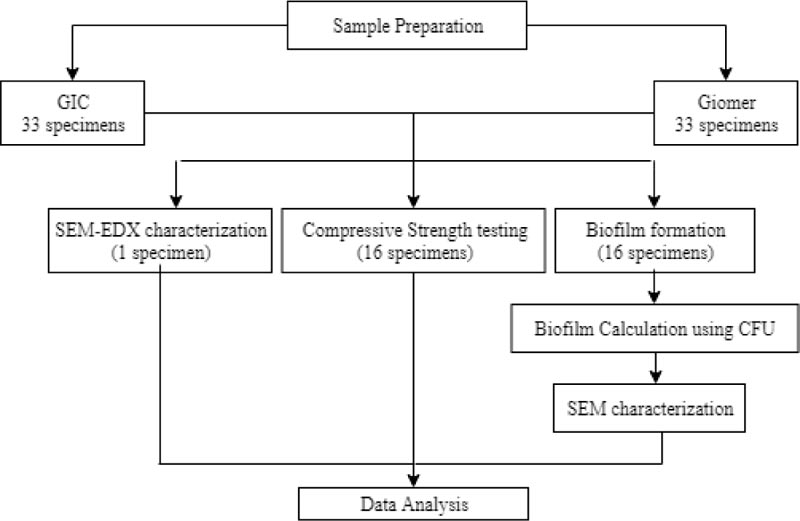
2.1. GIC Specimen Preparation
The powder and liquid of the materials were mixed according to the manufacturer’s instructions. A spoon of powder and a drop of liquid were manually mixed with a plastic spatula using a folding motion on the mixing pad and mixing slab for a homogeneous consistency.
For the compressive strength measurement, 16 specimens of GIC were shaped into a cylindrical diameter of 4 mm and a thickness of 6 mm. The surface was flattened by pressing it using a mylar strip and a microscope glass slide. After the GIC set, 16 specimens were coated with varnish and stored in an incubator at 37°C for 24 hours [20].
For the measurement of biofilm formation, 16 specimens of GIC were prepared with a diameter of 4 mm and a thickness of 6 mm. Specimens were placed for 24 hours at 37°C with 100% humidity before sterilization. All specimens were then sterilized at 55°C [20]
2.2. Giomer Specimen Preparation
To prepare 16 specimens for the compressive strength measurement, Giomer was applied to a cylindrical acrylic mold with a diameter of 4 mm and a thickness of 6 mm. The specimens were incrementally light-cured using an LED unit with an intensity of light 900 mW / cm2 for 10 seconds on each layer. After the final increment, the surface was flattened by pressing it using a mylar strip and a microscope glass slide. After polymerization, the specimens were taken out from the mold and stored in an incubator at 37°C for 24 hours [20].
For the measurement of biofilm formation, 16 specimens of Giomer were prepared with a diameter of 4 mm and a thickness of 6 mm. The specimens were placed for 24 hours at 37°C with 100% humidity before sterilization. All specimens were then sterilized at 55°C [20]
2.3. Compressive Strength Testing Procedure
The compressive strength of specimens was tested using Universal Testing Machine (Shimadzu, AGS 5 KnX). Before the test, the specimens’ diameter and thickness were measured using a digital caliper, and they were then placed into a machine plate at an angle of 900 to the specimen’s surface. The specimens were subjected to a maximum force of 5 kN with a crosshead speed of 1 mm/minute until the specimens fractured. The monitor showed the load (Newton) and the compressive strength value (MPa). Compressive strength was calculated in megapascals using the formula CS = P / πr2, where CS is compressive strength (MPa), P is the load (Newton), and r is the radius of the specimen (millimeter) [21].
2.4. Biofilm Formation
Colonies of S. mutans bacteria (ATCC UA159 strain) were cultured in a 3 mL BHI broth and left overnight in an incubator at 37°C and 5% CO2. A serial dilution or a tenfold dilution of the bacteria was performed for the inoculation medium. The specimens were placed in 24-well plates, inoculated with 1mL of inoculation medium, and then incubated at 37°C and 5% CO2 for 3 days [20].
2.5. Biofilm Calculation Using CFU
The GIC and Giomer specimens that had been affixed by a biofilm were cleaned using sterile PBS and then transferred to eppendorf tubes with 1mL BHI broth. The biofilm was then taken using a vortex mixer for 5 minutes to be transferred and dispersed from the surface of the specimen to the BHI broth. A dilution was produced for the bacterial suspension, and then it was placed on BHI agar plates. The plates were then incubated at 5% CO2 and 37°C for 3 days. After incubation for 3 days, the number of colonies was calculated in line with the dilution factor to calculate the total CFU of each specimen [22].
2.6. SEM-EDX Characterization Procedure
Each specimen with biofilm was cleaned with 1x sterile PBS and dipped in 2.5% glutaraldehyde in PBS 2x each for 10 minutes. It was then dissolved using 1% osmium tetroxide for 1 hour and cleaned with PBS for 10 minutes 2x and cleaned again with distilled water. Each specimen was dried with a dryer.
Before all the specimens were scanned using SEM-EDX (EDAX®, AMETEK®, Berwyn, PA, USA), they were sputter-coated with gold (Au) to avoid charging, which can cause image distortion. The specimens were then placed on the specimen stage, followed by the vacuuming process to remove air in the tube [23]. Next, the specimen was scanned with a beam of electrons emitted by an electron gun with a SEM-EDX device. The characterization of specimens by SEM-EDX was performed at 1000x, 2000x, 3000x, and 5000x magnifications. The data were then obtained in the form of images, graphs, and percentages of chemical elements.
2.7. Statistical Analysis
The data of compressive strength were analyzed statistically using computer software SPSS [20]. The test statistic used was the Independent T-test for the significance value. Next, the surface morphology images and the chemical elements of the specimens from the SEM-EDX analysis were observed, specifically the correlation to the compressive strength values and biofilm colonization on GIC and Giomer surfaces.
3. RESULTS
The results of the compressive strength test are shown in Table 1. It shows that GIC has a lower compressive strength than Giomer. Based on the data, the Independent T-test was performed, and the results in Table 1 show that the compressive strength value between GIC and Giomer is significantly statistically different (p <0.05).
Variable | Mean (SD) MPa | P Value |
---|---|---|
Glass Ionomer Cement (n = 16) | 118.5869 (20.13164) | 0.001 |
Giomer (n = 16) | 204.6675 (30.28604) |
The results of the bacteria that formed in GIC and Giomer are shown in Table 2. It shows that GIC has a lower bacteria formation than Giomer. Based on the data, the Independent T-test was performed, and the results in Table 2 show that the number of bacteria that formed between GIC and Giomer is insignificantly statistically different (p >0.05).
Variable | Mean (SD) | P Value |
---|---|---|
SIK (n = 16) | 3270.75 (690.007) | 0.893* |
Giomer (n = 16) | 3286.88 (808.492) |
Surface morphology was analyzed using SEM with four different magnifications, as shown in Fig. (2). The figure shows that GIC has a more irregular external surface than Giomer. Giomer’s surface has smaller particle sizes with a denser arrangement compared to the GIC’s surface. In addition, GIC’s surface has more voids than the surface of Giomer. For both materials, cracking was not found.
The observations of biofilm images on GIC and Giomer surfaces shown in Fig. (3) were carried out using a Scanning Electron Microscope (SEM) tool with a magnification of 2500x. The biofilm images on GIC are shown with a red circle. The biofilm colonization is thin and round. The picture of the biofilm shown by the green circle on Giomer resembles elongated spheres in the form of chains. The biofilms in the image are tight and dense.
The results of the EDX analysis showed that a spectrum was obtained from energy due to interactions between electrons and specimens. The spectrum of the EDX test results is shown in Fig. (4). Based on the energy emission, the chemical elements of the material can be seen in Table 3. Based on these data, there are no variations in the chemical elements between the two materials. Basically, GIC and Giomer have carbon (C), oxygen (O), fluorine (F), sodium (Na), aluminum (Al), silica (Si), phosphorus (P), and calcium (Ca) properties.
Element | GIC | Giomer | ||
---|---|---|---|---|
wt% | at.% | wt% | at.% | |
C | 19.15 | 29.16 | 24.18 | 36.23 |
O. | 28.70 | 32.80 | 27.47 | 30.90 |
F | 09.95 | 09.58 | 04.15 | 03.93 |
Na | 05.16 | 04.10 | 02.12 | 01.66 |
Al | 17.53 | 11.88 | 16.33 | 10,89 |
Si | 17.20 | 11,20 | 25.09 | 16.07 |
P. | 01.68 | 00.99 | 00,16 | 00.09 |
Ca | 00.61 | 00.28 | 00.50 | 00.22 |
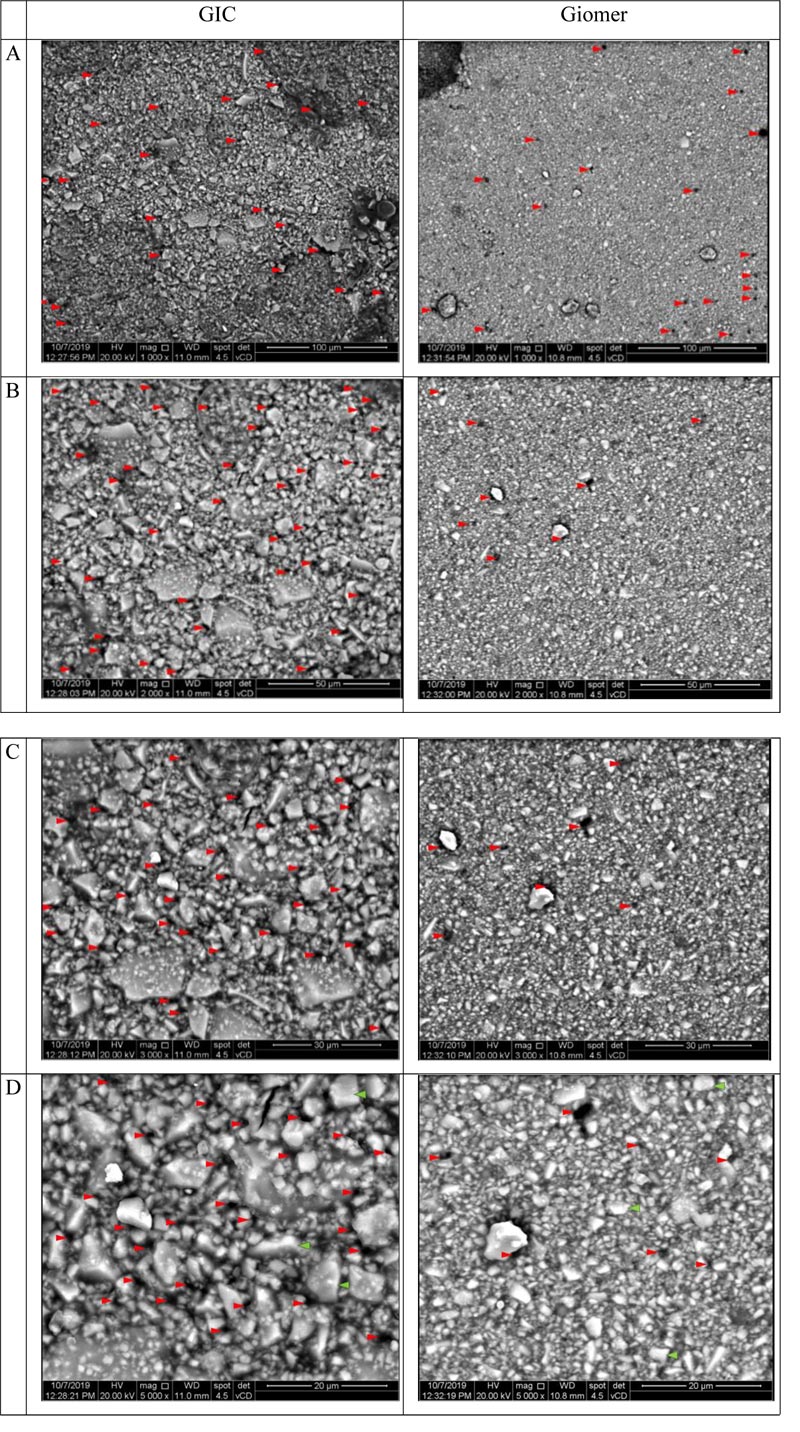
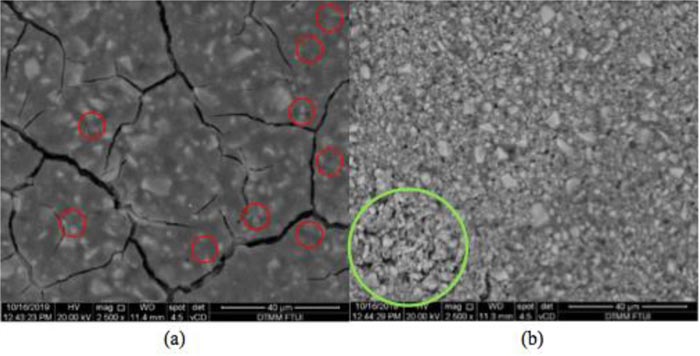
Table 3 shows that the silica content in Giomer is much higher at 25.09% compared to GIC at 17.20%. The calcium content in GIC is higher at 0.61% compared to Giomer at 0.5%. The phosphorus content in GIC is also higher at 1.68% compared to Giomer at 0.16%, along with a higher fluorine content in GIC at 9.95% compared to Giomer at 4.15%.
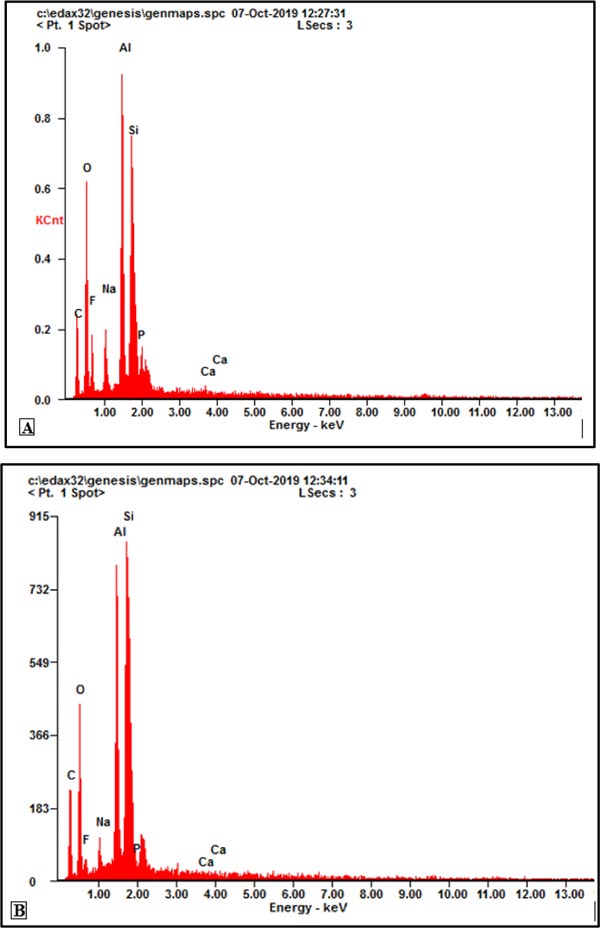
4. DISCUSSION
In this study, the mean value of GIC compressive strength was found to be 118.59 ± 20.13 MPa. This value is slightly different from the control specimens in the study by Sufyan Garoushi et al. (98.0 ± 12.0) [24]. This may be due to the differences in specimen preparation, which included the limitation of a mixing time of less than one minute and storing the specimens in wet conditions before the compressive strength test was performed. The mean value of Giomer’s compressive strength in this study is 162.34 ± 30.29 MPa. This value is slightly lower than the value found by SM Abdul Quader, which is 271.36 ± 19.65 [21]. This may be due to the different curing units and techniques used, i.e., using visible light units (Selector, Taiwan) with several irradiation times, 40 seconds on the specimen’s upper and lower surfaces, and 40 seconds on each specimen’s side surface. The superiority of Giomer’s strength may be due to the cross-linked polymer matrices. Therefore, it seems to have a higher compressive strength and hardness value than the gel network formed by acid-base reactions in GIC [21, 25, 26]. The unstable properties of GIC during the initial stage of setting when exposed to air also degraded the mechanical properties of the GIC [25].
The coupling agent plays an important role in improving the mechanical properties of polymer matrices in Giomer and facilitating pressure transfer [27]. Nevertheless, in GIC, the compressive strength was affected by ionic cross-links. This was proven in research by Liu J. et al., who added Al2O3-SiO2 particles and increased the liquids’ acidity. This condition improves the mechanical properties due to the release of more Al3+ ions, a source of ionic cross-linking [28]. The same will occur in a limited liquid condition, which made it difficult to form ionic cross-linking, resulting in decreased mechanical strength [28]. Another aspect that may affect the compressive strength is the duration of storage. The water component will evaporate in GIC that has been stored too long. Lack of water causes an increase in liquid viscosity and a decrease in compressive strength over time [28, 29]. The duration of storage of Giomer affects the photoinitiator, which will degrade over time and make the polymerization less optimal [29]. Moreover, incremental techniques in preparing Giomer may increase the possibility of voids and internal failure of specimens, which can affect the compressive strength [26]. It should be noted that the mechanical characteristics of an unstable GIC due to water loss and uptake in the initial stages of the setting reaction may remove ions and degrade the mechanical properties. Therefore, it is important to test GIC in a clinical condition simulation [25, 30].
The qualitative analysis based on surface morphology shown in Fig. (2) indicates that GIC presented a more irregular surface than Giomer. Furthermore, the surface of GIC has more voids than Giomer. This could be caused by the incorporation of air bubbles while mixing the powder and liquid manually [31, 32]. Giomer’s surface also has smaller particle sizes with a denser arrangement than that of the GIC’s surface. According to this finding, Giomer has a more regular external surface, fewer voids, and smaller filler particle size hence has a higher compressive strength value. Edoardo Pacifici et al. stated that different particle sizes influence material properties, such as fracture toughness, compressive strength, abrasion resistance, and surface microhardness [31]. In other studies, factors such as the integrity of the interface between the glass particles and the polymer matrix, particle size, and number and size of voids have been shown to play an important role in determining mechanical properties [32, 33]. Nevertheless, voids in GIC play an important role in releasing fluorine ions because this occurs through three mechanisms, one of which is diffusion through voids and cracks.
In a resin-based material, the release of fluorine ions occurs slowly due to the lower number of voids on the material [34, 35]. The modification of filler particle size and shape may affect the mechanical properties of GIC. Generally, a smaller and denser filler particle size increases the compressive strength and hardness of GIC, while larger particles can lead to higher wear resistance [36]. According to research by Shariq Najeeb et al. on nano-modified glass ionomers, GIC with a smaller filler particle size shows a smoother and more polished surface [32,36]; however, the effect of nano-sized filler particles on mechanical properties requires further investigation because in the study by Wen Lien and Kraig S., who compared the compressive strengths between several different types of composites, showed that Hybrid, Filtek Z250 with a large spherical-shaped of filler particles (~ 3.5 μm) has the highest strength properties. Similar to a nanocomposite, supreme filtek has a high compressive strength as well; however, Filtek LS with multimodal filler particles (0.04-1.7 µm) showed the lowest compressive strength [24]. Filler particles with smaller sizes show a smoother surface and a higher compressive strength. It is not the only size that matters but also the type, shape, number, and coupling agent used [36, 37].
The quantitative analysis based on chemical elements using an EDX analysis showed no variations in the chemical elements between the two materials; however, in Table 3, the silica (Si) and carbon (C) in Giomer are shown to be higher at 25.09% and 24.18% compared to GIC at 17.20% and 19.15%, respectively. Based on the compressive strength values, materials with a higher compressive strength have higher silica and carbon. Previous studies by Nayef H. Felemban and MI. Ebrahim, who added silica particles to RMGIC Resin Modified Glass Ionomer Cement (RMGIC), and Ali N. Alobiedy et al., who added carbon particles to GIC, both showed an increase in compressive strength values [38, 39]. Silica (Si) is the main component of the glass core and forms the glass network of GIC. Therefore, the small amount of silica causes the weakening of the glass network. Maho Shiozawa et al. found that instead of fluoride, the decrease in silica is considered more critical in deteriorating the mechanical properties of GIC19; however, Griffin and Hill, who conducted studies on the nature of GIC with an alumina-silica ratio, stated that the alumina-silica ratio on the glass did not have a clear influence on compressive strength [23]. In this study, the silica content was conformable with the compressive strength values of GIC and Giomer.
Restorative materials with good antibacterial properties can help prevent secondary caries [40]. In this study, the researchers used GIC and Giomer specimens. GIC is a restorative material commonly used in dentistry. This material contains calcium and strontium aluminosilicate powder combined with a water-soluble polymer, which, when mixed, will occur in a setting reaction involving the neutralization of acid groups by glass powder [9]. Giomer is a restorative material whose basic material consists of a matrix resin filler and polyalkenes acid. Giomer undergoes a polymerization reaction that provides strength followed by acid-base reactions due to the hydration of polyalkenes acid by water absorption after being placed in the cavity [40].
In this study, the amount of mature biofilm that formed in GIC and Giomer, which were allowed to stand for three days with the bacterial culture Streptococcus mutans, was examined. An in vitro study showed that biofilm maturation occurred after [24-72] hours depending on the species and environmental conditions; however, it was assumed that this maturation process occurred for 72 hours, although the host’s diet immunity also influenced the maturation process [41]. In another study on the formation of biofilms by Staphylococcus aureus, it was stated that bacterial biofilm maturation occurred, and 10 hours later, there was an increase in population density [42]. Biofilm can form on all surfaces exposed to the natural environment of the oral cavity [43]. Biofilm can develop on the surfaces of teeth, mucosa, and restorative materials [43].
The formation of biofilms on composite resin damages the material and surface and causes bacteria to damage the surface of the teeth between the restoration and teeth, thus causing secondary caries [41]. In this study, biofilms that formed on the surface of mature GIC and Giomer and developed after three days were left in an incubator at 37°C. This temperature is considered the optimal temperature in the growth and development of biofilms because it is similar to the oral cavity temperature condition [20]. In the previous studies, tests were carried out on the effect of temperature on bacterial growth, and significant differences in the development of bacteria allowed to settle at 28°C, 37°C, and 45°C were found [44]. The observation of the number of biofilms was carried out using a bacterial viability calculator called the Colony Forming Unit with a CFU/mL unit that previously did not need to be treated for bacterial dye because there was a lighting adjustment on the CFU. The contents of different agar plates also affect the lighting used [45]. After the observations using the Colony Forming Unit, the results showed that live bacteria present on the surface of Giomer were higher than those found on the surface of GIC, although there was no statistically significant difference. In previous studies evaluating the antibacterial ability of GIC, Giomer, and Composite Resin, it was found that there were significant differences between the bacteria on GIC and Giomer [46].
In other studies regarding the luminescence intensity of a S. mutans suspension, it was found that the luminescence intensity in Apatite Ionomer Cement (AIC) and GIC is significantly more effective in reducing the intensity of S. mutans compared with Giomer. A study on the zone of inhibition of bacterial development in restoration materials showed that the zone of GIC inhibition was best compared to other restorative materials, namely Giomer and composite resin [8]. GIC can prevent the development of several species of oral cavity bacteria. Giomer is in the second position in preventing Streptococcus mutans after GIC. Giomer can release 20% of fluorine from GIC [47].
Based on the observations using SEM-EDX, there are various similarities in ion types found in GIC and Giomer, including Ca, O, F, Na, Al, Si, P, and C, with different percentages of ions in each material. Based on the observations, the fluorine content in GIC is 7.49% of the total ions, while the fluorine content in Giomer is only 4.13%. Previous studies that comparatively evaluated the amount of fluorine release and aesthetic restoration material release in GIC, RMGIC, Giomer, and Compomer showed that the highest ability to release fluorine is achieved by the GIC material followed by RMGIC, Giomer, and Compomer [48].
The ability of fluorine release affects the anti-cariogenic and antibacterial properties. In other studies on fluorine release and the antibacterial properties of the polyacid-modified composite Dyract AP, it was found that the rate of fluorine release can affect the ability to prevent the development of caries-causing bacteria. In this study, Yotis and Brenann concluded that fluorine ions’ differences in bacteria binding were influenced by the quantity and susceptibility of fluorine binding to bacterial cells [49]. The ability to release fluorine in Giomer results from reactions in the S-PRG filler content through ligand exchange [40]. This conclusion is strengthened by the microscopic image results using the Scanning Electron Microscopic (SEM) test. In this study, the author used a 2500x magnification. In the GIC, there is a difference between the biofilm images compared with the biofilm images of Giomer. The biofilm image on the surface of GIC resembles a soft, thin layer, while the biofilm image of Giomer shows a dense and very clear biofilm colony. In previous studies on the differences in the image of biofilms on GIC and TiO2, the biofilm’s appearance on the surface of GIC was thin and not dense compared to other materials [50]. The development of biofilms in the material is influenced not only by the ability to release fluorine from the material but also by the surface roughness of the material. On irregular surfaces, bacteria will adhere for a longer time [51]. Based on this discussion, it can be concluded that a higher fluoride release in GIC can result in the formation and accumulation of fewer bacterial biofilms. In contrast, for the lower fluoride release of Giomer, there was a formation of biofilm accumulation.
CONCLUSION
Within the limitations of this study, the following conclusions can be made:
- Giomer showed a significant high compressive strength compared to GIC
- GIC showed inferior in term of surfaces morphology characteristic compared to Giomer
- Among the tested material, fluoride content in GIC is slightly higher than Giomer in qualitative analysis
- A higher fluoride release in GIC can result in the formation and accumulation of fewer bacterial biofilms
- The lower fluoride release of Giomer can result in the formation of biofilm accumulation.
ETHICS APPROVAL AND CONSENT TO PARTICIPATE
Not applicable.
HUMAN AND ANIMAL RIGHTS
Not applicable.
CONSENT FOR PUBLICATION
Not applicable.
AVAILABILITY OF DATA AND MATERIALS
Not applicable.
FUNDING
The authors would like to thank Universitas Indonesia for funding this research through PUTI Grant with contract number BA-1630/UN2.RST/PPM.00.03.01/2020.
CONFLICT OF INTEREST
The authors declare no conflict of interest, financial or otherwise.
ACKNOWLEDGEMENTS
Declared none.