The Evaluation of Osteoblastic Cell Behavior on Treated Titanium Surface
Abstract
Background:
There are several potential advantages in optimizing the initial events of osseointegration in the benefit of clinical outcome.
Objective:
The objective of the present study was to evaluate the behavior of osteoblastic cells on surfaces treated by double acid etching using HNO3 and H2SO4.
Methods:
Commercially pure titanium (grade 4) discs measuring 6 mm in diameter and 2 mm in thickness were used. The discs were divided into two groups: machined group and double acid-etched discs (HNO3 and H2SO4). Surface characteristics were assessed using Scanning Electron Microscopy. Pre-osteoblastic MC3T3-E1 cells were used for cell culture on the tested surfaces to assess proliferation, viability (MTT), as well as secretion (ELISA) and cytoplasmic expression (Western blot) of type I collagen.
Results:
The data obtained were analyzed using t-test or two-way ANOVA followed by Bonferroni’s test at 95% significance. The titanium surfaces showed average roughness values for the machined and treated surfaces of 0.29 and 1.16, respectively (p<0.05). An increase in cell proliferation was observed, which was corroborated by the viability assay. Both type I collagen secretion and intracellular expression were higher on the double acid-etched surface compared to the machine surfaces (p<0.05).
Conclusion:
Implant surfaces treated by double acid etching positively affected the early events of the interaction between titanium and osteoblastic cells, suggesting optimization of osseintegration.
1. INTRODUCTION
Titanium is commonly used in dental implants because of its well-established biocompatibility [1]. The presence of a titanium implant during bone healing induces signaling for the activation of the immune system via inflammatory mediators and therefore permitting osseointegration [2]. In addition, the adaptive capacity of bone to dental implants is under the influence of stress and tension due to mastication, the initial period of osseointegration being the critical point to promote anchorage of dental implants [3]. Despite such biocompatibility of titanium, some strategies have been used to improve anchorage of dental implants, e.g. different surface treatments.
The Macro and microgeometry of the implant have been reported to play an important role in the process of bone healing [4]. As for macrogeometry, implant design and the number and position of implant threads can influence osseointegration, along with surface treatments [5], which can greatly reduce the time required for satisfactory osseointegration [6, 7].
Previous studies have shown faster osseointegration of dental implants with greater surface area obtained after treatments, characterized by the formation of new bone, which results in improved predictability of immediate or early loading procedures [8-10]. Among the surface treatment types, many commercial brands use subtractive treatment with acids, which in addition to allowing decontamination of the surface with homogeneous removal of the oxide layer, results in greater roughness.
Among the various acid solutions used, the immersion of titanium implants in a mixture of hydrochloric acid (HCl) and heated sulfuric acid (H2SO4) (double acid etching) has been widely used to modify the titanium surface. Double acid-etched surfaces enhance osteoconduction by attaching fibrin and osteogenic cells, resulting in bone formation directly on the implant surface [11]. In contrast, nitric acid treatment has been indicated after polishing in order to reduce the concentration of surface contaminants [12].
Thus, considering the potential advantages of HNO 3 ftfor titanium surface treatment, the objective of the present study was to evaluate the behavior of osteoblastic cells on surfaces treated by double acid etching using HNO 3 and H 2 SO 4 , in order to optimize the initial events of osseointegration,
2. MATERIALS AND METHODS
2.1. Sample Selection
Commercially pure titanium (grade 4) discs measuring 6 mm in diameter and 2 mm in thickness were used. The discs were divided into two groups: machined group and double acid-etched discs (HNO3 and H2SO4) mimicking the same surface treatment used in commercial implants (Intraoss®, Itaquaquecetuba, SP, Brazil).
2.2. Scanning Electron Microscopy
Surface characteristics of the samples were assessed using Scanning Electron Microscopy (SEM). The samples were mounted on metallic platforms (stubs) and viewed in a SEM, JEOL 6100 (Jeol USA Inc., Peabody, MA, USA) and the images processed on Adobe Photoshop.
2.3. Cell Culture
Pre-osteoblastic MC3T3-E1 cells were obtained from the American Type Culture Collection (ATCC, Manassas, VA). The culture conditions were DMEM/F-12 medium (LGC Biotechnology, São Paulo, SP, Brazil) combined with bovine fetal serum at 10% (LGC Biotechnology) and penicillin at 100 U/ml plus streptomycin at 100 μg/ml (Sigma, St. Louis, Missouri, USA). The culture medium was replaced every 2 to 3 days and the cell cultures were maintained in a moist incubator at 37 °C containing 5% CO2 and 95% air. For every experiment using cell culture, it must be considered that the disc of titanium was placed on the bottom of well plate and cell suspension was placed over the disk.
2.4. Cell Proliferation
Cell proliferation was ascertained by conventional cell counting. Briefly, the cells from 3 randomly chosen wells from each group were enzymatically retrieved using 0.25% trypsin and 1mM EDTA (Gibco) solution at 24h, 48h and 72h of culture. Cell counting was performed manually using a hemocytometer (Hausser Scientific, Horsham, PA) and reported as number of cells x 104.
2.5. MTT Assay
Cell viability was assessed using the 3-[4,5-dimethylthiazol-2-yl]-2,5- diphenyl tetrazolium bromide (MTT, Sigma) method at 24h, 48h and 72h of culture from 3 randomly selected wells from each group. The cells were incubated with MTT at 10% in culture medium (5 mg/mL) at 37 °C for 4 h. The MTT solution was then discarded by aspiration and 200 µL of Dimethyl Sulfoxide (Sigma) was added to each well followed by gentle agitation for 5 min on a plate shaker. Subsequently, 150 μL from each sample solution was transferred onto a fresh 96-well plate. Optical density was assessed at 570-650 nm on a spectrophotometer (Epoch; Bio-Tek, Winooski, VT) and the data expressed as absorbance.
2.6. Enzyme-Linked Immunosorbent Assay
Quantification of Type I Collagen was evaluated at 24h, 48h and 72h. Briefly, culture medium was collected from each sample (machined and double acid etching discs) and centrifuged at 336 g for 10 min and the supernatant was aliquoted and stored at -80°C. Type I Collagen quantification was performed using the Mouse Collagen Type I Kit (Wuxi Donglin Sci&Tech Development, Wuxi/Jiangsu Province, China) following the instructions provided by manufacturer. The values were expressed as ng/ml.
2.7. Western Blotting
Cells were cultured on the titanium discs from both groups for 24h, 48h and 72h. Cell lysates were then prepared by homogenization at 4°C and centrifuged at 1.000 g for 15 minutes at 4°C. Protein concentration was measured via BCA (Pierce, Rockford, IL). Protein extracts were separated in 10% sodium dodecylsulfate–polyacrylamide gels, transferred onto polyvinylidene difluoride membranes (Hybond; Amersham Biosciences, Piscataway, NJ) and incubated for 1 hour with the primary antibodies anti-type I collagen (1:1000; Abcam, Cambridge, UK) diluted in TBST + 5% skimmed milk and the anti-GAPDH (1:1000; Santa Cruz Biotechnology, Santa Cruz, CA), which was used as an endogenous control. After incubation with a rabbit monoclonal secondary antibody (1:2.500), the reaction was revealed using Western blotting chemiluminescence detection reagents (Opti-4CN; Bio-Rad Laboratories, Hercules, CA) on a Luminescent Image Analyzer (ImageQuant LAS 4000, G&E). Optical density measurements were established on ImageJ 1.37 (National Institutes of Health, Bethesda, MD). All experiments were performed in triplicate. Fold induction was calculated by normalizing relative optical density values to that of the control value.
3. RESULTS
Ultrastructural analysis of the titanium surfaces showed that the double acid-etched surface presented characteristic peaks and valleys of such surface modifying treatment (Fig. 1A) whereas the machined surfaces showed irregular grooves resulting from the machining process (Fig. 1B). The machined titanium disks had lower roughness values, with an average of 0.29 (± 0.15) in relation to the double acid-etched surface 1.16 (± 0.20). This difference was statistically significant (p <0.001). The data are shown in Fig. (1C).
The results showed an increase in cell proliferation over time, especially after 72h, with significantly greater proliferation of osteoblastic cells on the double acid-etched surface compared to the machined surface (Fig. 2A). Corroborating the cell proliferation assay, an increase in cell viability was observed on both surfaces over time (Fig. 2B), though this was not statistically significant (p>0.05).
The results from type I collagen secretion by the osteoblastic cells cultured on both surfaces analyzed are presented in Fig. (3). The treated surface showed significantly higher synthesis of type I collagen in relation to the machined surface at all evaluated times (p <0.05). The expression of type I collagen by extracts of osteoblastic cell cultures after 24h, 48h and 72h was performed using Western blotting. As demonstrated in Fig. (4), type I collagen was overexpressed in all analyzed time periods for the discs with treated surface, reiterating the secretion findings obtained from ELISA.
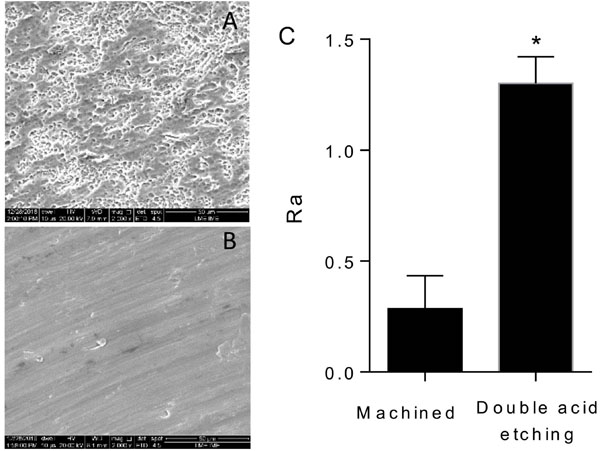
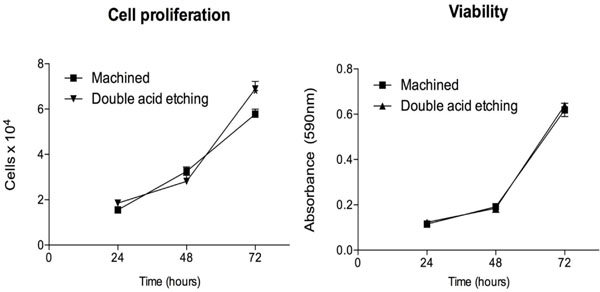
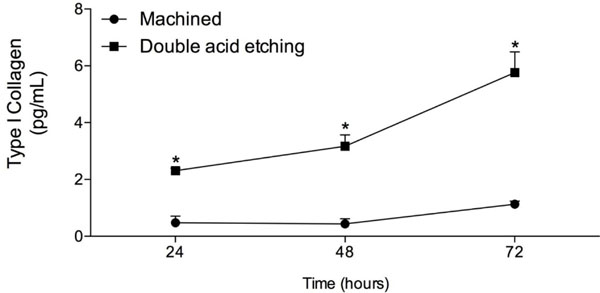
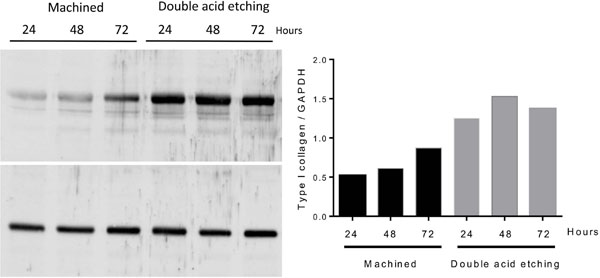
4. DISCUSSION
In addition to roughness, the treatment of dental implants surfaces with acids increases surface area for various cellular responses, including adhesion and migration of cells involved in osseointegration [ 13 ] . Thus, different acid etching strategies have been adopted by the companies, involving type, concentration, time and temperature of the acids used for the treatment of implant surfaces. Although many studies have been conducted using H2SO4 and HCl solution for titanium surface treatment, the present study was carried out to evaluate the etching treatment with HNO3 and H2SO4, in the initial events of osseointegration.
A series of coordinated events, including cell proliferation, cell differentiation and bone tissue synthesis, may be affected by different surface topographies [14]. In this context, surface roughness has a positive influence on cell migration and proliferation, modulating the tissue-biomaterial interaction, which in turn, allows better bone-implant contact results [15]. As demonstrated by the SEM images and confirmed by the roughness test, the surface treatment with double acid etching was effective, showing the formation of pores of similar dimensions in the areas analyzed. In recent years, much interest has been placed on the nanoscale and chemical modifications of surfaces and the resulting impact on osteogenic capacity. In the present study, the acid etching method used yielded a mean roughness value of 1.16 (± 0.20) μm, regarded as moderate parameters [16]. A moderately rough surface promotes osteoblastic differentiation and osteogenesis in both micrometric and nanoscale scales and increases the rate of bone-implant contact, thus increasing biomechanical interconnection [17].
The roughness values obtained were effective with respect to the biological behavior of the surface treatment with double acid etching, since it upregulated the proliferation of osteoblastic cells and, consequently, greater production of type I collagen, which suggests a shorter time for implant incorporation into bone [18]. Despite other extracellular matrix proteins exert an important function for bone mineralization, type I collagen is a key protein for bone formation, and consequently, favoring bone healing and early events of osseointegration [19].
Implant installation into the alveolar bone induces a cascade of healing events starting with the formation of a clot followed by maturation of the bone in contact with the implant surface. From the biological point of view, osseointegration is associated with decreased inflammation and gene expression upregulation associated with osteogenesis, angiogenesis and neurogenesis during the early stages of wound healing [20]. Bone remodeling begins with osteoclast bone resorption characterized by the dissolution of inorganic bone matrix. Osteoblasts then secrete non-collagenous proteins that permeate surface irregularities and undergo mineralization, forming a thin layer of non-collagenous mineralized extracellular matrix known as the “cement line”. Subsequently, osteoblasts secrete collagen fibers that become organized, mineralized and bond to the cement line [21]. Interestingly, both ELISA and Western blotting assays demonstrated that the surface treatment used in this study was able to stimulate the osteoblastic cells to synthesize larger amounts of type I collagen.
In addition to its structural function, type I collagen is secreted by osteoblasts and represents approximately 90% of the extracellular bone matrix, being considered an early marker of bone development and osteoblastic differentiation [19]. In this context, collagen I is indispensable in the initial events of the formation of mineralized matrix. Several studies in vitro have shown that increased expression and secretion of type I collagen are important factors for good biological performance of treated titanium surfaces [7] which corroborates the findings of the present study.
CONCLUSION
Based on the results obtained in vitro , implant surfaces treated by double acid etching with HNO 3 and H 2 SO 4 positively affected the early events of the interaction between titanium and osteoblastic cells.
ETHICS APPROVAL AND CONSENT TO PARTICIPATE
Not applicable.
HUMAN AND ANIMAL RIGHTS
No animals/humans were used for studies that are the basis of this research.
CONSENT FOR PUBLICATION
Not applicable.
AVAILABILITY OF DATA AND MATERIALS
The datasets analyzed during the current study are available from the corresponding author upon request.
FUNDING
This work was supported by Conselho Nacional de Desenvolvimento Científico e Tecnológico (CNPq) Brazil (Research Productivity Fellowships were awarded to MHN and EFM) - 303493/2016-0 and Coordenação de Aperfeiçoamento de Pessoal de Nível Superior (CAPES), Brazil - 001.
CONFLICT OF INTEREST
The authors declare no conflict of interest, financial or otherwise.
ACKNOWLEDGEMENTS
The authors wish to thank Pollyanna Tombini Montaldi for her excellent technical support.