Growth Hormone and Craniofacial Tissues. An update
Abstract
Growth hormone is an important regulator of bone homeostasis. In childhood, it determines the longitudinal bone growth, skeletal maturation, and acquisition of bone mass. In adulthood, it is necessary to maintain bone mass throughout life. Although an association between craniofacial and somatic development has been clearly established, craniofacial growth involves complex interactions of genes, hormones and environment. Moreover, as an anabolic hormone seems to have an important role in the regulation of bone remodeling, muscle enhancement and tooth development. In this paper the influence of growth hormone on oral tissues is reviewed.
INTRODUCTION
Growth hormone (GH)/insulin-like growth factor I (IGF-I) axis influences normal bone metabolism, and is a major regulator of postnatal growth and development [1]. GH is secreted by the anterior pituitary gland and acts directly on tissues via specific GH receptors or indirectly via the production of insulin-like growth factor I (IGF-I). Metabolic agents and hormonal systems such as growth hormone/insulin-Like Growth Factor-I axis, have a strong influence on the metabolism of oral tissues particularly during the period of growth [2, 3]. The process of bone modeling and remodeling is orchestrated by a constellation of local growth factors, cytokines, and systemic hormones of which GH and IGF-1 are key components [4].
The purpose of this study is to critically review the influence of Growth hormone/ Insulin-Like Growth Factor-I on oral tissues including the mandibular condyle. To find relevant articles, a Medline search from 1966 to March 2014 was conducted. The Medline search for English language articles was based on the key words ‘growth hormone’, ‘insulin-Like Growth Factor-I’, ‘oral tissues’ and ‘mandibular condyle’. Bibliographies of related articles were assessed for relevant studies to identify additional published references.
GH/IGF-I AXIS
Growth hormone (GH) is a 191-amino acid, 22-kDa polypeptide, that is synthesized and secreted by cells called somatotrophs in the anterior pituitary under the control of hypothalamus [1]. It has many actions in the body including the regulation of bone growth and metabolism [2-4]. GH has a pulsatile secretion with age-dependent concentrations characterised by low secretion in the prepubertal period, a rise at puberty (≈0.4–0.5 mg/24 h), and a decrease in old age [4]. GH secretion is under the influence of additional hormonal signals such as sex steroids and thyroid hormone, whereas glucocorticoids inhibit its secretion [5, 6]. GH circulates bound to a liver synthesized GH-binding protein [7]. GH affects several tissues including liver, muscle, kidney, and bone [8]. Insulin-Like Growth Factor-I (IGF-I) is critical for promoting the protein anabolic effects of GH [4, 5, 8]. The genes of the GH family are assigned to chromosome 17q23-q24 [9].
The somatomedin theory [1, 10] introduces the concept that GH stimulates skeletal growth by stimulating Insulin-Like Growth Factor-I (IGF-I) which, in turn, stimulates longitudinal bone growth in an endocrine manner. The somatomedin hypothesis was challenged by Isaksson et al., [11, 12] and Green and co-authors [13] who introduced the “dual effector theory”. According to this theory [14-16] the two hormones act independently at different stages of endochondreal maturation and differentiation. GH was found to stimulate young preadipocytes, whereas IGF-I stimulated cells at a later stage of development (Fig. 1).
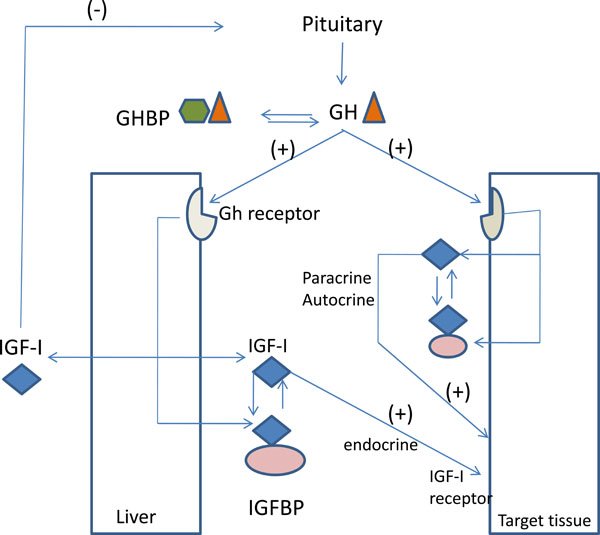
The GH–IGF axis. Abbreviations are: ALS, acid labile subunit; GH, growth hormone; GHBP, GH binding protein; GHRH, GH releasing hormone; IGF, insulin-like growth factor; IGFBP, IGF binding protein.
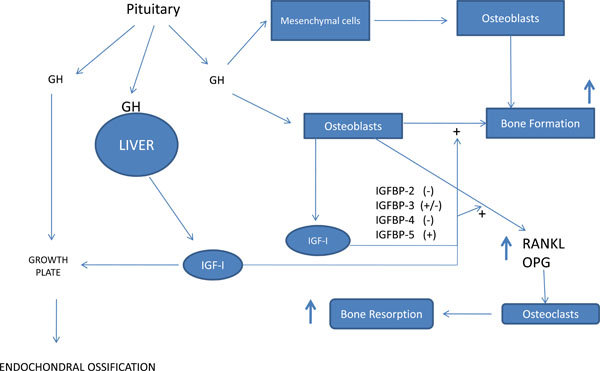
Growth hormone and bone metabolism. GH, growth hormone; IGFBP, IGF binding protein.
In support of a growth hormone direct effect, growth hormone receptor (GHR) has been detected in the hypertrophic zone of the cartilage that develops into a secondary ossification center [17, 18]. GH exerts pleiotropic effects on growth and development through GHR. The GHR consists of extra-cellular Gh-binding domain, transmembrane domain, and an intracellular domain involved in signal transduction [19]. The GHR belongs to class I of the hematopoietin superfamily of cytokine receptors, which includes more than 30 members, among others prolactin, erythropoietin, and leptin [14, 15]. GHR gene located on chromosome 5p13.1-p12 and is 87 Kb long, with 10 exons encoding 620 amino acids [19, 20]. GHR and IGF-I receptors has been immunolocalized in the PDL, along the adjacent mineralized tissue surfaces as well as in the dental tissues. These appeared to be fibroblast-like, mononuclear cells [21].
EFFECTS OF GH ON OSTEOBLASTS
GH and IGF-I are anabolic hormones and have the potential to regulate bone modeling and remodeling. Growth factors that regulate local bone metabolism include growth hormone (GH), insulin-like growth factor-I (IGF-I), epidermal growth factor (EGF) and interleukin-1 alpha (IL-1alpha). GH stimulates the proliferation in a number of osteoblastic cell lines and primary isolated cells of various origins including human cells [2, 16, 28]. It seems to stimulate the proliferation, differentiation and the production of type I procollagen, osteocalcin and alkaline phosphatase in osteoblastic cells [20, 22]. Furthermore, the osteoblasts responded to GH by expressing bone morphogenetic proteins (BMP) 2 and 4 [25]. BMPs that bind to surface receptors on progenitor and mature osteoblasts can trigger a signaling pathway that promotes osteoprogenitor cell differentiation and the upregulation of osteoblast activity, and periodontal ligament (PDL) cells.
The anabolic effects of IGFs on osteoblasts are modulated by several IGF binding proteins, mainly IGFBP-3, -4, and -5 [20, 23]. The stimulatory effect of IGF-I is increased by IGFBP-3 and -5, whereas it is decreased by the IGFBP-4 [24]. It influences osteoblastic function in all stages of development and increases the replication of cells of the osteoblastic lineage [26, 27]. These proteins are believed to function in several ways: by enhancing the binding of IGF-I to its receptor, by decreasing the bioavailability of IGFI; by controlling the transport of the IGF to specific cell types thereby potentiating its activity in skeletal tissues; and by direct IGFI independent actions on target cells [21, 23]. One can assume that the GH and IGF-1 signaling pathways serve both independent and overlapping functions in the skeleton [25-29] (Fig. 1).
Osteoblasts express also GHR, which is a member of the cytokine receptor superfamily [19, 23, 24]. IGFBPs regulate both the number and the activity of GHR through inhibition of IGF activity, recommending a local feedback of the GH/IGF axis at the tissue level by two possible mechanisms: i) liver and bone-derived IGF-I inhibits pituitary GH secretion, and ii) bone-derived IGF-I inhibits local action of GH by reducing GHR availability [20, 23, 25, 28]. It appears that some of the effects of GH on osteoblasts are mediated by IGFs, but others are not.
EFFECTS OF GH ON OSTEOCLASTS
When GH stimulates bone formation, bone turnover is enhanced, thereby releasing molecules from activated marrow stromal cells and osteoblasts that also lead to enhanced osteoclastogenesis and mature osteoclast activity [29]. When either GH or IGF-I was added to bone cells, the formation as well as the resorption activity of osteoclast-like cells was increased [30]. GH stimulates osteoclastic bone resorption through both direct and indirect (IGF-I and IL-6) actions on osteoclast differentiation. It has been demonstrated that IGF-I supports activation and formation of osteoclasts in cultures of unfractionated mouse bone cell [29], while human osteoclasts express functional IGF-I receptors [3]. During tooth eruption and tooth movement, alveolar bone osteoblasts and osteoclasts demonstrate GHR immunoreactivity. The greatest numbers of positive cells were located in the PDL space than on the mineralized tissues [29, 31] (Fig. 2).
EFFECTS OF GH ON ALVEOLAR BONE AND ORAL MUSCLES
Bone growth is a three-dimensional process and, as such, bone length and bone width naturally grow in proportion to each other. GH stimulates periosteal apposition through: i) the action of osteoblasts that add mineralized tissue on the outer (periosteal) bone surface, a process called periosteal apposition [32, 33] and ii) indirectly through the forces acting on bones by the muscles, itself being regulated by anabolic effects of GH and IGF-1 on muscle tissue.
GH exerts growth-promoting and metabolic effects in target tissues by binding to the transmembrane GHR and triggering enhanced GHR association with, and activation of the cytoplasmic tyrosine kinase JAK2 [9, 19]. Skeletal muscle cells respond rapidly to GH administration through increased tyrosine phosphorylation of the GHR [34]. GHR is required for normal skeletal muscle development. First, by regulating myoblast production of IGF-1, normal myofiber type specification, myonuclei accumulation, and expansion of myofiber diameter [35]. Second, promotes GH functions (independent of IGF-1) facilitating normal insulin action in skeletal muscle, which ultimately impacts global nutrient metabolism [36, 41]. It should be noticed also, that muscle mass accompanied by increasing muscle strength, preceded and exceeded any bone mass gain during GH therapy [37-41]. Sotiropoulos et al., [41] has shown that GH signaling profoundly influenced muscle mass, predominantly by promoting fusion of myoblasts with nascent myotubes. Moreover, have shown that mice globally deficient in GH receptor have reduced muscle mass with defective myofiber specification and growth. Similar, the loss of anabolic GH/IGF-1 action on GHD individuals could affect muscle mass and muscle force and as a consequence the bone adaptation and the cortical thickness [42].
EFFECTS OF GH ON MANDIBULAR CONDYLE
The condylar cartilage in the mandible is a secondary type cartilage and it is unique among ossifying cartilages in the skeleton in that it is derived from cells of periosteal origin of the neural crest. Secondary cartilages are structurally distinct from limb growth plate and differ primarily in their superficial layers, which comprise a perichondrium in which prechondroblastic cells secrete a type I collagen rather than type II secreted by chondrocytes [43, 44]. In mandibular condyle, chondrogenesis is activated when the external stimuli, e.g., condylar repositioning, generate the differentiation of mesenchymal cells in the articular layer of cartilage into chondrocytes, which proliferate and then progressively mature into hypertrophic cells [45].
Molecular genetic analysis has shown that both GHR and IGF-I receptors are present in the chondroprogenitor and chondroblast layers of the mandibular condyle [46]. The local production of IGF-I is parallel to the distribution of IGF-I receptors, whereas the action of GH on this cartilage appears to be IGF-I dependent [47]. Under GH excess, local IGF-I synthesis is stimulated; the mitotic activity and the mature cells of the mandibular cartilage are increased, leading to more endochondral ossification [48, 49]. Conversely, a lack of GH decreases mitotic activity through less IGF-I synthesis, leading to less endochondral ossification [50].
Idiopathic short stature and Laron syndrome is caused by the dominant-negative defects of the intracellular domain of GHR, and affected individuals have smaller facial measurements too, including the length of the mandible [51]. The relationship between craniofacial morphology and GHR mutations (P56IT variant) was studied in normal adult Japanese males and females. At position 1777 in GHR, a transversion of amino acid from cytosine to adenine changed codon 561 from proline to threonine (P561T), affecting the cytoplasmic domain of the GHR [52]. Individuals without P56IT had a significantly greater mandibular ramus length than did those with P56IT. This suggests that the GHR gene P56IT variant may be associated with mandibular height growth and can be a genetic marker for it. When the relationship between craniofacial morphology and single-nucleotide polymorphisms (SNPs) in GHR were investigated in Chinese Han individuals [53], patients with a genomic polymorphism at codon 526 (I526L) of the GHR gene had a greater mandibular ramus length than the control group. Tomoyasu et al., [54] analyzed 5 single-nucleotide polymorphisms (SNPs) of the GHR in Japanese population found an association between P561T, C422F and mandibular ramus height. However, they couldn’t report a correlation between the I526L polymorphism of the GHR and mandibular ramus height like Chinese Han individuals. Similar are the results in the Korean population [55]. In addition, the effect of P561T heterozygous mutation on children with and without mandibular protrusion was investigated in Japanese children [56]. The mandibular linear parameters tended to be smaller in subjects carrying the heterozygous mutation, suggested that P561T variant functions as an inhibitory factor in the process of mandibular growth.
EFFECTS OF GH ON TOOTH TISSUES
The development of the dentition is an integral part of craniofacial growth, even though it is not closely related to general growth. At the cellular level, the differentiation of odontoblasts from the neural crest cells is a long process comparable with the process of osteoblast differentiation. GH is known to increase the formation of bone and hard tissues of the tooth (dentine, cementum, and enamel), as do bone morphogenetic proteins [25]. GH receptors are expressed in these tissues and could mediate local growth responses. This is found in the distal cytoplasm of the future amelodentinal junction [58]. Moreover, IGF-I receptors has been demonstrated in the early stages of rat molar tooth bud development in vivo [57]. Thus, GH may be the major regulator at IGF-I expression in the early stages of tooth development.
The effects of GH on odontogenic epithelial cells were investigated on the growing root tip of the incisors of 8 Lewis dwarf rats- four of them treated by GH (66μg/100gr body/wt) compare to 4 normal Lewis rats [57]. Significantly fewer odontogenic epithelia were found in dwarf rats, but in treated dwarf rats the numbers of nuclei in the internal enamel epithelium, stratum intermedium, and Hertwig root sheath were equivalent to normal rats. GH seems to increase cell proliferation of both the inner dental epithelium (IDE), dental papilla, as well as, in Hertwig's Epithelial Root Sheath (HERS) determining the root dimensions. They speculated that the epithelial stem cells are targets for GH as a mitogenic. The GH mitogenic stimulus is mediated through epidermal growth factor (EGF) which acts as a GH-dependent mitogenic. An alternative mechanism may lie in some feedback mechanism resulting from differentiation. Thus, if GH promotes the odontogenic cells to differentiate, feedback would induce the stem cells to divide to maintain the pipeline.
Similarly, in order to evaluate the effects of GH and IGF-I on odontogenesis in early bell stage, recombinant hGH (50 or 100 ng/ml), IGF-I (100 or 200 ng/ml) or fatal calf serum were added to the media of 16-day old, fetal mouse’s first molar tooth germs [58]. The GH-treated tooth germs had increased mitotic indices and higher cell densities in the dental papillae, while the greatest extent of differentiation and the higher volumetric changes were observed on IGF-I-treated germs. Moreover, GH and IGF-I induce production of morphogenetic protein 2 and 4 (BMP-2, BMP-4) and Transforming Growth Factor-beta superfamily, affecting the odontoblast differentiation as well as dentine formation. Growth hormone can be detected as early as the cap stage within the dental papilla. However, the undifferentiated dental mesenchymal cells of the dental papilla, up until the cap stage, showed very weak amount for GH receptor. The same is true for the IGF-I receptors. It seems that GH and IGF-I on the proliferation of the odontogenic mesenchyme is similar to that postulated for cartilage and bone i.e. that both are mitogenic, but that GH primes a small proportion of preodontoblasts which then become IGF-I sensitive. This suggests that the effect of GH on dentine growth in dwarf rats is to produce a larger population of odontoblasts subsequently active in dentine matrix deposition [59].
The effect of GH status on the cellular cementum as well as on the tooth shape and size was investigated in decalcified first mandibular molars of GH-excess, dwarf and GH-receptor-knockout mice [60, 61]. Cellular cementum reduced nearly 10 times in GH receptor-knockout mice, three times in GH antagonist mice, and increased twice in giant mice [60]. Moreover, morphometric analysis of 10 linear dentin matrix dimensions revealed that Dwarf animals showed smaller crown, shorter and smaller dentin roots and mesio-distal width at the cementoenamel junction (CEJ) axis. Interestingly, even if GH-excess models had longer roots, neither the mesio-distal crown width nor the root dentin thickness was affected by the GH excess [62].
Moreover, dental maturation has shown to be consistently delayed in GHD children, although to a lesser degree than skeletal maturation. Microdontia, missing third molars, smaller premolar crowns, and partial eruption of the permanent dentition as well as delayed dental age have been reported [63-68]. In idiopathic short stature children and GHD patients, GH administration helps to normalize skeletal growth but dental development is not significantly affected [67]. One the other hand, Krekmanova et al., showed an acceleration of dental maturity after 2 years GH therapy in GHD child [66].
DISCUSSION
Both modeling and remodeling are distinct physiologic responses to integrated mechanical and metabolic demands. GH acts directly on osteoblasts to stimulate bone formation whereas stimulate osteoclastic bone resorption too, through its direct action on the maturation of osteoclast precursor cells and through its indirect activation of mature osteoclasts, possibly via stromal cells [30]. Markers of bone metabolism, including serum alkaline phosphatase, procollagen 1 carboxylterminal propeptide, and deoxypyridinoline, increased significantly by GH administration [71]. Finally, GH powder application around dental implants placed immediately after tooth extraction enhances bone density and collagen fibers [72, 73].
Most experimental orthodontics studies have shown that 10–14 days are required for one complete bone remodelling cycle to take place [21]. The peak in bone resorption on the compression side occurs 7 days after orthodontic force application appliance activation. The amount of GH receptors was transiently decline in the compressed PDL area during the first three days. The initial depression of the amount of GHR is the result of the trauma of the orthodontic force application. However, the gradual increase in GHR immunoreactivity at days 7 and 14 (peak osteoclastic activity) suggests that cells have an altered function, possibly undergoing differentiation and proliferation [29]. Rh-Gh application on the periodontic cells can improve the recovery of pathologic changes caused by the orthodontic forces [74]. Orthodontic tooth movement seems to produce tissue reactions that are associated not only with local factors related to teeth and occlusion, but also with systemic factors related to bone metabolism [69, 70].
Furthermore, GH/IGF-I axis influences the loading-related bone formation modulating the responsiveness of bone tissue to mechanical stimuli by changing thresholds for bone formation [71-75]. Cortical bone formation rate and cancellous bone volume increases when bone is reloaded and IGF-I is added [76]. GH/IGF-I axis interacts with sex steroids in periosteal apposition challenging the traditional concept of androgen- stimulatory and estrogen-inhibitory effects on periosteal expansion [77].
GH affects muscle tissues too, which regulate cortical bone geometry [38]. Muscle enlargement is accompanied by increasing muscle strength leading to secondary adaptive bone gain [37]. Growth of the facial bones such as maxilla and mandible occurs partly from direct remodeling of the surfaces of the bone. Normal muscle development and function is a prerequisite for normal bone adaptation and enlargement. Administration of GH regulates muscle mitochondrial function by increasing the levels of several key mitochondrial proteins, and by switching fuel utilization toward fat oxidation [78]. In GHD not only the muscle weight is reduced, but also the myosin chain isoform and fiber type distribution is changed resulting in a deficit of periosteal bone formation [79]. Similar mechanisms in the craniofacial area could be account for the much reduced growth rate of facial bones in GHD individuals compared to normal one [49]. GH through periosteal apposition and muscular growth seems to play an important role in facial growth and development [33, 35, 38].
The condylar cartilage has quite a different embryologic origin than the cartilage of the cranial base synchondroses or the epiphyses of the long bones. In young individuals it displays some functional characteristics of both a growth plate and an articular cartilage [43]. The adaptive remodeling of condylar cartilage proceeds with the biomolecular pathway initiating from chondrogenesis and finalizing with osteogenesis. While condylar cartilage from the young animals responded only slightly to GH [47, 48] a significant response was observed on cartilage from the old animals [80]. It seems that at a later age, when the chondroblasts are no longer dividing in the condylar cartilage, the undifferentiated cells constitute the target of GH, similar to the condition in long bone epiphyseal cartilage [11, 15]. The increased Knowledge of the germinal cells that proliferate in mandibular condyle, as well as, the effects of growth factors, is promising application to clinical treatment in the near future.
The process of tooth formation is quite similar to that of bone formation. Dental mesenchyme cells differentiate into odontoblasts, which produce and secrete collagenous and non-collagenous matrix protein during dentin formation. GH is able to induce proliferation of epithelial stem cells in molar buds, along with preameloblast differentiation and dentine matrix formation [57]. Growth hormone, GHR and its binding protein are detectable during tooth bud formation at the embryonic cap and bell stages provoked morpho-differentiation, and dentinogenesis [81]. GH through morphogenetic protein 2 and 4 (BMP-2, BMP-4) and growth factor-b superfamily, effect the odontoblast differentiation as well as dentine and enamel formation [82] Inhibition of BMP-4 expression because of GH deficiency results in loss of tooth crown morphology [67].
The effect of GH supplementation on craniofacial growth has been studied in children with idiopathic short stature (ISS) or GHD and those born small for gestational age (SGA), as well as in children with syndromes or hypopituitary deficiency [49, 67, 82-84]. These children have small facial height and width, small head circumference, immature small face, protruding frontal bones, saddle nose and convex profile. Most cephalometric studies demonstrated short ramus height as well as small linear dimensions in the posterior cranial base and mandibular and maxillary lengths. Furthermore, the cranial base angle and gonial angle as well as the angle between the maxillary and mandibular planes are larger than normal. It seems that structures maturing more rapidly are more susceptible to the influence of external factors like GHD than more slowly maturing structures [85].
Despite the relatively widespread use of GH to augment stature, the effects of this practice on the growth of the craniofacial complex have not been extensively investigated. The greater increases in growth in the mandibular length (ramus, not the corpus) and posterior face height indicate that the interstitial cartilage growth of the condyles and spheno-occipital synchondrosis are influenced more by GH treatment than the periosteal and sutural growth sites [67, 83, 84, 86, 87]. As a general rule, the earlier the GH administration, the larger were the positive effects on craniofacial structures such as the posterior cranial base. Most studies have suggested delaying orthodontic treatment because GH therapy can cause unpredictable mandibular growth [88].
CONCLUSION
GH/IGF-I axis seems to elicit varying responses in craniofacial region. It plays a major role in regulating growth during childhood and adolescence and also regulates metabolism through its binding to the growth hormone transmembrane receptor. It especially affects growth sites with endochondral ossification, such as the condylar cartilage and the cranial base. It has the potential to regulate odontogenesis, bone modeling and remodelling, exerting its anabolic effects on both trabecular and cortical bone. Although the understanding of the role of the GH in craniofacial tissues has increased enormously in recent years, there are still lots of things which need to be the subject of research studies.