All published articles of this journal are available on ScienceDirect.
Temperature Profiles Along the Root with Gutta-percha Warmed through Different Heat Sources
Abstract
Objectives:
To evaluate temperature profiles developing in the root during warm compaction of gutta-percha with the heat sources System B and System MB Obtura (Analityc Technology, Redmond, WA, USA). Thirty extracted human incisor teeth were used. Root canals were cleaned and shaped by means of Protaper rotary files (Dentsply-Maillefer, Belgium), and imaging was performed by micro-CT (Skyscan 1072, Aartselaar, Belgium).
Methods:
Teeth were instrumented with K-type thermocouples, and the roots were filled with thermoplastic gutta-percha. Vertical compaction was achieved through the heat sources System B and System MB, and temperature profiles were detect-ed by means of NI Dac Interface controlled by the LabView System. With both heat sources, higher temperature levels were recorded in the region of the root far from the apex. When the warm plugger tip was positioned at a distance of 3 mm from the root apex, temperature levels of about 180°C were used to soften gutta-percha, and no statistically significant differences were observed between peak temperatures developed by the two heating sources at the root apex. However, a temperature level higher than 40°C was maintained for a longer time with System MB.
Results:
Statistically significant differences were observed in peak temperature levels recorded far from the root apex. Thus, with a temperature of about 180°C and the warm plugger positioned at 3 mm from the root apex, both heating sources led to a temperature slightly higher than 40°C at the apex of the root, suggesting that the gutta-percha was properly softened. Significance: A temperature level higher than 40°C was maintained for a longer time with System MB, thus providing an ad-equate time for warm compaction of the gutta-percha.
INTRODUCTION
Endodontic or root canal therapy is performed to protect a tooth when the pulp becomes infected, and sealing of the root canal by means of a synthetic material dates back more than two thousand years [1]. Since then, several root-end filling biomaterials have been proposed, and investigations have been undertaken to establish the sealing ability and inflammatory potential of these materials. It has been shown that amalgam causes more severe periapical inflammation than zinc-oxide-eugenol cements [2], while mineral trioxide aggregate leaks significantly less than amalgam [3]. Conversely, thermoplastic aliphatic polyesters [4] and reinforced polyesters [5,6] are susceptible to both alkaline and enzymatic hydrolyzates [7], while gutta-percha suggested a sealing ability superior to that of mineral trioxide aggregate [8]. Therefore, even if mineral trioxide aggregate and aliphatic polyesters are interesting materials for a tissue-engineering approach [9-12] to treatment of the root apex, these materials prevent long-lasting root sealing [7]. The hermetic sealing of the root canal system is considered a key factor for successful endodontic therapy, and gutta-percha is the most popular material used for this purpose [13-16]. Teeth undergoing endodontic treatment are often restored through the post-and-core approach [17-19]. Stability of the coronal restoration and its interfaces with dentin is fundamental to preserve root sealing [20]. Moreover, the hermetic sealing of the endodontic space also depends on the morphology and anatomical variations of the root canal. For mandibular first and second molars, the incidence of accessory canals, lateral canals, inter-canal communications, and apical delta is higher than 10% [21, 22]. Finally, the preparation of the isthmus and cleaning of the root canal are other important aspects to be considered to avoid the failure of endodontic treatment [23, 24]. Although cold condensation is a common technique to fill the root canal with gutta-percha [25], this method prevents hermetic sealing of the root [26], and the higher levels of force used to compact gutta-percha may result in root fracture [27]. To overcome this issue, thermoplasticized gutta-percha applied through a variety of warming methods has been introduced [16, 28]. Gutta-percha is a trans-1,4-polyisoprene-based thermoplastic resin [29], and it must be heated at about 40°C to soften the material and to allow for proper condensation and adaptation to the root walls [16]. The melting point of gutta-percha is about 60°C [30]. Similarly to aliphatic polyesters such as poly(caprolactone), characterized by the same melting temperature, gutta-percha can be considered a thermal energy storage system [31]. Several heating devices have been developed to warm gutta-percha for clinical procedures. These systems basically consist of a temperature-controlled electric heater that warms a metallic plugger [32]. Different constraints of this technique have been well-documented [32, 33]. The complexity of the three-dimensional architecture of the root canal [34] in some cases prevents positioning of the heated plugger close to the root apex; thus, the temperature may be insufficient to heat gutta-percha properly in the apical region. Conversely, higher temperature levels of the plugger can be harmful for the tissues involved in the tooth joint (i.e., the periodontal ligament) [36]. In a previous investigation, it was shown that by positioning the heat plugger at a distance of 5 mm from the root apex, peak temperature levels lower than 40°C were recorded in gutta-percha positioned in the root apex [32-38]. In the present in vitro investigation, the effects on temperature profiles developing along root canals endodontically treated by two heating sources, differing in the modality by which the heating power develops, were evaluated.
MATERIALS AND METHODS
Twenty human central maxillary incisors were selected. The root portion of each tooth was scanned by a fan-beam x-ray with µCT SkyScan 1072 (Aartselaar, Belgium) at the following settings: 10 W, 100 kV, 98 µA, and 1 mm thick. 2D lateral images of the specimens were obtained through a 180° rotation around the vertical axis at a pitch rotation step of 0.90°, and the x-ray exposure time was 5.9 seconds. The set of images was then processed through a modified Feldkamp cone-beam reconstruction algorithm, and digital data were processed with reconstruction software (NRecon V1.4.0; SkyScan). Figs. (1A) and (1B) show the 3D imaging of typical roots.
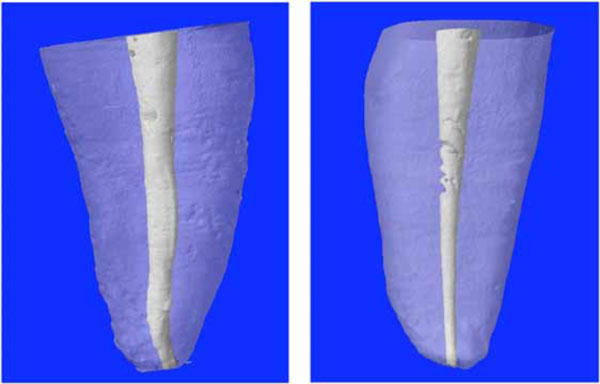
3D reconstructions of selected teeth. Typical roots with an almost straight (A) or more complex (B) geometry of the root canal.
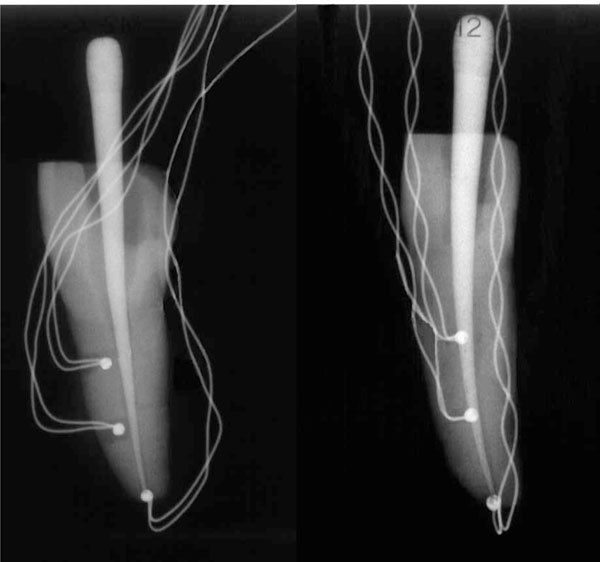
Teeth instrumented with type-K thermocouples. TA is the thermocouple positioned in the apex, while T1 and T2 are the thermocouples positioned at 5 mm and 10 mm from the apex, respectively.
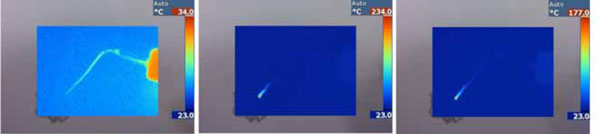
Temperature profiles along the heat pluggers detected from the thermal imaging camera. (A) Profile of the heat plugger before heating; (B) profile of the heat plugger after heating with System B; and (C) profile of the heat plugger after heating with System MB.
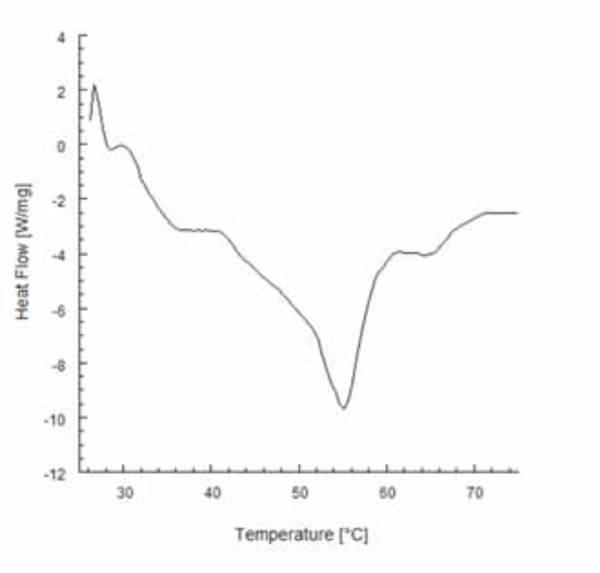
Typical DSC thermogram obtained for gutta-percha heated at a rate of 10°C/minute.
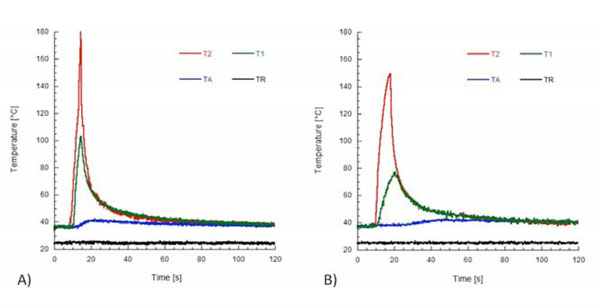
Temperature profiles recorded from the thermocouples with the plugger positioned at 3 mm. (A) Temperature recorded with System B. (B) Temperature recorded with System MB. TR is the room temperature.
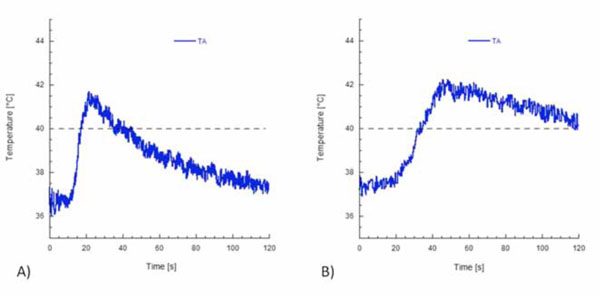
Temperature profiles at the root apex recorded with (A) System B and (B) System MB.
A water-cooled rotating diamond ball bur (diameter, 2 mm) and a Batt bur were used to gain access to the pulp chamber and the root canal. Hence, each root canal underwent a manual sequence of K files 0.8, 0.10, 0.15, and 0.20 (Dentsply-Maillefer, Belgium). The working length was then optically measured by means of an operatory microscope [24]. Mechanical treatment through the sequence of Sx, S1, S2, F1, F2 Ni-Ti Protaper instruments (Dentsply-Maillefer) was performed following the manufacturer’s instructions. Instrumentation was performed in conjunction with irrigation by a sodium hypochlorite and ethylenediaminetetraacetic (EDTA) water solution [36]. Two holes with a diameter of about 0.6 mm were drilled along the root of each tooth. These holes were positioned at 5 mm and 10 mm from the root apex. Type-K thermocouples (RS Components, Corby, UK), namely, T1 and T2, positioned at 5 mm and 10 mm from the root apex, respectively, were inserted into these holes and cemented with Duralay resin. A third thermocouple, TA, was inserted and cemented in the root apex. X-ray radiographs were performed to verify the correct positioning of the thermocouples (Fig. 2).
Each instrumented tooth was cemented with Duralay resin in a stainless steel tube, and a fourth thermocouple was used to monitor room temperature. A dedicated device consisting of a thermo-controlled heater and an aluminum block was used to control the temperature of the external environment of the tooth at 37°C. Thermocouples were connected to the NI DAC interface (National Instruments Corporation, Austin, TX, USA), and signals were recorded through the LabView system (National Instruments Corporation) at a rate of 10 points per second.
Gutta-percha cones (Dentsply-Maillefer) were adapted to seal each root canal. Teeth were randomly divided into two groups, each composed of 10 specimens, and the heat sources System B Obtura (Analytic Technology, Redmond, WA, USA) and System MB (Shibrun Medical s.r.l, FE, Coronella, Italy), equipped with a 30-04 heat plugger, were used to warm and compact gutta-percha according to the continuous wave technique [37]. System B was set immediately at a temperature of 180°C, while System MB was set at a temperature of 180°C, reached after 7 seconds. A sample consisting of 5 specimens of gutta-percha was analyzed through differential scanning calorimetry (DSC) with the DSC Mettler 25 apparatus. Temperature was increased at a rate of 10°C/min from room temperature to 70°C. A thermal imaging camera, Fluke Ti10 (Fluke Corporation, Everett, WA, USA), was used to detect temperature profiles along the heat pluggers. One-way analysis of variance with the OriginLab Pro7 software (Northampton, MA, USA) at a significance level of 0.05 using the Bonferroni method was performed to compare temperature data.
RESULTS
Temperature profiles along the heat pluggers detected from the thermal imaging camera are shown in Fig. (3). It is interesting to note that they are not uniform (Figs. 3B and 3C), and peak temperatures of 234°C and 177°C were detected for System B and System MB, respectively. Fig. (4) shows the calorimetric behavior of gutta-percha heated at a rate of 10°C/min. DSC thermograms showed a melting point for gutta-percha of about 56°C. The beta to alpha transition that occurred in the crystalline structure of gutta-percha was also observed, and the onset temperature was 40.6°C, while the end temperature was 42.7°C. These values are consistent with the findings of Song et al. [31]. Temperature profiles recorded from the thermocouples with the plugger positioned at 3 mm from the root apex are shown in Fig. (5). Lower peak temperatures were recorded from the thermocouple T1 of both systems (Fig. 5). These temperature levels may be sufficiently high to melt gutta-percha. Moreover, a temperature increase of about 5°C was measured at the apex of the root for both heating systems (Figs. 6A and 6B), thus suggesting
that the gutta-percha was sufficiently softened. It is worth noting that temperature profiles at the root apex strongly depended on the way the heat was transferred to the gutta-percha. However, no statistically significant difference was observed between the peak temperatures measured at the root apex with the two heating sources. In particular, peak temperatures at the apex of 41.6°C and 42.1°C were obtained with System B and System MB, respectively.
Table 1 reports the peak temperature levels and the rates of temperature increase recorded by the thermocouples TA, T1, and T2 for both systems. It is interesting to note that, for both heating systems, the rate of temperature increase depended on the position of the thermocouple, and higher rates were recorded for T2. For each thermocouple, the difference between the mean values of the rate of temperature increase with System B or System MB was statistically significant (P < 0.05). It is worth noticing that when the heat plugger was positioned at a distance of 3 mm, the gutta-percha was heated to a temperature of about 42°C with both heating systems (Table 1 and Fig. 6). The difference between the peak temperature levels when System B or System MB was used was not significant. However, a temperature level higher than 40°C was maintained for a longer time when System MB was used. Similarly to other polymeric materials, this effect is ascribed to the thermal energy storage capability of gutta-percha, that is, the capability of the material to absorb thermal energy and slowly release this energy during cooling [32]. Peak temperature values recorded by the thermocouples T1 and T2 with System B were statistically significantly different from those recorded with System MB (P < 0.05). However, the difference between the peak temperature levels recorded by the thermocouple TA was not significant. The time available to compact gutta-percha at a temperature higher than 40°C was about 20 seconds for System B (Fig. 6A), but higher than 60 seconds with System MB (Fig. 6B).
Peak temperature levels and the rate of temperature increase recorded by the thermocouples TA, T1, and T2 for System B and System MB.
Heating Source |
Peak temperature [C°] | Rate of temperature increase [°C/seconds] | ||||
---|---|---|---|---|---|---|
TA | T1 | T2 | TA | T1 | T2 | |
System B | 41.6 (0.6) | 101 (5) | 177 (8) | 0.39 (0.09) | 17.8 (0.9) | 33.1 (9.5) |
System MB | 42.1 (0.7) | 73.3 (8) | 149 (9) | 0.17 (0.06) | 8.4 (0.7) | 17.4 (8.4) |
DISCUSSION
Heating devices [34] have been developed to warm gutta-percha, and gutta-percha softening is an important factor for guaranteeing the hermetic sealing of the root canal according to the continuous wave technique [13, 15, 16, 37, 38]. The two heating sources implemented in our experiments differ in the modality by which the power is supplied to the root canal. Similar to the modulation of the curve power of light-curing devices [38], a soft start is implemented in System MB equipment. While System B provides almost constant heat power to the root canal for about 3 seconds, System MB develops a ramp power over 7 seconds. For both systems, temperature profiles developing along the heat plugger were not uniform (Figs. 3B and 3C), and peak temperatures of 234°C and 177°C are suggested for System B and System MB, respectively.
The effect of the modality by which the heat power is developed on the temperature profile recorded by the thermocouples is clearly evident in Fig. (5). In fact, steeper temperature increases can be detected for System B.The rate of temperature increase (Table 1) obtained by the use of System B was almost double than that achieved with System MB. For each thermocouple (Table 1), the difference between the mean levels of temperature increase for Systems B and MB was statistically significant (P < 0.05). Of course, this effect is also consistent with the higher temperature levels measured on the heat plugger of System B (Fig. 3), which allows for faster heat transfer to dental tissues and gutta-percha.
Also, peak temperatures strongly depend on the modality by which the heat power develops in the root canal. However, higher peak levels were detected far from the root apex (i.e., thermocouples T1 and T2). For both systems, higher peak temperatures were recorded from thermocouple T2. This temperature increase (higher than 100°C) may be ascribed to at least two reasons: The pluggers of both heating systems were not homogeneously warmed, and a lower temperature level can be recorded in the region of the tip. Moreover, the higher diameter of the plugger in the region close to the thermocouple T2 allows higher heat to be transferred to the surrounding environment. Peak temperature levels recorded by thermocouples T1 and T2 with System B were almost double than those obtained through System MB (Fig. 3 and Table 1). For each of these thermocouples, the difference between the mean levels of peak temperature achieved through System B and System MB were statistically significant (P < 0.05).
Surprisingly, differences between peak temperature levels in the root apex (i.e., thermocouple TA) with either System B or System MB were not significant. However, the temperature increase of about 5°C (Table 1 and Fig. 6) measured at the apex of the root for both heating systems suggests that the gutta-percha was sufficiently softened. In fact, temperature levels to be reached by gutta-percha to provide a proper condensation according to the continuous wave technique [36] depend on the calorimetric properties of gutta-percha (Fig. 4). It is important to provide a temperature increment of at least 5°C to soften gutta-percha and to benefit from the warm compaction. The distance of the heat plugger from the root apex is probably the most important factor affecting peak temperature levels in the root apex. By positioning the heat plugger at a distance of 5 mm from the root apex, we showed that peak temperature levels were lower than 40°C [38] and thus were not sufficient to soften gutta-percha [16]. Therefore, the shape of the heat plugger is also important to overcome the geometric constraints related to the complexity of the three-dimensional architecture of the root canal, which prevents deep insertion of the heat plugger into the root canal [20, 35].
Interestingly, the modality by which the heat power is supplied to the root canal affects the release of heat by the gutta-percha. From this point of view, gutta-percha behaves similarly to phase change materials, such as paraffins and aliphatic polyesters [5]. Also, melting points of gutta-percha (Fig. 4) and aliphatic polyesters [5, 31] such as poly (caprolactone) are very similar. When heated from body temperature, these materials are capable of absorbing thermal energy and slowly releasing this energy during cooling [32]. Fig. (6) clearly shows how the stored thermal energy is released by gutta-percha. The final portion of the temperature profile recorded by the thermocouple TA (Fig. 6) clearly shows the effects of the two heating systems on the steepness of the cooling cycle of gutta-percha. From a clinical point of view, this cooling steepness determines the time available to the clinician to perform the condensation of gutta-percha.
CONCLUSION
In conclusion, our results suggest that, during clinical procedures, if a heated plugger is positioned at a distance of 3 mm from the root apex, both the heating sources tested in this in vitro study might allow for a temperature sufficiently high to soften gutta-percha. However, compared with System B equipment, a temperature level higher than 40°C is maintained for a longer time when System MB is used, thus providing an adequate time for the clinician to perform the warm compaction of apical gutta-percha.
CONFLICT OF INTEREST
The authors confirm that this article content has no conflict of interest.
ACKNOWLEDGEMENTS
The authors are grateful to Prof. Sandro Rengo of the University of Naples "Federico II" for useful discussions.