Development of A Three-Dimensional Tissue Construct from Dental Human Ectomesenchymal Stem Cells: In Vitro and In Vivo Study
Abstract
Application of regenerative medicine technology provides treatment for patients with several clinical problems, like loss of tissue and its function. The investigation of biological tooth replacement, dental tissue engineering and cell culture, scaffolds and growth factors are considered essential. Currently, studies reported on the making of threedimensional tissue constructs focused on the use of animal cells in the early stages of embryogenesis applied to young biomodels. The purpose of this study was the development and characterization of a three-dimensional tissue construct from human dental cells. The construct was detached, cultured and characterized in mesenchymal and epithelial cells of a human tooth germ of a 12 year old patient. The cells were characterized by specific membrane markers (STRO1, CD44), making a biocomplex using Pura Matrix as a scaffold, and it was incubated for four days and transplanted into 30 adult immunosuppressed male Wistar rats. They were evaluated at 6 days, 10 days and 2 months, obtaining histological sections stained with hematoxylin and eosin. Cell cultures were positive for specific membrane markers, showing evident deviations in morphology under phase contrast microscope. Differentiation and organization were noted at 10 days, while the constructs at 2 months showed a clear difference in morphology, organization and cell type. It was possible to obtain a three-dimensional tissue construct from human dental ectomesenchymal cells achieving a degree of tissue organization that corresponds to the presence of cellular stratification and extracellular matrix.
INTRODUCTION
Research in the field of regenerative medicine and tissue engineering has been exponential and the possibility of replacing lost or damaged tissue through the development of tissue constructs is becoming more tangible [1]. With the discovery of adult stem cells from dental pulp (DPSCs) in third molars [2] comes the use of a new population of stem cells in the oral cavity, emphasizing research in the field of dental tissue engineering. Oral cavity stem cells have a highly proliferative capacity and high plasticity, and are considered as a new source of cells in tissue engineering with a wide range of applications. Recently, great efforts in the regeneration of dental tissues and teeth themselves have been made, mainly because the loss of teeth, either by decay, trauma, and periodontal disease has been considered a serious health problem. Different strategies have been proposed to achieve a biological replacement tooth. Within the most promising ones are the stimulation of the third dentition and the making of dental tissue constructs by bioengineered tissue using the components in isolated form, growing biodegradable scaffolds with stem cells and producing dental embryonic-like primordia of cultured cell populations [2]. Following this line of investigation, cell suspensions obtained from tooth germs and the bone marrow of rats, pigs or mice have been placed on the surface of biodegradable polymeric scaffolds and constructs of cells/polymers, which have been successfully re-implanted in immunosuppressed hosts [3]. Sharpe et al. performed experiments in order to replace a tooth through the development of dental tissue constructs from mice, primarily focusing on producing a dental primordium in the early stages of embryogenesis. In 2007, they conducted experiments using dental mesenchymal stem cells taken from tooth germs of mice, which lead to the formation of complete dental crowns [4]. In 2008, they determined the genetics and molecular biology of tooth formation, recognizing the signaling molecules expressed during this important process [5]. Tsuji et al. developed a technique to create a fully functional dental organ based on epithelial cells from normal mice and mesenchymal cells of eliminar espacio entre la coma y la palabra, obtaining a significantly smaller tooth with a similar shape compared to a normal tooth [6]. Even with all the studies reported to this date, the molecular mechanisms that regulate the interactions between epithelial and mesenchymal dental cells remain poorly understood. It is known that interactions between mesenchymal and epithelial cells (EP-MS) assure the development of all ectodermic organs such as teeth, hair follicles and salivary glands, therefore it is assumed that a better understanding of such regulatory mechanisms of the epithelial-mesenchymal interactions in tooth development, would not only help dental regeneration, but could also benefit the regeneration of other ectodermic organs [7-9]. Now, thanks to the research efforts that have been made to create a biological tooth, the bases have been set in the field of dental tissue engineering, focusing primarily on three important parts: the cell culture, scaffolds and the growth factors, all of which are considered essential components of the tridimensional tissue constructs [3].
Although, recent studies have reported that the size and shape of certain regenerated tissues such as skin, bone or cartilage can be controlled by the use of scaffolds, the shape and size of regenerated dental tissue have proven difficult to control. This is why a promising and potential solution proposed to the above is to replicate the natural process of developing a dental organ facilitating early sequence and reciprocal interactions between dental mesenchyma and epithelia [7]. Until now, the most successful models that have been preferred for the development of three-dimensional (3D) tissue constructs and therefore dental regeneration are: a) The cell dissociation-scaffold model, b) The combination of layers derived from cells of the enamel organ and dental pulp mesenchymal cells, and c) The drop model of collagen. This third method, considered ideal, is achieved by injecting a high concentration of epithelial and mesenchymal cells dissociated from early-stage tooth germs in adjacent regions within a collagen drop [7]. The current challenge restrains the development of reliable methods for regenerating teeth of predetermined shapes and sizes by using cells derived from postnatal tissues.
MATERIALS AND METHODS
Subject. A normal enucleated germ third molar was collected from a 12 years old male subject under approved guidelines set by Faculty of Dentistry, University of San Luis Potosi after informed parental consent was obtained. Permission to use human third molar was granted by the Bioethical Committee of the University.
The germ was placed in a 15ml conical tube with phosphate saline buffer in the presence of 100µg/ml streptomycin and100 IU/ml penicillin as antibiotics and 10 µg/ml amphotericin B, as antifungal (all from Sigma-Aldrich, USA) and preserved at 4°C until ready to use.
In Vitro
Cell Cultures
Once the tooth germ was obtained, epithelial cells were separated from the mesenchyma through the macroscopic identification by regions in the tooth germ with a scalpel blade # 15 as described by Tsuji [7] (Fig. (1A and B). Then the separated regions were cultured independently of one another by using the explant technique (Fig. 1C and D). The obtained cells were then cultured in EmbryoMax® ES (Millipore, USA) containing 100µg/ml streptomycin and 100 IU/ml penicillin as antibiotics and 10 µg/ml amphotericinB (all from Sigma-Aldrich, USA) as antifungal.
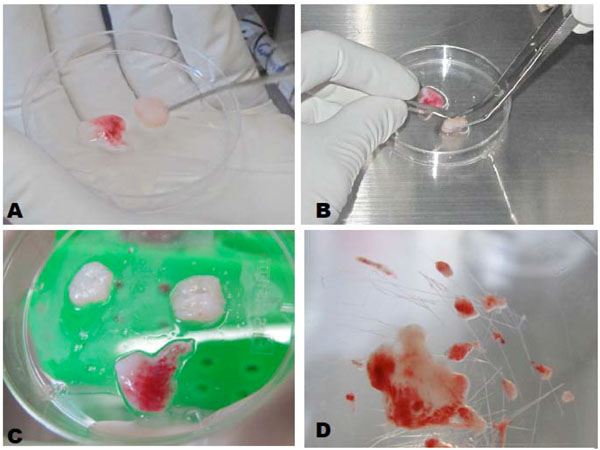
A, B. Macroscopic separation of epithelial and mesenchymal stem cells. C, D Explant culture technique.
Cell Cultures Characterization
Once the cultures were at confluence, some of these cells were fixed with 3.7% buffered formalin for indirect immune fluorescence assessment. Specific monoclonal antibodies used for mesenchymal stem cells were antiSTRO-1, anti-CD105 and anti-CD44, and as negative control, anti-CD34, anti-CD45. The antibodies for epithelial cells used were anti-cytokeratins 5 and 14 (Santa Cruz Biotechnology, USA). A monoclonal secondary antibody Alexa fluor 488 (Invitrogen) was used. The cells were grown on round glass cover slips (12 mm), after 12hrs they were fixed for 30 minutes with neutral formalin, permeabilized with Triton X100 0.025% for 20 min at room temperature, then blocked with BSA 1% 45 min. The first antibody was incubated for 2hrs at room temperature and the second antibody was incubated for 2hrs at room temperature and protected from light. Between each step, there were three washes with phosphate buffer saline. The observations were performed by confocal microscopy (Leica, Model DMI4000B, Germany) and LASAF ®software (Leica, Germany).
Biocomplex Development
Epithelial and mesenchymal stem cells were detached from culture dishes using TrypLE Express (Invitrogen, USA), then they were centrifuged and resuspended in culture medium and were quantified by Scepter (Millipore, USA). The drop model with modifications was selected to make the biocomplex, where one micro liter of mesenchymal cells (containing approximately 1000 cells) was used and placed according to the technique, in the center of the scaffold. 3 microliters of epithelial cells (containing approximately 3000 cells) were placed around the first type of cells in order to simulate the tooth germ [7]. Each biocomplex was incubated at 37°C, 5% CO2, and 95% air atmosphere for 4 days. For the control group which corresponded to a hydrogel drop without cells, 30 drops of Puramatrix hydrogel were prepared on micro slides and incubated at 37°C, 5% CO2, and 95% air atmosphere for 4 days.
In Vivo
Biocomplex Transplant
It was conducted on 30 adult male Wistar rats, approximate weight of 250gr, and immunosuppressed with cyclosporine A (10mg/kg/day). 4 study groups were chosen, one per quadrant of the rat´s mouth, as follows: Group 1: Positive control, without treatment; Group 2: Control extraction; Group 3: Control drop without cells and Group 4: Construct placed. Each trial was evaluated three times with assessments after 6 days, 10 days and 2 months, with 10 trials for each group at each time tested. The groups were randomly determined for the evaluation times for each test.
Animals were sedated with general anesthesia by intra peritoneal injection of ketamine (80 mg/ kg). First, we performed the extraction of a tooth of the rat, assuming that the dimensions of the socket would be appropriate (Fig. 2A, B, C and D). For those specimens where the size of the socket was not large enough, it was expanded with a surgical burr under drip irrigation with saline solution. A drop of the hydrogel was placed into the socket using a micropipette tip modified in the same way as for the biocomplex. Once the construct and a cellular drop were in place, the socket was closed with 7 zeros sutures (Ethicon, Johnson & Johnson).
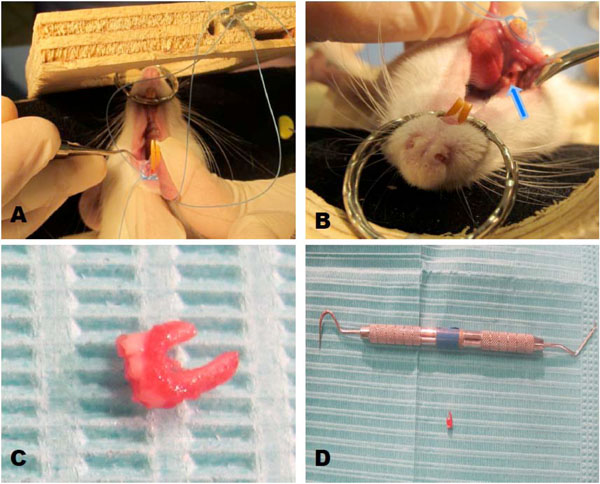
Biocomplex transplantation. A and B: Preparation of the alveolus or socket after removal molar. C and D. Extracted tooth.
Histological Characterization
Specimens were sacrificed by overdose of anesthetic, followed by the dissection of the mandible and maxilla of each rat.
Each segment of the maxilla and mandible was suspended in a test tube with 4% neutral formalin for 24 hours at 4°C. After fixation, the tissue was dehydrated in increasing concentrations of alcohol, cleared in xylene and finally embedded into paraffin. Histological sections obtained were 5 microns thick. The samples were stained with hematoxylin and eosin [10]. The observations were carried out by optical microscope DMI500 (Leica, Germany) and images were developed through the LAS EZ capture Leica System.
Results
Cell Cultures
It was possible to isolate and culture the mesenchymal stem cells and the epithelial cells from the tooth germ. In addition, the dental follicle was obtained from the separation. The mesenchymal stem cells showed an irregular morphology with an extended cytoplasm, whereas the epithelial cells showed a spindle shape (Fig. 3A and B).
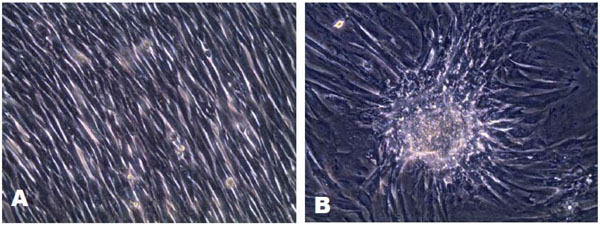
A. Epithelial stem cells with a fusiform morphology. B. Mesenchymal stem cells, cell clusters observed with extended cytoplasm and irregularly shaped cells.
Cell Cultures Characterization
The mesenchymal stem cells cultures were positive for STRO-1 antibody, anti-CD105 andanti-CD44, and negative for anti-CD34, anti-CD45and anti-HLA-DR. Meanwhile, the epithelial cells cultures were positive for cytokeratins 5 and 14.
Biocomplex Development
The biocomplex was developed using the drop model following the cellular placement explained above. Of the techniques tested in this study, we found that the drop model by inoculation with PuraMatrix (Table 1) was the most suitable. The diameter of each biocomplex measured approximately 6mm and after being incubated showed a higher cell concentration while retaining the cellular arrangement determined at the time of development.
Histological Description
The next observations were obtained after looking at every sample with the optical microscope:
- Positive Control; we observed intact dental organs; a tooth at the center shows the dental pulp composed of odontoblasts with the typical palisade organization at its periphery, with more fibroblasts and collagen fibers observed at the center. Next to the pulp the dentin was observed with the presence of dentinal tubules and cement perforated by periodontal ligament fibers. These fibers coated the tooth.
- Control extraction; we observed an empty alveolus. At the periphery, we observed the presence of root fragments, while in the surrounding bone a polymorphonuclear inflammatory cell type, fibroblasts and collagen fibers corresponding to granulation tissue was observed.
- Control acellular drop; at 6 days showed the presence of PuraMatrix in the empty alveolus. At 10 days, there was a size reduction of the matrix, and the presence of inflammatory cells and fibroblasts denoting granulation tissue. At 2 months, PuraMatrix was not present; in contrast granulation tissue typical of an alveolar repair process was observed.
- Six- day construct; it showed the presence of the construct implanted in the alveolus. It was evident that the cells were organized with two layers of cellse) Ten- day construct; cell proliferation was observed with a production of fibers in the internal structure of the biocomplex. In the outer portion the cells began to acquire a parallel organization in some areas.
- Two months construct; the samples were made at 3 different levels, one buccal, one medial and one lingual. They showed the presence of a structure formed by an internal mass of cells with a different organization d in the peripheral tissues. It was surrounded by an outer capsule of cells of different morphology and arrangement from the inner mass. Fibroblast-like cells were evident in the internal mass . It was also possible to observe the presence of capillaries and venules in the external cell capsule,and the internal mass (Fig. 4 and 5A, B, C, D, E and F).
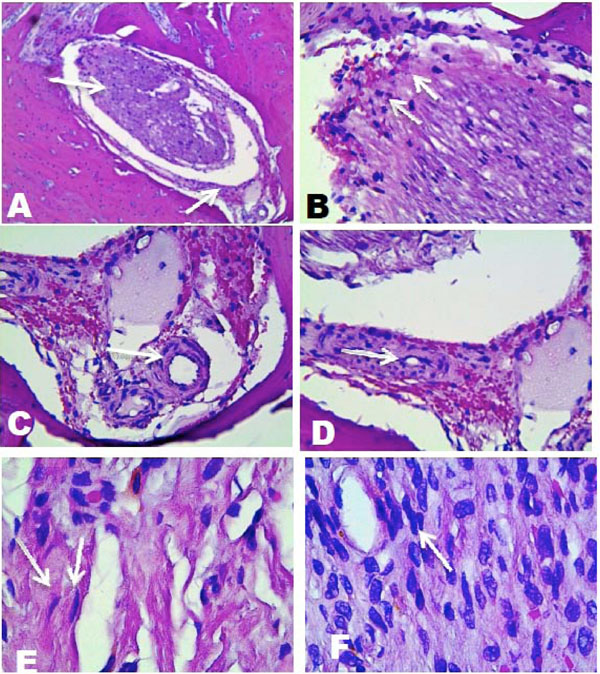
A), Histological analysis, vestibular side, 10X, peripheral cell layer surrounding most populated inner cell mass, B) Cells of the inner cell mass, 40X. C) Formation of capillaries, 40X. D) Capillaries in formations and extracellular matrix, 40X . E) and F) fibroblast-like elongated cells with different types of organization. 100X.
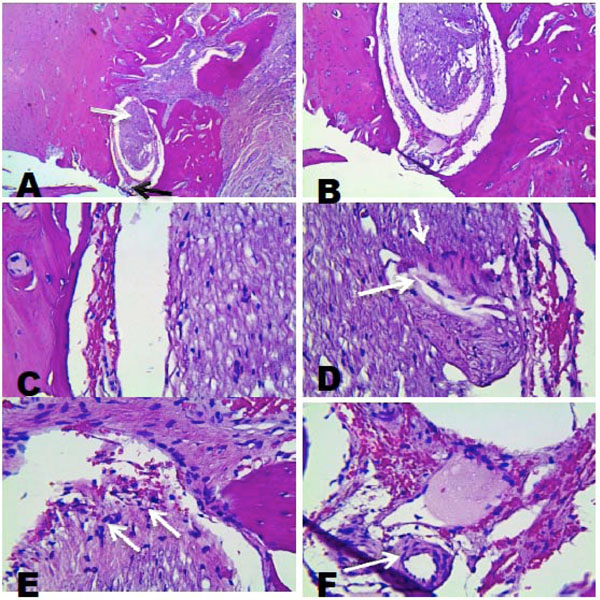
A), Histological analysis, lingual side. 4X, peripheral cell layer sorrounding an inner mass of cells. B) 10X, C) 40X , presence of blood cells. D) 40X, inner cell mass showing cells of different shapes and sizes. E) 40X, inner cell mass oriented to the external. F) 40 X, presence of blood vessels.
Statistics Data
Each group was evaluated at the three times mentioned above, having a series of 10 repetitions each (Graphic 1). In relation to tissue organization, it can be observed that the higher degrees of organization correspond to the construct groups at 2 months (80%) with organization of grade 2.The other experiments evaluated were observed with 0 and 1 degree of organization, with the exception of the positive group which had a grade 3 (Graphic 2). In relation to the presence of inflammatory cells, we observed them in the extraction controls at 6 and 10 days in 100% of the cases, while at two months these cells had decreased in number by 20%. Cell differentiation was observed in 100% of the positive control group and in 80% of the constructs of 2 months, as demonstrated by the presence of blood vessels and fibroblasts.
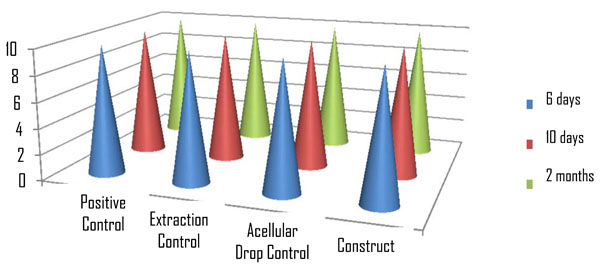
Control groups and constructs distribution.
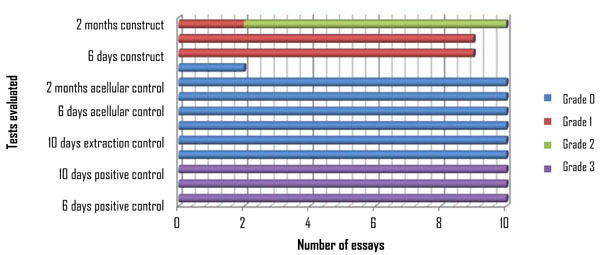
Tissue Organization.
STATISTICAL ANALYSIS
We applied chi-square statistical test with a confidence level of 99%. The statistical package used was JMPINV4.0.1. It was possible to observe statistically significant differences between the groups evaluated, noting that in regard to tissue organization, there is a statistically significant difference (p <0.0001, X2) between the construct with respect to the positive control group, extraction and drop acellular groups.
DISCUSSION
The existence of stem cells in the dental pulp, both permanent and deciduous, raises the possibility of using these cells to achieve autologous dental tissue regeneration in humans [11, 12]. Through previously reported studies, it is known that dental tissue regeneration is possible if the right environment is provided. These studies have focused on using stem cells from embryonic tooth germs of animals, mainly rats, mice and pigs, seeded in the surface of different biomaterials, particularly polymers, obtaining constructs that were successfully reimplanted in immunosuppressed animal models [7, 13, 14]. In the present study, we used stem cells taken from a permanent tooth germ, that is, adult stem cells of human origin, which were isolated and cultured to create a construct that was implanted in a biological immunosuppressed animal model (Wistar rats). Since Sharpe [5] has conducted several studies of the production of dental origin tissue constructs from murine stem cells, focusing mainly on the production of a dental primordium similar to the early stages of the embryogenesis [2, 5, 6]. Based on this, it was considered possible to generate a 3D dental tissue construct of human ectomesenchymal cells.
In previously reported studies, stem cells from tooth germs in the first 2 stages of formation [2, 5, 6, 15] reported favorable results. Our study, used stem cells obtained from a tooth germ, which were in an advanced bell-like stage [16]; and radiographically according to Nolla’s stages, at step 2 meaning an initial calcification. This corresponds to the third stage of odontogenesis, in which the enamel organ and dental papilla, an issue that was important for the determination of our longer evaluation time, can be distinguished.
The duration of this study was 6 days, 10 days and 2 months. These were defined based on the literature, since several studies reported results with similar evaluation times observing cell proliferation and differentiation. This research group evaluated their construct at 14 days and one month after transplantation, observing cell proliferation and differentiation of dental germs [7], however they used younger stem cells and Biomodels. In our study we decided to give a maximum time of 2 months, mainly because in our case, we used both adult cells and animal models, where the tissue regeneration can be decreased [17]. Consequently, the most promising findings were observed, both radiographic and histological, at the 2-months evaluation time, since in the 6 and 10 day times, it was not possible to observe a consolidated tissue behavior.
In reference to the histological findings; in the first week it was only possible to observe the construct in the rat alveolus preserving the cell arrangement given in vitro. This is, because at this early stage of transplantation, the graft tissue, in this case the construct, only binds with blood vessels and connective elements after the intervention, seeking a nutrition source, then receives external molecular signals through the surrounding tissue fluid [18-20]. At the same time, the host´s immune system “wakes up”, triggering the immune response, which due to cyclosporine A does not reject the construct and can follow the normal course of proliferation [21]. At 10 days, we could observe cell proliferation and fibers in the internal structure of the biocomplex. This was attributed to an established nutrition, where the construct is then initiating molecular signaling to the body. Thus, we observed an organization in the outer portion where the cells are arranged in several areas in parallel. This contrasts with the results obtained in the test at 2 months, where we see the proliferation and viability of our construct in the prepared alveolus. We observed the presence of cells organized into two distinct structures; one outer cell layer with an irregular morphology and blood vessels, In the center, there was an inner mass cell, with cells at different degrees of differentiation keeping a distinct spatial relationship with its external capsule. In this inner mass, we observed in histological sections, the presence of capillaries and fibroblasts, and cells in the periphery starting an orientation towards the external capsule, which is not possible to observe in the most superficial histological section.
However, in relation to the tissue organization shown by our constructs (Fig. 6), we observed that these range from grade 0, where tissue is absent and only the biocomplex fragments are seen (at one week), to a degree 2 wherein a cellular stratification is present and extra cellular matrix (at 2 months). This is in contrast to our positive control, which showed the dental organ tissues; enamel, dentin, cementum and dental pulp.
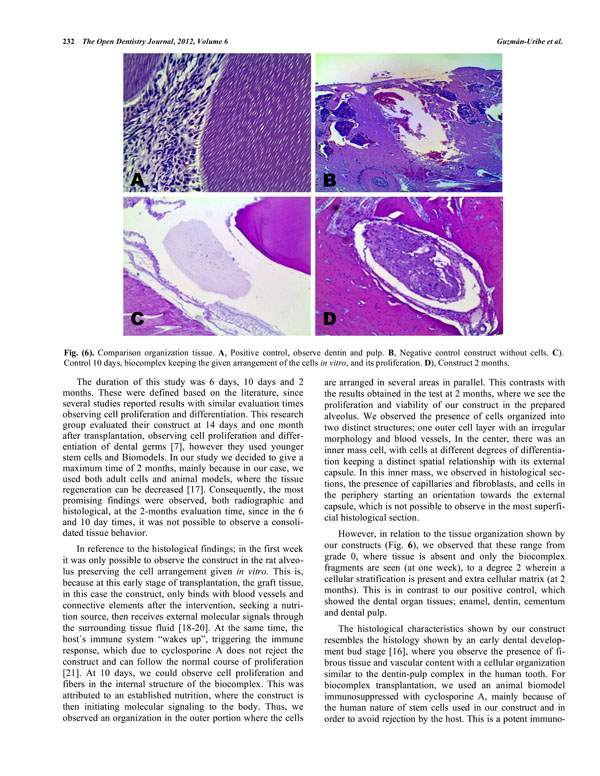
Comparison organization tissue. A, Positive control, observe dentin and pulp. B, Negative control construct without cells. C). Control 10 days, biocomplex keeping the given arrangement of the cells in vitro, and its proliferation. D), Construct 2 months.
The histological characteristics shown by our construct resembles the histology shown by an early dental development bud stage [16], where you observe the presence of fibrous tissue and vascular content with a cellular organization similar to the dentin-pulp complex in the human tooth. For biocomplex transplantation, we used an animal biomodel immunosuppressed with cyclosporine A, mainly because of the human nature of stem cells used in our construct and in order to avoid rejection by the host. This is a potent immunosuppressive drug showing very good results in therapies for various auto immune diseases and organ transplantation. Because the primary immunosuppressive action occurs on the T-lymphocytes it has become a good candidate for inducing experimental immunosuppressant in animals and humans [21].
In relation to the technique used in this study, the Drop model has been evaluated by other studies [6, 7], and is considered among the most successful in making 3D tissue constructs. Our experiment was carried out using three different strategies (Table 1). We found that the drop model by inoculating cells was the best option, as it met all three features that were fundamental to our study; structural stability, appropriate size, and easier handling during the whole process both in vitro and in vivo. Furthermore, the cell disposition given at the beginning of the formation of the biocomplex was preserved during all phases of our study, denoting the functionality of this technique for the development of such constructs.
Evaluation Drop Model Techniques
Technique | Structural Stability | Work Ability | Alveolus/Construct Proportion |
---|---|---|---|
Collagen sponge / PuraMatrix technique | + | + | + |
PuraMatrix mixed with cells technique | +++ | ++ | ++++ |
Cell inoculation into PuraMatrix technique | ++++ | +++ | ++++ |
On the other hand, the scaffold used, Pura Matrix, which is a synthetic hydrogel consisting of standard amino acids (1%) and water (99%), used to create 3D micro environments defined for a variety of cell cultures. Under physiological conditions, it is a 3D hydrogel showing a fibrous structure with an average pore size of 50 to 200nm, which greatly favors the establishment of the cells/extracellular matrix relationship. The hydrogel was used for our study because it is a biocompatible scaffold, resorbable, and is devoid of animal derived materials and pathogens. In addition, tests performed indicate the soluble material can be injected in vivo studies in animals and then form a 3 D hydrogel upon contact with a physiological medium. This was fundamental in our research and allowed cell proliferation.
Studies report that part of the side effects of performing an osteotomy is bone necrosis, mainly associated with the heat generated by friction of the work piece and the cutter used. This effect also increases when there is not enough irrigation, or the vascularity of the bone is reduced. In our particular study, probably the irrigation provided was not sufficient and in addition the vasculature may have been diminished.
Finally, it is important to note that the statistical analysis made in this paper, reveals significant differences between the construct group in relation to controls. The proliferation and differentiation, and the establishment of the new transplanted biocomplex tissue are due to the interaction of the construct with the surrounding tissues. Among the aspects that could be tested in future studies, we propose longer evaluation times, new biomaterials that can provide better features than our experiment, obtaining dental germ cells in earlier stages of odontogenesis, and use of different biomodels, among others. However, the current challenge remains the realization of reliable methods to generate dental tissue constructs of predetermined shapes and sizes using cells derived from human postnatal tissues, such as stem cells of the oral cavity.
CONCLUSIONS
It was possible to isolate and culture mesenchymal and epithelial stem cells from a tooth germ of human origin, and place this in an immunosuppressed rat socket. The dental tissue construct from cells of human origin, we observed to proliferate and organize similar to the earliest stages of odontogenesis in vivo. We found that the drop method using cell inoculation was a better strategy for the development of 3D tissue constructs. The hydrogel PuraMatrix was established as a suitable scaffold for these animal experiments to be stable both physically and biologically.
Ethical Approval of Studies and Informed Consent:
the protocol was evaluated in the ethical and legal aspects and bio safety by the members of the Ethics in Investigation Committee of the Stomatology Faculty of the UASLP with the following key: CEIFE-006-011.
Also there was an informed consent signed by the parents of the child that donated the germ tooth for our study.
CONFLICT OF INTEREST
The authors confirm that this article content has no conflicts of interest.
ACKNOWLEDGMENTS
We acknowledge Professor Takashi Tsuji, Tokyo University of Science, to orient us in the process of obtaining cell cultures.
This research was supported by PROMEP/103.5/10/5562 grant.