All published articles of this journal are available on ScienceDirect.
Adherence of Streptococcus mutans to Fiber-Reinforced Filling Composite and Conventional Restorative Materials
Abstract
Objectives.
The aim was to investigate the adhesion of Streptococcus mutans (S. mutans) to a short glass fibers reinforced semi-IPN polymer matrix composite resin. The effect of surface roughness on adhesion was also studied. For comparison, different commercial restorative materials were also evaluated.
Materials and Methods.
Experimental composite FC resin was prepared by mixing 22.5 wt% of short E-glass fibers, 22.5 wt% of IPN-resin and 55 wt% of silane treated silica fillers using high speed mixing machine. Three direct composite resins (Z250, Grandio and Nulite), resin-modified glass ionomers (Fuji II LC), amalgam (ANA 2000), fiber-reinforced composite (FRC) (everStick and Ribbond), and pre-fabricated ceramic filling insert (Cerana class 1) were tested in this study. Enamel and dentin were used as controls. The specimens (n=3/group) with or without saliva were incubated in a suspension of S. mutans allowing initial adhesion to occur. For the enumeration of cells on the disc surfaces as colony forming units (CFU) the vials with the microbe samples were thoroughly Vortex-treated and after serial dilutions grown anaerobically for 2 days at +37°C on Mitis salivarius agars (Difco) containing bacitracin. Bacterial adhesion was also evaluated by using scanning electron microscopy. Surface roughness (Ra) of the materials was also determined using a surface profilometer. All results were statistically analyzed with one-way analysis of variance (ANOVA).
Results.
Composite FC resin and other commercial restorative materials showed similar adhesion of S. mutans, while adhesion to dentin and enamel was significantly higher (p<0.05). Surface roughness had no effect on bacterial adhesion. Saliva coating significantly decreased the adhesion for all materials (p<0.05). Composite FC resin had a significantly higher Ra value than control groups (p<0.05).
Conclusions.
Short fiber-reinforced composite with semi-IPN polymer matrix revealed similar S. mutans adhesion than commercial restorative materials.
1. INTRODUCTION
The increasing attractiveness of tooth coloured restorations has promoted research in this particular area of dental material during last few years. Attempts have been made to change the type of fillers or filler size and their surface silanization, by changing the polymerization kinetics of resins matrices and degree of monomer conversion has tried to be influenced [1,2]. Reinforcing the resin with micro-glass fibres [3], with fiber-reinforced composite (FRC) substructure [4,5], whiskers [6], particulate ceramic fillers (dense and porous) [7] and optimization of filler content are among the methods that have been studied [1,8]. However, after many significant material improvements restorative composite resin still suffers lack of mechanical properties and problems related to polymerization shrinkage. Such shrinkage induces contraction stress at interface between composite resin and cavity walls leading to gaps formation and secondary caries. This is a major problem in current restorative dentistry. In addition, the rough surface of a restoration increases plaque accumulation, which may result in an increased secondary caries. In vivo and in vitro studies have shown that Streptococcus mutans (S. mutans) is one of the bacteria isolated in plaque samples from natural and artificial surfaces during early stages of caries development [9,10]. However, it is well known that the first stage of colonization by an organism involves adherence of the organism to a host surface. From this viewpoint, evaluation of S. mutans adhesion and colonization to tooth surfaces and restorative materials are of most importance for their success [9].
Glass fibers have been investigated to reinforce dental polymers for over 30 years [11]. They have documented reinforcing efficiency and good esthetic qualities compared to carbon or aramid fibers [11,12]. Polymethyl methacrylate (PMMA) and dimethacrylate based semi-interpenetrating polymer network (semi-IPN) matrix have been established as a polymer matrix in denture base materials [13]. Also some products of fiber-reinforced composite (FRC) use semi-IPN-polymer in the matrix [14]. Although previous investigations [15-18] on the use of experimental semi-IPN matrix in combination with short E-glass (2-3 mm) fibers in restorative filling composite show enhancement in flexural strength and load-bearing capacity, the effect of short glass-fiber reinforcement on bacterial adhesion and surface roughness has not been reported. It has been hypothesized that using isotropic short-fiber fillers will not effect on the surface roughness and bacterial adhesion of composite resin.
Thus, the aim of this study was to compare S. mutans adhesion and surface roughness of experimental short glass-fiber composite resin with different commercial restorative materials.
2. MATERIALS AND METHODS
2.1. Materials
Eight commercial restorative materials, three filling composite resins, resin-modified glass ionomers, amalgam, two fiber-reinforced composites and pre-fabricated ceramic filling insert were tested in this study. They are listed in Table 1. Enamel and dentin were used as control groups.
Materials Used in the Study
Brand | Manufacturer | Lot no. | Composition |
---|---|---|---|
FC | Experimental short fiber composite | Look at material part | |
Filtek Z250 | 3M ESPE Dental products, St paul, MN, USA | 20061003 | BisGMA, UDMA, BisEMA |
Grandio | Voco, Cuxhaven, Germany | 630615 | Aliphatic and aromatic dimethacrylates, 78% by weight filler |
Nulite | Hornsby NSW, Australia | 021703 | BisGMA based hybrid composite resin |
Fuji II LC | GC corporation, Japan | 540 1042 | Resin modified Glass-ionomer cement |
Amalgam (ANA 2000) | Nordiska Dental AB, Ängelholm, Sweden | 95127-3468 | Silver, copper, tin, zinc and mercury |
everStick | StickTeck Ltd, Turku, Finland | 2060727-ES-158 | E-glass fibers, PMMA, BisGMA |
Ribbond | Ribbond Inc, Seattle, Washington, USA | 9541 | UHMW-polyethylene, BisGMA |
Cerana class 1 | Nordiska Dental AB, Ängelholm, Sweden | 141002-26XL | Leucite reinforced glass ceramic |
PMMA, poly methyl methacrylate, Mw 220.000
BisGMA, bisphenol A-glycidyl dimethacrylate.
TEGDMA, triethylenglycol dimethacrylate.
UDMA, urethane dimethacrylate.
BisEMA, bisphenol A polyethylene glycol diether.
UHMW, ultra high molecular weight
Dimethacrylate (BisGMA 67% [bisphenol A-glycidyl dimethacrylate] and TEGDMA 33% [triethylenglycol dimethacrylate]) resin consisting 50 wt% nanofillers with an approximate diameter of 20 nm in size (SiO2) (Hanse Chemie, Germany) and E-glass fibers with BisGMA-PMMA [polymethylmethacrylate, Mw 220.000]) resin matrix (everStick, StickTech Ltd, Turku, Finland). In addition, radio-opacity fillers of BaAlSiO2 (3±2 μm in size) (Specialty Glass, USA) were incorporated to the resin system. Before the BaAlSiO2 filler particles were incorporated into the resin matrix, they were silane treated using previously reported technique [19].
Experimental fiber composites (FC) were prepared by mixing 22.5 wt% of silinated short E-glass fibers (2-3 mm in length) [15-17] to 22.5 wt% of resin matrix and then 55 wt% of BaAlSiO2-radio-opacity-fillers were added gradually to the mixture. The mixing was carried by using high speed mixing machine for 5 min (SpeedMixer, DAC, Germany, 3500 rpm). The dimethacrylate based resin matrix consisting PMMA forms semi-IPN polymer matrix for the composite of FC.
2.2. Methods
Nine specimens for each material (2 mm-thick disc with a diameter of 6.5 mm) were photo-polymerized for 40 s using a light source with an irradiance of 800 mW/cm2 (Optilux-500, Kerr, CT, USA). After polymerization, specimens were polished by diamond cub (Edenta AG, Switzerland) under water cool using low speed handpiece rotating at approximately 20.000 rpm. Discs from enamel and dentin (n=9, control) were obtained from freshly extracted sound third molars using a slow speed diamond saw (Ernst Leitz GMBH, Wetzlar 1600, Germany). Specimens were water stored for 24 h at 37°C before testing.
Measurement of Surface Roughness
The surface roughness of the specimen (n=3) was measured using a surface profilometer (Mitutoyo surftest 301, Mitutoyo Corporation, Kanagawa, Japan), with a standard cutoff of 0.8 mm, a transverse length of 0.8 mm, and a stylus speed of 0.1 mm/s. The roughness average (Ra) of a specimen was defined as the arithmetic average height of roughness component irregularities from the mean line measured within the sampling length. Three profilometer tracings were made for each specimen and numerical average was determined for each group.
Preparation of Saliva for the Adhesion Tests
Paraffin-stimulated saliva was collected from 4 volunteers. The pooled saliva was clarified by centrifugation (12000 g, 10 min), stored in aliquots at -70 ˚C. Before use it was thawed and diluted 1:5 with adsorption buffer (see below) [20].
Cultivation of the Microorganism and Preparation of Cell Suspensions
For the adhesion experiments Streptococcus mutans Ingbritt (kindly provided by Dr. Carlén, University of Göteborg, Sweden) was cultured on blood plates (Orion Diagnostica, Espoo, Finland) for 16 h at +37 ˚C in an anaerobic athmosphere. In these conditions the cells occur in suspension as singles, pairs or triplets (Fig. 1), which makes further homogenization unnecessary [20]. The cells were harvested from the plates with plastic loops, washed twice (10000 g, 10 min) with phosphate-buffered saline (PBS; 137 mM NaCl, 10 mM phosphate, 2.7 mM KCl, pH 7.4) and suspended in the adsorption buffer to an optical density of 0.35 (A550) corresponding to 5 x 108 CFU per ml [20].
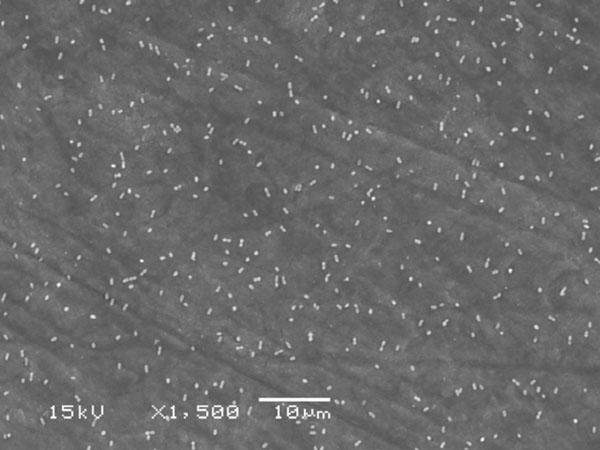
SEM photograph of saliva coated enamel surface with adhered S. mutans cells.
The cell density was checked in preliminary adhesion experiments showing in scanning electron microscopy (SEM) even distribution of the cells on the material surfaces with abundant space for surface colonization (Fig. 1).
Adhesion Tests
The adhesion experiments were essentially performed as described earlier for granular hydroxyapatite [20]. The discs (n=3/group with or without saliva) were first preincubated in 2 ml adsorption buffer/diluted saliva at room temperature for 30 min using gentle rolling (The Coulter Mixer, Luton, UK) in 14 ml capped plastic test tubes with an inner diameter of 16 mm (Falcon, BD Biosciences, Bedford, MA, USA). The mixer was tilted in a 15 degree angle to ensure that the discs were covered by liquid at all times. After the preincubation the discs were rinsed once in 50 ml saline (0.9% NaCl, Orion Diagnostica, Espoo, Finland) and then transferred to test tubes with 2 ml of the cell suspension prepared as described above. The discs were rolled in the cell suspensions for 30 min and then rinsed gently 3 times in 50 ml saline. Thereafter the attached cells from one side of the disc were scraped to 0.5 ml of transport medium (Tryptic Soy Broth, Difco Laboratories, MI, USA). Three applicators dipped into fresh transport medium before the procedure (Quick-Stick, Dentsolv AB, Sweden) were used to scrape the cells from the disc. The brush ends of the applicators were cut into the transport media. In preliminary experiments, one Vortex-treatment of the vials efficiently removed the bacteria from the brushes 1 and 2, increasing the number of scrapings from 3 to 4 did not increase the cell yield. The same method has been used to collect early plaque formed on different materials [21]. The experiments were performed with 3-4 replicates and repeated at least once.
For the enumeration of cells on the disc surfaces as colony forming units (CFU) the vials with the microbe samples collected from the surfaces were thoroughly Vortex-treated and after serial dilutions of the samples grown anaerobically for 2 days at +37 ˚C on Mitis salivarius agars (Difco).
In some experiments the materials were subjected to fixation followed by scanning electron microscope (SEM) as described below.
SEM
For the SEM examinations the samples were fixed for 5 min (2% glutaraldehyde and 2% formaldehyde in phosphate-buffered saline [Orion Diagnostica, Espoo, Finland], pH 7.4), rinsed once in distilled water and dried in an ascending ethanol series: 50% EtOH for 5 min, 70% EtOH for 10 min, two times 96% EtOH for 10 min and absolute EtOH for 5 min. Finally the specimens were sputter coated with gold and examined with SEM (Model JSM 5500, JEOL Ltd., Tokyo, Japan).
3. RESULT
Experimental composite FC resin and other tested materials showed similar adhesion of S. mutans (Fig. 2), while adhesion to dentin and enamel was significantly higher (p<0.05).
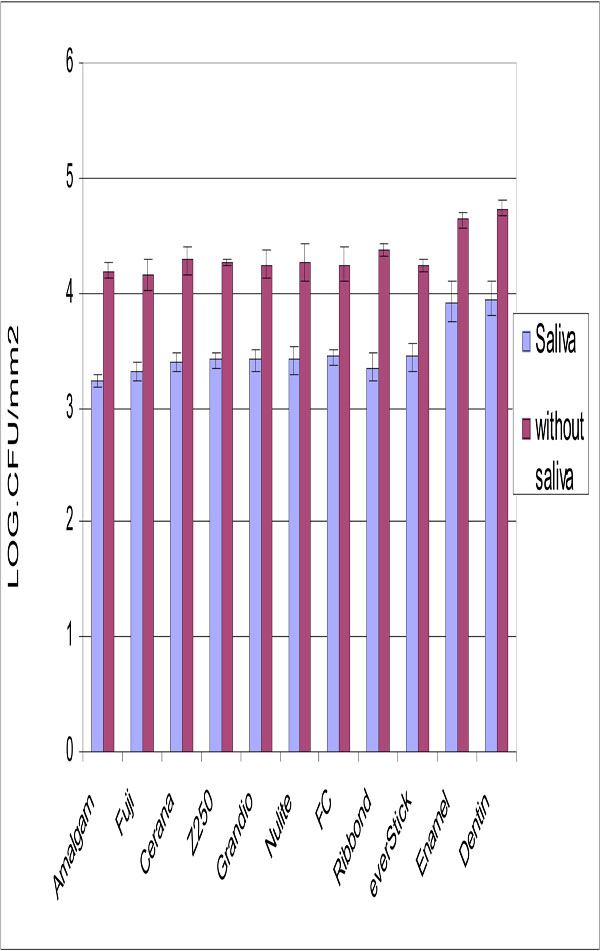
Number of adhered S. mutans cells on tested restorative materials with and without saliva surface precoating. Vertical lines represent standard deviations. Horizontal line above the bars indicates groups that statistically differ from others.
Saliva coating significantly decreased the adhesion for all materials but dentine and enamel still showed the highest S. mutans adhesion.
No relation was found between surface roughness and S. mutans adhesion. Significant differences were detected in the surface roughness of studied materials (Fig. 3). The lowest Ra value was obtained with specimens made from Cerana class 1 (0.31 μm ±0.1) and specimens made from Ribbond had highest values (2.33 μm ±0.31). Experimental composite FC resin had a significantly higher Ra value than control groups (p<0.05).
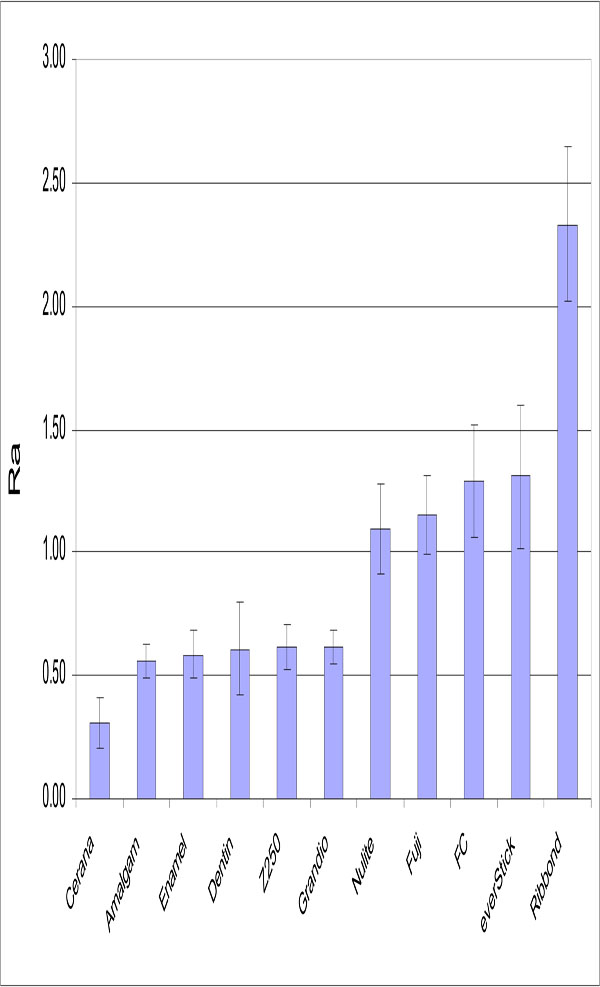
Mean Ra (μm) values of the materials tested. Vertical lines represent standard deviations. Horizontal line above the bars indicates groups that do not differ statistically from each other.
Scanning-electron microscopy of bacteria binding to non-coated and saliva-coated specimens revealed less bacteria on the specimens after saliva coating.
4. DISCUSSION
Bacterial colonization of S. mutans is usually the first step leading to secondary caries development. Adhesion of S. mutans to short fiber composite FC resin and different commercial restorative materials were studied in relation to surface roughness.
Based on the results of the present in vitro study S. mutans does not demonstrate difference adhesion to experimental composite FC resin than to other restorative materials tested (Fig. 2), despite of different surface roughness they have. Interestingly, adhesion of S. mutans was previously shown to increase with increasing surface free energy [9,21]. However, glass fibers with higher surface energy seemed to adhere same S. mutans than the restorative materials with lower surface energy. Our findings are in agreement with a studies by Tanner et al., which showed that glass fiber-reinforced composite (FRC) resembles restorative composite with respect of plaque accumulation and adherence of S. mutans [21,22]. Our observations clearly demonstrated no influence of surface roughness on S. mutans adhesion. This is in accordance with Eick et al., who demonstrated that no correlation found between surface roughness and the number of colony forming units (CFU) of S. mutans [23].
Enamel and dentin demonstrated higher bacterial adhesion than tested restorative materials. This is most likely due to hydrophobic interaction of tooth structure. Several authors showed the influence of saliva pellicle on the degree of bacterial adhesion in comparison with uncoated surfaces [24-26]. The results of the present study support these observations showing a lower degree of S. mutans binding after saliva coating on all tested materials.
Methodologically, one limitation of the present study is related to the scraping test of the cells and not labeling of them since we wanted to collect both sides of the material specimens. However, repeatability of the scraping method was good as judged by the small standard deviations of the replicates. This enabled also the assessment of CFUs and SEM detection from the same specimen.
The SEM was not a valid method to study the attachment since most materials were too uneven for SEM. Scanning results were in agreement with the scrapings (Fig. 2) to demonstrate how homogenous the bacterial suspension was with no S. mutans chains.
Mechanical profilometers have been used to measure surface roughness for in vitro investigations. The profilometers provide somewhat limited two-dimensional information. As apparent in Fig. (3), there were proportional significant differences between the Ra values of different restorative materials. The Ra value of composite FC resin was at same level to the commercial packable composite resin (Nulite) and resin-modified glass ionomers (Fuji II LC). On the other hand, Grandio and Z250 as hybrid composite resins revealed lower Ra value than other composite resins. However, the Ra value of short fiber reinforced composite resin was within the clinical acceptable range [27,28]. Roughness can be related to a combination of factors that include the characteristics of the matrix, ratio and size of inorganic particles, exposition of these inorganic particles and formation of air bubbles during material preparation. FC, Nulite and Fuji resins present the higher mean particle size according to manufacturer data. In contrast, Z250 and Grandio contain a smaller range of filler particles size than other composites tested, which may partially explain the lower roughness obtained with this composite resin. In addition, resin composite filler particles appear to play an intrinsic role in how well a composite finishes. In composites where the filler particles are significantly harder than the matrix, the resin phase may suffer a special loss during finishing. This will result in the positive relief of filler particles on the surface [29,30].
Another aspect reported in the literature that may have an influence on the surfaces roughness is finishing and polishing techniques [28,29]. However, in this study all specimens were finished to closely simulate clinical conditions, i.e. by using diamond-finishing cup. Moreover, finishing time, and press-on force should also be taken into consideration [31].
Based on the results of this study and our previous published data of short fiber composite resin [15, 32-34], it is suggested that experimental FC composite could be used successfully to fulfill the requirements for a posterior restoration. However, it should be emphasized that clinical trials are necessary in order to evaluate the usefulness of FC composite resin in dental restorations.
5. CONCLUSIONS
The new experimental short-fiber composite does not change the S. mutans adhesion tendency, although surface roughness values were higher than for commercial composite resin.
ACKNOWLEDGMENT
The authors gratefully acknowledge Mrs. Milla Lahdenperä, Ms. Jasmina Bijelic, and Ms. Oona Hällfors for kind help in this study.