All published articles of this journal are available on ScienceDirect.
Fracture Resistance in Severely Damaged Central Incisors Restored with Two-restoration Techniques: An In Vitro Study
Abstract
Background
The endocrown is defined as a fixed restoration crafted from a homogeneous, adhesive ceramic designed to restore the coronal portion of the tooth. It derives its support from the pulp chamber, with or without extension into the pulp canals.
Aims
This study aimed to compare the fracture resistance between IPS e.max press endocrowns and conventional crowns made from the same material, supported by a fiber post and composite core, in restoring endodontically treated maxillary central incisors after subjecting them to oblique compression loads.
Materials and Methods
A total of 20 extracted permanent maxillary central incisors were collected and randomly divided into two groups. The first group (n=10) was prepared to receive IPS e.max press endocrowns, while the second group (n=10) was restored using a fiber post and composite core and then prepared to receive IPS e.max press crowns. Subsequently, the fracture strength of the restorations was tested using a universal testing machine. Statistical evaluation was performed using SPSS 13.0.
Results
The mean fracture resistance was measured at 383 ± 68.65 N for the endocrown group and 333 ± 55.79 N for the conventional crown group. No significant difference was observed between the two groups regarding fracture resistance (p = 0.091).
Conclusion
In the context of this study, no difference in fracture resistance was found between the bonded ceramic endocrowns made of IPS e.max and the traditional technique when restoring severely damaged endodontically treated maxillary central incisors.
1. INTRODUCTION
The rehabilitation of endodontically treated and compromised anterior teeth remains a significant challenge for clinical practitioners due to the loss of resistance characteristics resulting from the removal of pulp tissue and a substantial amount of dental tissue [1]. The stability of restorative crowns is often at risk, necessitating the use of an intra-radicular core and post [2]. Despite the successes achieved with intra-radicular posts, they have several disadvantages, such as the removal of dental tissue from the canal to create space for the post [3]. The traditional method for rehabilitating these teeth involves restoring them with crowns supported by a core and post. However, many studies have indicated that this method may weaken the remaining dental tissues, thereby increasing the risk of accidental fracture of the teeth [4].
This situation is influenced by various factors, including the amount of remaining dental tissue, the properties of the post, the characteristics of the canal, the dimensions of the post used, and the type of material from which it is made [5]. Clinically, there is growing interest in selecting core materials that possess a modulus of elasticity similar to that of dentin, as this ensures better stress distribution and reduces the likelihood of irreparable fractures [6, 7]. The preparation of the root canal is a major factor that weakens the fracture resistance of teeth. Preparing the canal to accommodate a large post or inserting the post deeply within the canal can significantly reduce the fracture resistance of the teeth and greatly affect the apical seal [8].
The medical literature recommends that the post length be two-thirds the length of the root canal and, at a minimum, the post length equals the clinical crown length. Nonetheless, this issue remains a topic of considerable debate, as numerous laboratory and simulation studies have failed to demonstrate a clear effect of post length on the biomechanical properties and fracture resistance of teeth [8]. This traditional treatment pattern, particularly for upper incisors, may result in the loss of up to 58.3% of dental tissue. It also carries the risk of bacterial penetration into the periodontal tissues and the potential danger of perforating the canal during preparation [9].
Many systems of intra-radicular posts have been utilized, including metal posts and cores, as well as newer post systems, such as epoxy resin posts reinforced with carbon, epoxy resin or methacrylate posts reinforced with quartz or glass, polyethylene posts, zirconia posts, and polyether ether ketone posts [5]. The initial concept for the endocrown technique originated from the use of amalgam within the pulp chamber, extending it into the pulp canals by 2 mm to ensure greater stability [10]. The endocrown was first proposed by Bindl and Mormann in 1999 as a ceramic restorative alternative to the core and post [9].
The endocrown is described as a fixed restoration made from a homogeneous, adhesive ceramic designed to restore the coronal portion of the tooth. It derives its support from the pulp chamber, with or without extension into the pulp canals [9]. Endocrowns are often crafted from adhesive ceramics and resin composites, including feldspathic ceramic, leucite-reinforced ceramic, lithium disilicate ceramic, or resin composite. Various fabrication techniques are employed for these ceramics, including sintering, injection molding, and CAD/CAM technology [9].
Due to the advances in adhesive techniques, endocrowns are now utilized as restorations for severely compromised posterior teeth, particularly in cases involving short and narrow roots, blocked canals, and insufficient occlusal space. Some studies have also suggested the possibility of using them for anterior teeth. One of the key advantages of this technique is its conservative approach, which requires the removal of minimal dental tissue compared to traditional cores and posts, while also minimizing intrusion into the canals and less time required [9].
Despite the growing popularity of endocrowns, the question remains whether practitioners should consider this technique a reliable alternative to conventional cores, posts, and crowns, particularly for anterior teeth. In fact, survey studies have indicated that there is still hesitance among practitioners to use this technique on anterior teeth, largely due to the lack of monitoring studies [11].
This laboratory study aims to compare the fracture resistance of compromised and endodontically treated upper incisors restored using the endocrown technique versus the traditional technique of crowns supported by posts and cores.
2. MATERIALS AND METHODS
This study was approved by the ethics committee (ethical permission No. 2379 on 25-10-2021) at Tishreen University, Faculty of Dentistry, and was conducted in accordance with the Declaration of Helsinki for human studies.
2.2. Sample Size
Based on a previous study [12] and utilizing G*Power 3.1 software, the sample size was calculated using a one-way ANOVA test with a power of 0.85 at an alpha level of 0.05, resulting in a required sample size of 20 teeth. The research sample consisted of 20 upper incisors that were free from cracks and caries and extracted for supportive reasons.
The selected teeth were of similar size and shape, measuring 23 ± 1 mm in length, with a mesiodistal crown diameter of 9 ± 0.5 mm and a buccolingual crown diameter of 7 ± 0.5 mm. The process of collecting the teeth took approximately 20 days.
The teeth were cleaned using hand scalers and then brushed with toothpaste before being stored in physiological saline until testing. The sample was allowed to accumulate completely. After identifying the cemento-enamel junction for all teeth, 2 mm of the coronal portion was resected above the junction using a straight handpiece and a 22 mm diameter stainless steel laboratory separating disc.
All measurements and manual tasks (including the preparation and cementation stages) were performed by a single operator (AK). All teeth underwent endodontic treatment using a mechanical preparation device (Eighteeth E-Connect endo motor, Changzhou Sifary, China). The canals were irrigated with a 5.25% sodium hypochlorite solution (Canox, Syria) after each instrument.
Once the preparation was completed, the canals were dried using paper points. They were then filled using the lateral condensation technique with gutta-percha points of taper 0.04 and size #25 (Pearl Endopia, South Korea), along with an eugenol-free sealer (Adseal Meta Biomed, South Korea) to eliminate the potential effects of eugenol on resin polymerization in subsequent stages.
The teeth were then placed into standardized plastic molds, which were filled with self-curing acrylic and positioned 1 mm away from the anatomic neck. The teeth were randomly distributed into two groups:
2.3. Preparation of the Abutments
2.3.1. Preparation of Teeth in Group One
After removing the excess gutta-percha around the entry of the pulp chamber, the pulp canals in group one were prepared to a depth of 3 mm inside the canal, ensuring that at least 1 mm of dental tissue remained surrounding the preparation (Fig. 1A-E).
2.3.2. Preparation of Teeth in Group Two
These teeth were prepared to receive full ceramic crowns made from IPS Emax press ceramic. The preparation was performed using a rounded head tapered bur (DiAMANT, Germany, 199X016 FG) under water cooling to achieve a cervical ferrule height of 2 mm and a finish line width of 1 mm at the cemento enamel junction, with the bur being replaced after every 5 uses. The width of the finish line was confirmed using a calibrated periodontal probe.
The canal was then prepared using a bur specifically designed to match the diameter of the post, leaving 4-5 mm in the apical area. The teeth in this group were restored using quartz fiber posts (Quartz Fiber Post Resin Post, RTT, China).
First, the canal was irrigated with 5.25% sodium hypochlorite (Canox, Syria) and then dried using an oil-free air stream. The self-adhesive dual-cure resin cement (Breeze Dual-Cure Self Adhesive Resin Cement, Lot 8725242, Pentron, USA) was mixed according to the manufacturer's instructions, applied to the post, and subsequently inserted into the canal.
Excess material was removed using a bonding brush, and the resin cement was cured for one minute from the buccal, lingual, and occlusal sides using a light-curing device (LED Light Curing, Medical Hemao, China) with an output exceeding 1000 mW/cm2.
After the post was bonded, the procedure for building the resin core using composite material (Tetric N-Ceram, Lot 23503, Ivoclar Vivadent, Liechtenstein) was initiated. The dentin was roughened with 37% phosphoric acid (Eco-Etch 37%, Ivoclar Vivadent, Liechtenstein) for 15 seconds, followed by rinsing and drying with an air stream.
The dentin bonding agent (Tetric N-Bond Universal, W08416, Ivoclar Vivadent) was then applied and cured for 10 seconds. Using a soft material filling instrument, the composite was applied in layers, with each individual layer not exceeding 1.5-2 mm. Each layer was cured separately for 40 seconds using the same curing device.
After completing the core build-up, the preparation was finished, and any excess areas were removed to ensure continuity between the core material and dental tissues, using a rounded-head tapered bur (Fig. 2A-B).
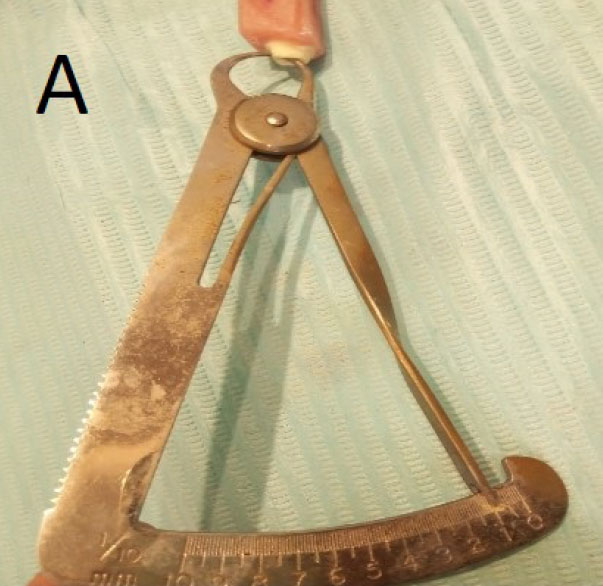
The remaining dentin thickness around the preparation in one of the abutments from group one.
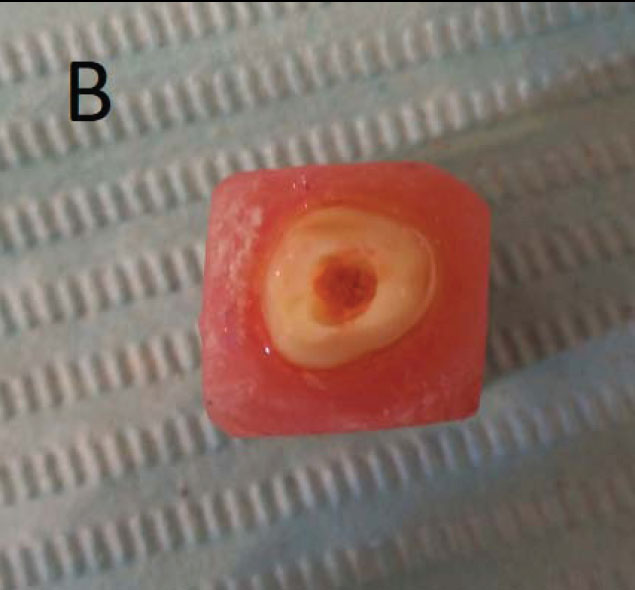
Occlusal view of the preparation in one of the abutments from group on.
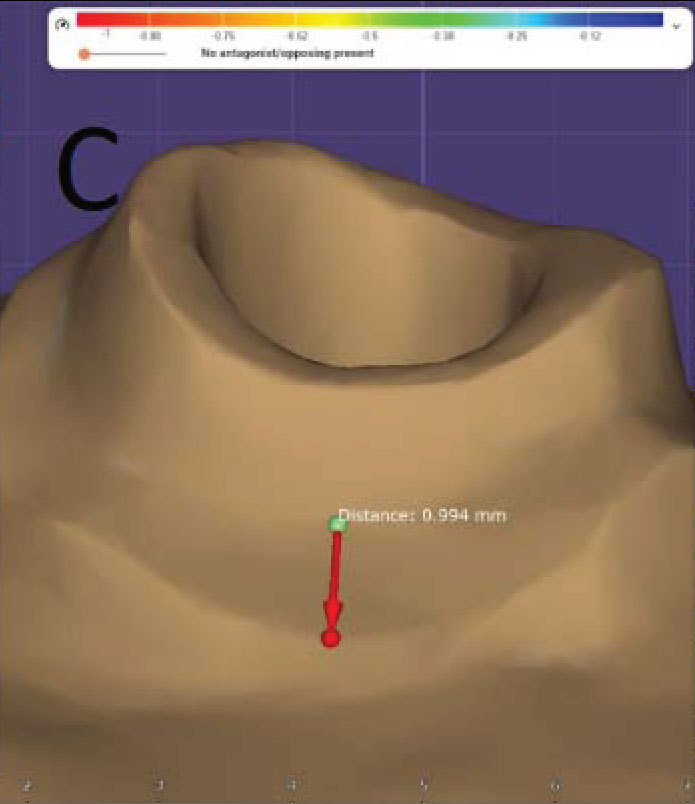
Display of the finish line in one of the abutments from group one after scanning with the milling scanner.
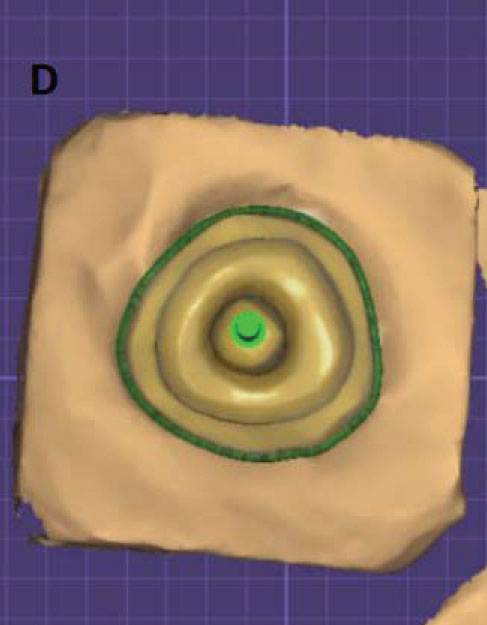
Occlusal view after scanning.
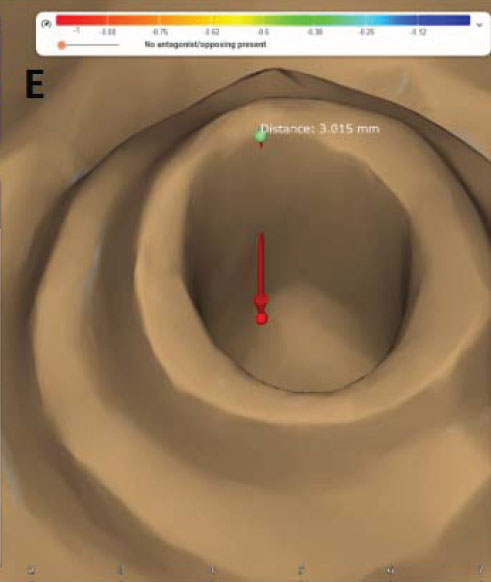
Measurement of the preparation depth inside the pulp canal after scanning.
Upon completion of the preparation of these abutments, the following characteristics were achieved:
- Cervical ferrule height: 2 mm
- Shoulder width: 1 mm
- Composite core height (measured from the finish line to the incisal edge): 7 mm
- Maximum mesiodistal width of the composite core: 7 mm
- Maximum buccolingual width of the composite core: 5 mm
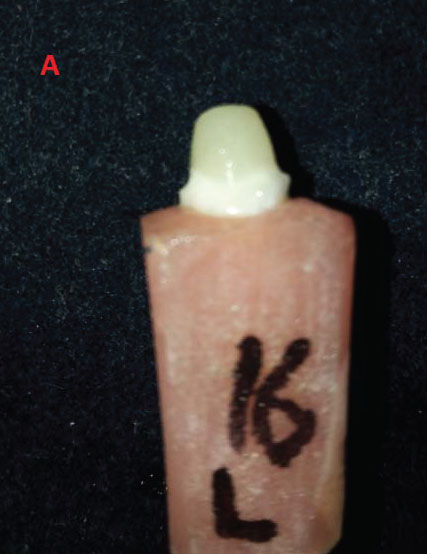
One of the abutments from group two.
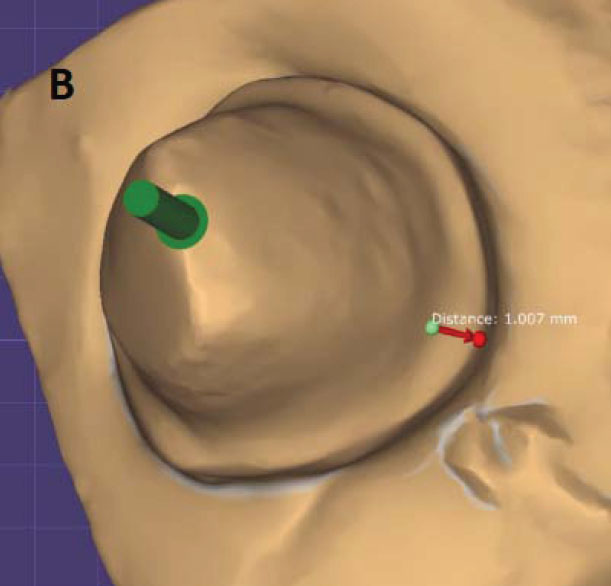
Occlusal view and measurement of the finish line width of one of the abutments from group two after scanning with the optical scanner.
After completing the preparation of the teeth in both groups, impressions were taken using a plastic tray with the double impression technique in one step, utilizing both hard and soft conditioning rubbers (Zetaplus, Putty and Light Body; Oranwash L, Zhermack, Italy). The teeth were stored in saline for 3 days until laboratory work was performed.
Subsequently, the impressions were poured using improved stone gypsum (Marmorock, Siladent), and the cast impressions were sent to the lab to complete the procedures. The gypsum models were scanned with an optical scanner from DGSHAPE, and the 3D models were processed on a computer using the software (exocad, DentalDB, version 2016).
The dimensions of the wax models for both groups were designed using the aforementioned software, resulting in:
- Incisal gingival height measured from the finish line: 10.5 mm
- Maximum mesiodistal width: 9.2 mm
- Maximum buccolingual width: 7 mm
A distance of 40 microns was maintained between the restoration and the tooth walls using the software (exocad, DentalDB, version 2016) (Fig. 3A-D).
Then, a wax disc (VeriCORE Mill Wax) was milled using a dry milling machine (ROLAND® DGSHAPE DWX-52DCI 5-AXIS Multi-Disc Dry Dental Mill), ensuring a 40-micron gap for the cement between the wax and the tooth walls. The resulting wax models were uniformly dimensioned.
The edges were sealed using casting wax (Renfert), and wax posts (Renfert) with a diameter of 3 mm were positioned according to the manufacturer’s instructions. Final compensating wax was then applied with the posts on the base of the injection crucible (IPS investment ring base, Ivoclar Vivadent) (Fig. 4A-D).
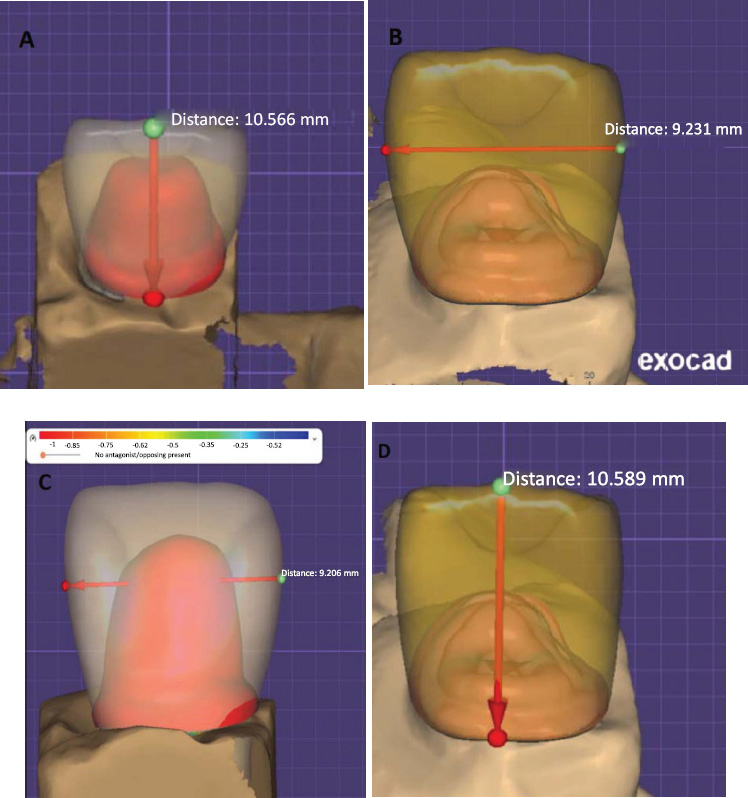
Measurements of the wax models before milling.
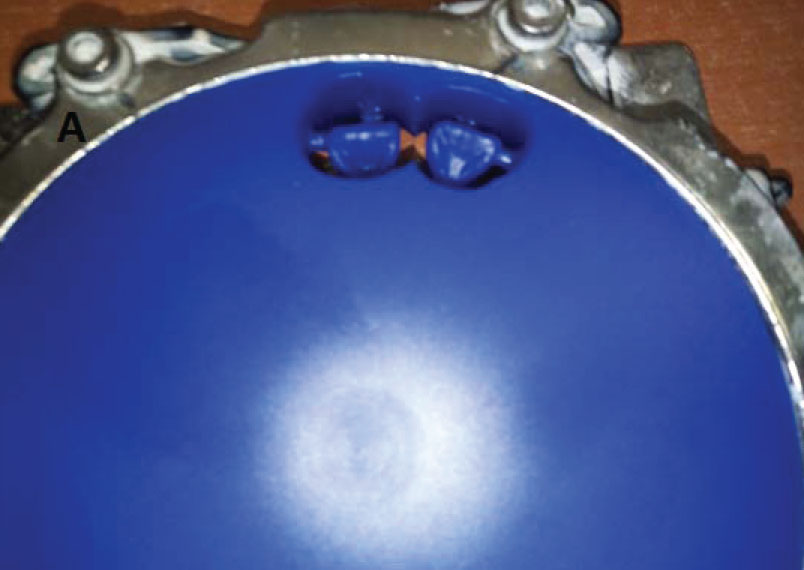
Wax disc milling.
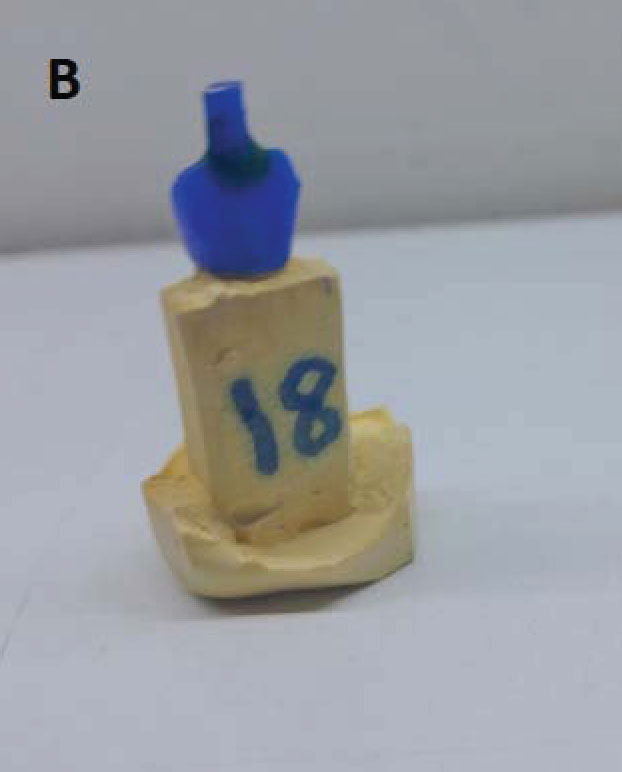
Wax model anchoring.
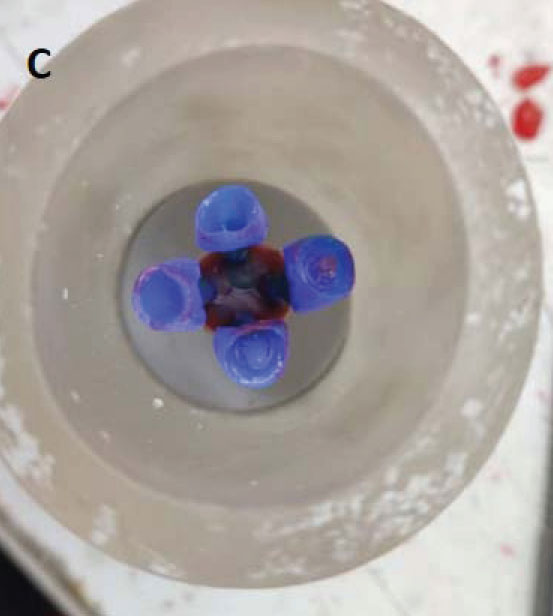
Final waxing with pins on the injection crucible base.
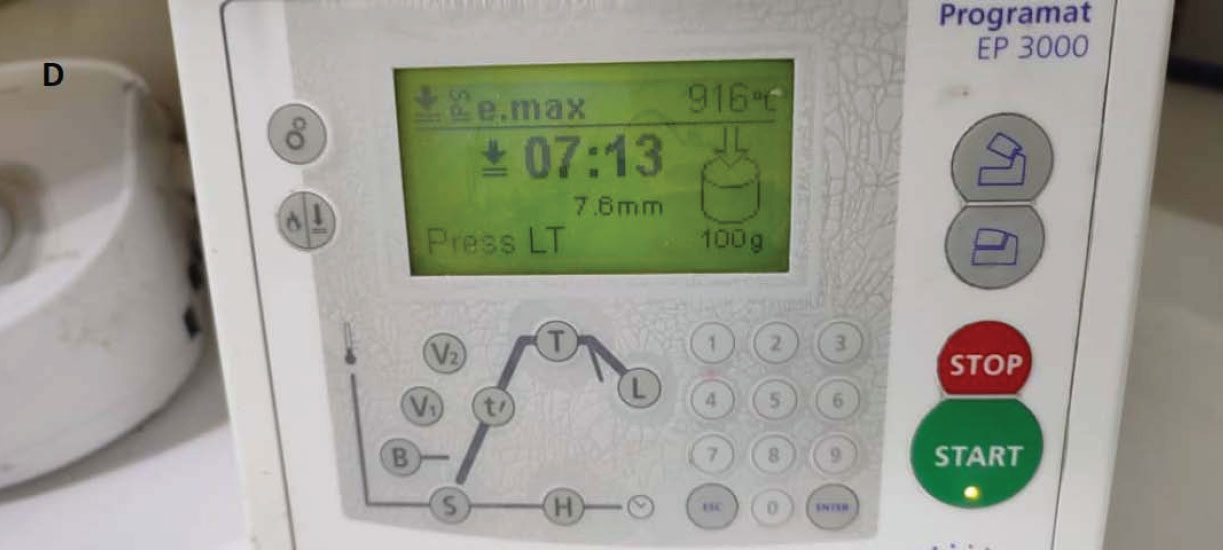
Setting up the thermal injection device.
The injection crucible was positioned, and the casing process was carried out using casting powder (IPS Press Vest, Ivoclar Vivadent). The crucible was then placed in the heating furnace, with the temperature set to 850 degrees Celsius for 45 minutes. Following this, the ceramic mold and aluminum plunger were placed inside the thermal ceramic injection furnace (Programat EP 3000, Ivoclar Vivadent).
Injection was then performed according to the manufacturer's recommended injection program. The crucible was allowed to cool at room temperature, and the ceramic models were cleaned of casting powder using a disc, followed by polishing with rubber spherical sand particles sized at 50 microns under 4 bar pressure.
The restorations were then immersed in a diluent solution of hydrofluoric acid (IPS e.max press invex liquid, Ivoclar Vivadent) at a concentration of less than 1% for 20 minutes, followed by polishing with rubber sand particles. The restorations were rinsed with an air-water stream, and the injection posts were cut using a diamond saw. The restorations were then trimmed using carborundum burs.
Finally, the external surface of the restorations was coated with glazing material (IPS e.max cream glaze powder and stain liquid, Ivoclar Vivadent). The powder and liquid were mixed according to the manufacturer's instructions before being applied to the external surface of the restorations. The restorations were then fired and allowed to cool at room temperature (Fig. 4A-D)
2.4. Cementation
The internal and marginal fit of the restorations was examined, and the internal surfaces of the restorations were subsequently treated. First, the porcelain restorations were cleaned with alcohol, rinsed with a stream of water and air, and then dried with air. The inner surfaces of the restorations, along with the recessed area in the pulp canal, were etched using 9.5% hydrofluoric acid (Porcelain Etchant 9.5%, lot 220005167, Bisco, USA) for 90 seconds. Subsequently, they were thoroughly washed with running water for 30 seconds and dried with an air stream. Then, two layers of the silane coupling agent (Porcelain Primer, lot 220004029, Bisco, USA) were applied and allowed to dry for one minute.
2.4.1. Preparation of the Abutment Surfaces
The surfaces of the prepared abutments were cleaned using pumice powder and a brush, then rinsed with a stream of water and air. Next, the abutments were etched with 37% phosphoric acid (lot T38390, Ivoclar Vivadent, Liechtenstein) for 15 seconds. Afterward, they were rinsed with running water and dried with air for 20 seconds. Next, the bonding agent (Tetric N-Bond Universal, lot W08416, Ivoclar Vivadent, Liechtenstein) was applied and gently blown with an air stream to ensure an even coat, then cured for 10 seconds. Afterward, the restorations were bonded using dual-cured resin cement (Metacem, Meta Biomed, Germany).
The base and accelerator were mixed in a 1:1 ratio, and the cement was applied to the internal surfaces of the restorations. The restorations were then placed onto their abutments with slight finger pressure, and any excess material was removed. The cement was subsequently cured using the previously mentioned light-curing device from the vestibular, lingual, mesial, and distal aspects for one minute (Fig. 5A-G).
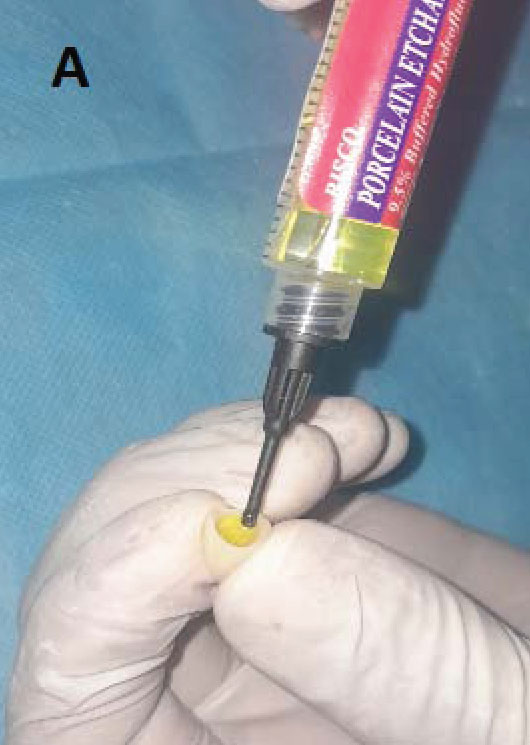
Etching the internal surfaces of the restorations with 9.5% hydrofluoric acid.
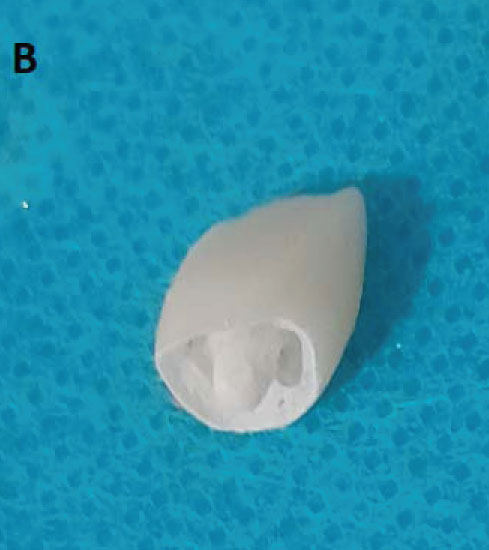
The chalky appearance of the porcelain after etching.
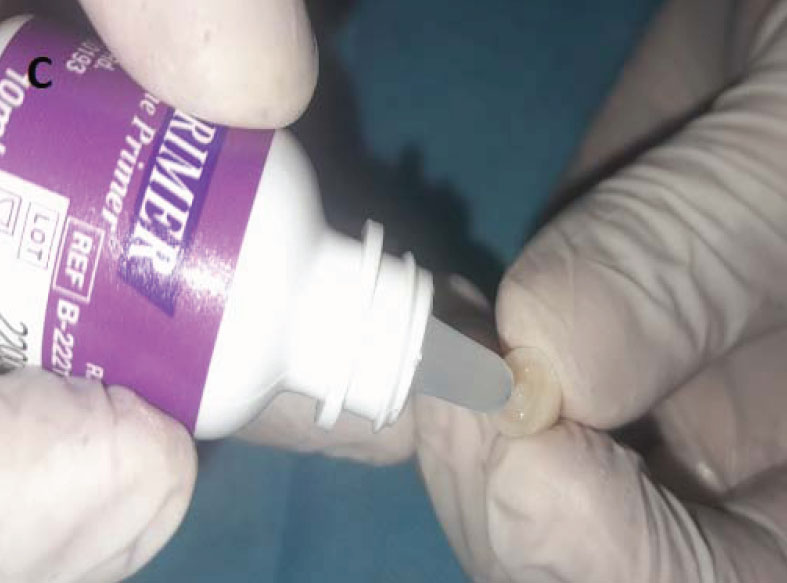
Application of the dual bond agent (silane).
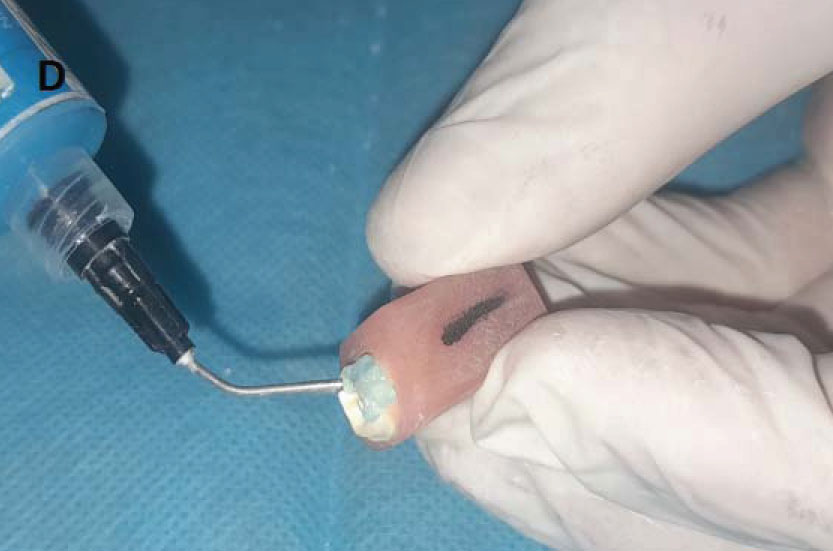
Etching the abutments with 37% phosphoric acid.
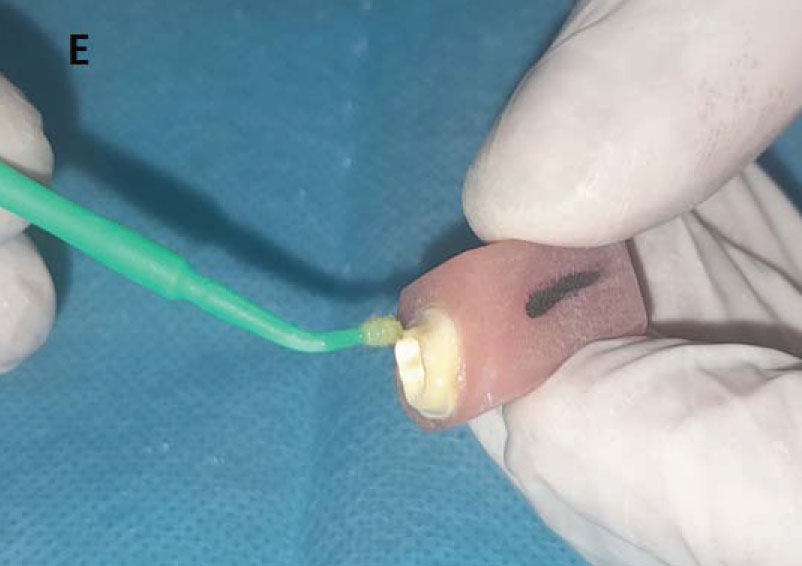
Applying bond.
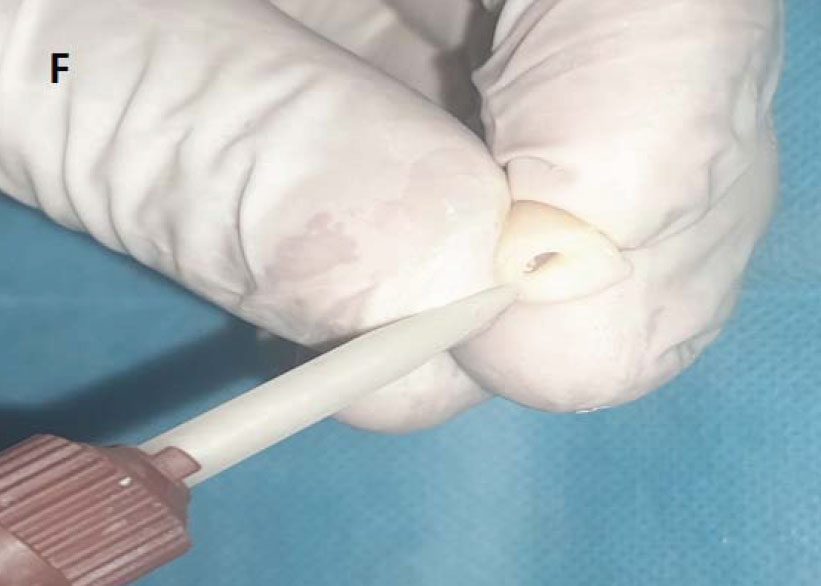
Application of the dual-cure resin cement.
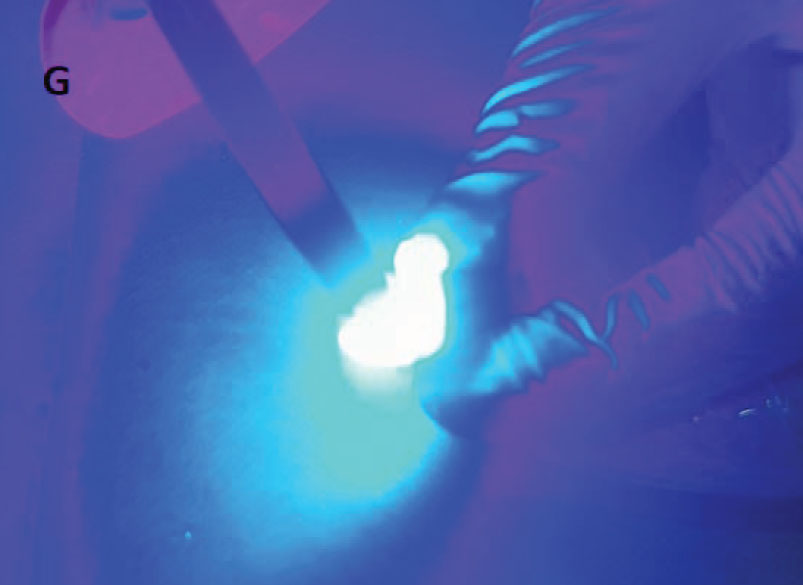
Curing the cement using a light-curing device.
A comparison of both groups is presented in Fig. (6). Upon completion of the bonding procedures, the specimens were stored in physiological saline until mechanical testing could be performed (Fig. 7A-B).
Mechanical tests were conducted under constant pressure using a universal testing machine available at the Mechanical Engineering Faculty of the University of Damascus. A custom base was designed to hold the teeth at a 45-degree angle. Forces were applied to the middle third of the palatal surface of the incisors fixed to the base, utilizing a knife-edge-shaped head with a width of 4 mm (Fig. 8A-B).
3. RESULTS
3.1. Sample Description
The research sample consisted of 20 healthy, non-carious root canines, which were divided into the following two equal main groups based on the restorative technique used:
3.1.1. Group One
Endocrown restoration made from IPS Emax press ceramic, with a depth of 3 mm inside the canal.
3.1.2. Group Two
Quartz-reinforced resin post, composite core, and IPS Emax press ceramic crown.
These groups serve as the basis for studying the effect of restorative techniques on the fracture resistance of teeth (Table 1).
Compensatory Technique Used | Number of Samples | Mean | Standard Deviation | Standard Error | Minimum | Maximum |
---|---|---|---|---|---|---|
Endocrown compensation made from emax ceramic at a depth of 3 mm inside the canal | 10 | 383.00 | 68.65 | 21.71 | 300 | 540 |
Quartz fiber-reinforced post with composite core and emax ceramic crown | 10 | 333.00 | 55.79 | 17.64 | 260 | 425 |
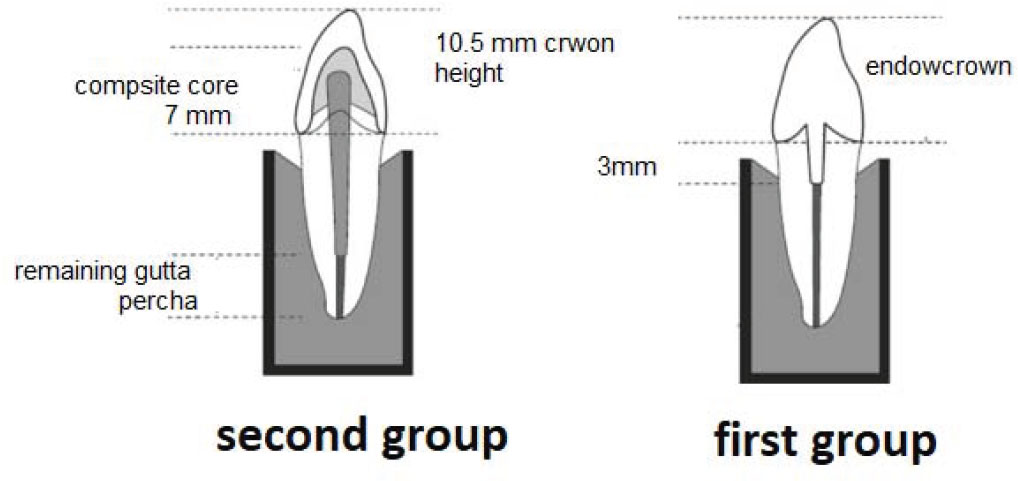
Illustrates the final compound of both study groups.
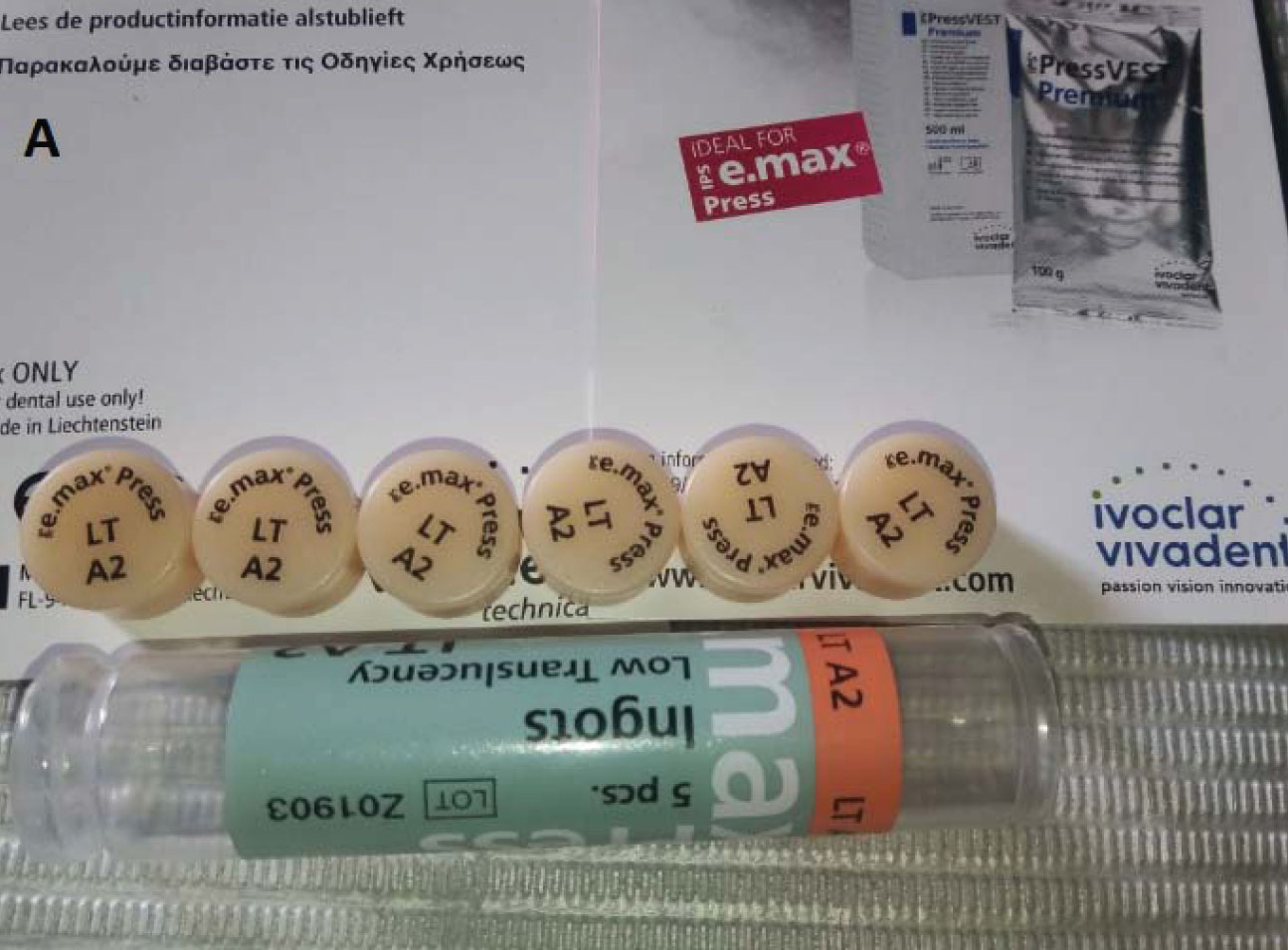
IPS e.max ceramic press ingots.
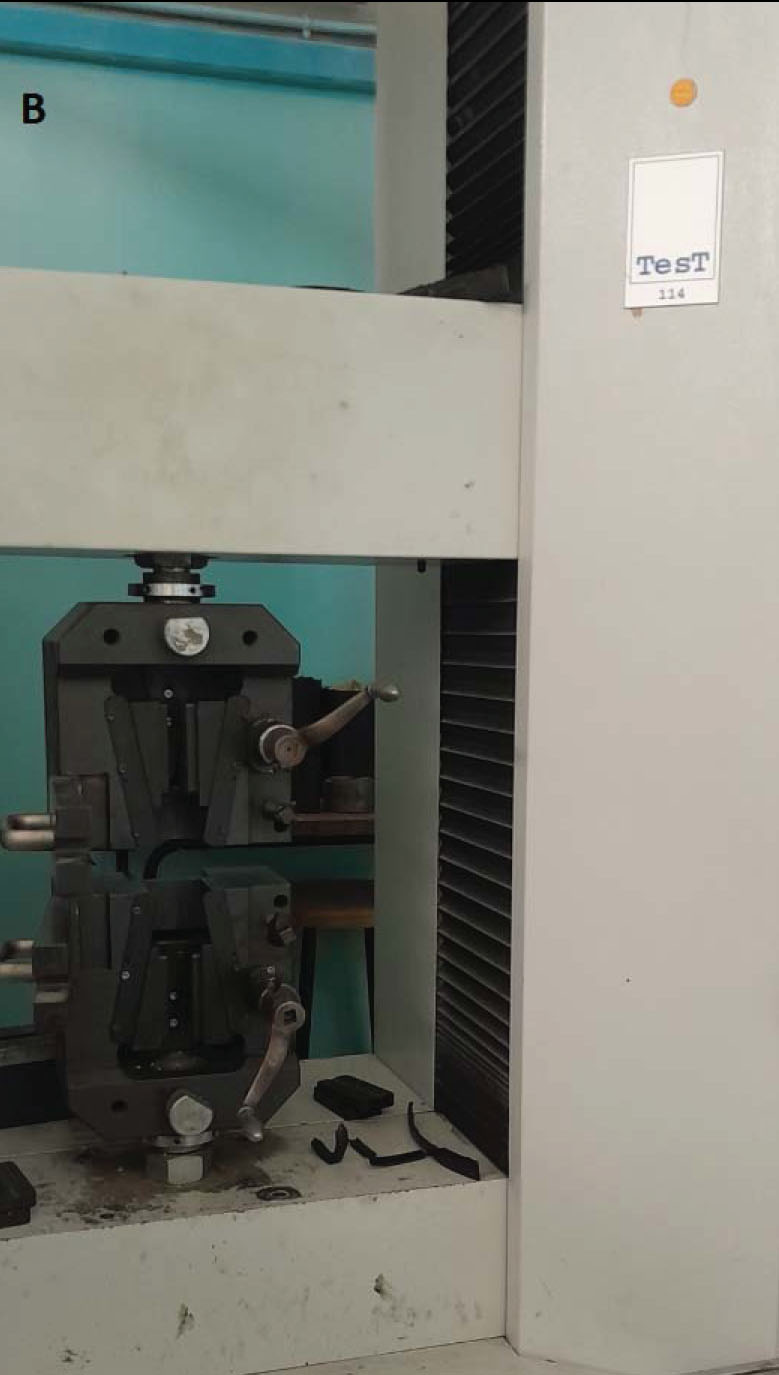
Universal testing machine.
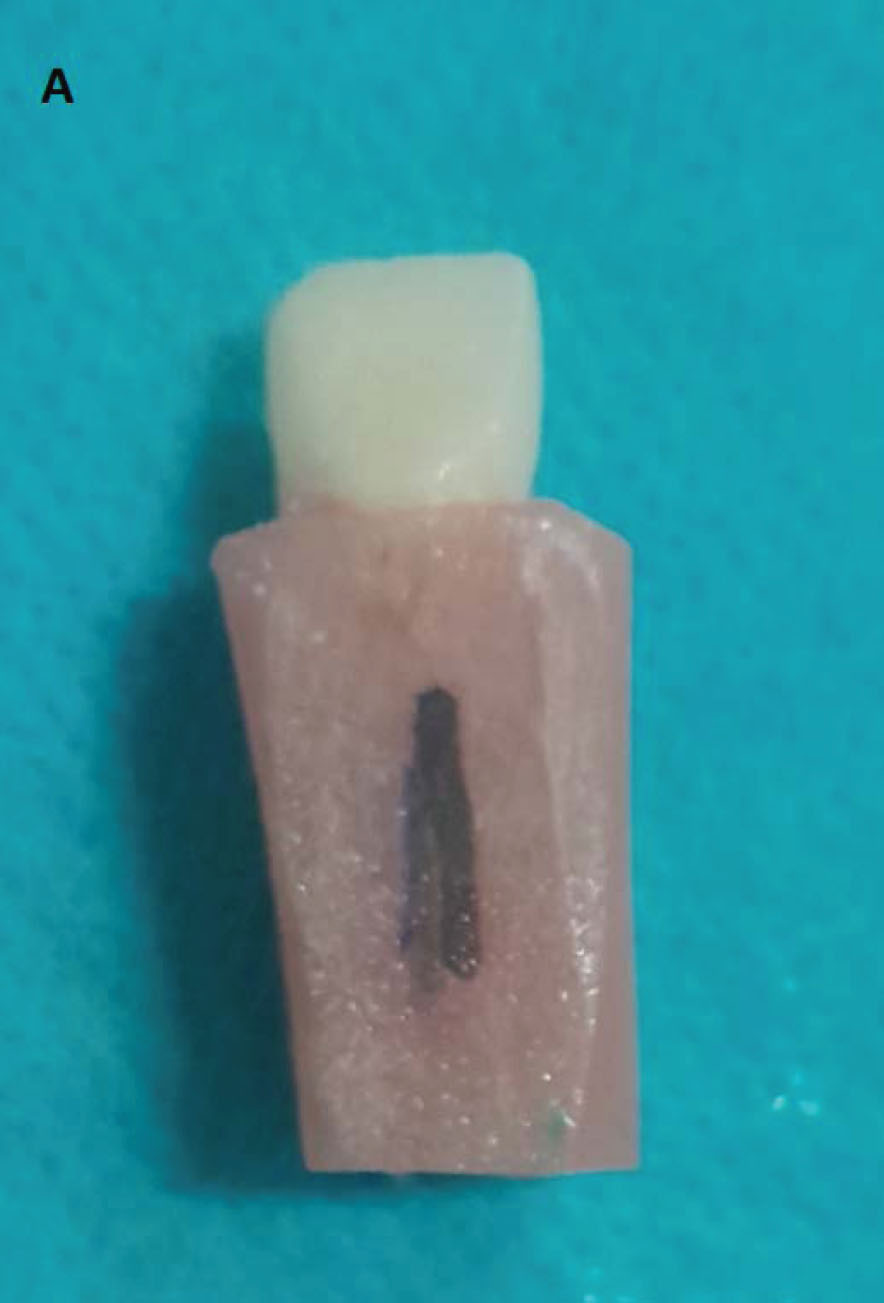
The final restoration after bonding.
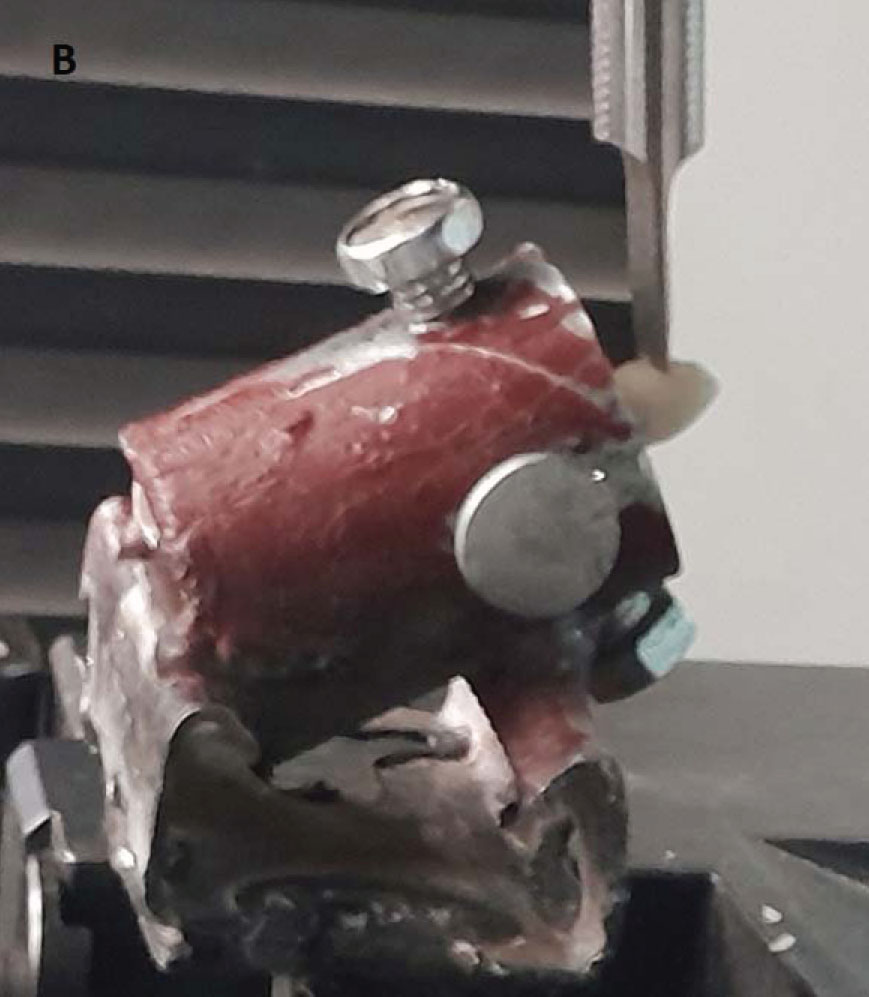
Applying oblique forces to the teeth at a 45-degree angle on a custom-made base.
Restorative Technique Used | Number of Canines | Percentage |
---|---|---|
Endocrown restoration made from e.max ceramic at a depth of 3 mm inside the canal | 10 | 50.0% |
Quartz-reinforced resin post, composite core, and e.max ceramic crown | 10 | 50.0% |
Total | 20 | 100% |
Difference Between Means | Calculated t Value | Significance Level | Significance of Differences |
---|---|---|---|
50.00 | 1.787 | 0.091 | No Significant Differences |
3.2. Study of the Effect of Restorative Techniques on Fracture Resistance Values
A students' t-test for independent samples was conducted to assess the significance of the differences in average fracture resistance values (in Newton's) between the endocrown compensation group made from IPS Emax press ceramic at a depth of 3 mm inside the canal and the group with a quartz fiber-reinforced post and composite core topped with an Emax ceramic crown. The results for the sample are as follows:
3.3. Descriptive Statistics
Table 2 presents the mean, standard deviation, standard error, minimum, and maximum values of fracture resistance (in Newtons) in the sample according to the compensatory technique used.
3.4. Results of the Independent Samples T-test
Table 3 presents the results of the independent samples t-test, which examines the significance of the differences in average fracture resistance values (in Newtons) between the groups based on the restorative techniques applied in the sample.
3.5. Studied Variable: Fracture Resistance (in Newtons)
The table above indicates that the significance level is greater than 0.05. This suggests that, at a 95% confidence level, there were no statistically significant differences in the average fracture resistance values (in Newtons) between the endocrown compensation group made from e.max ceramic at a depth of 3 mm inside the canal and the group with the quartz fiber-reinforced post, composite core, and e.max ceramic crown in the research sample.
4. DISCUSSION
This study closely mimics the biomechanical conditions of compromised upper incisors treated endodontically. Human-extracted teeth were utilized as a foundational basis for this research, enabling the simulation of these teeth as abutments while taking into account their morphological characteristics. Additionally, these teeth were chosen for their similarity in shape and size to minimize errors and variations as much as possible. The teeth were stored in saline for 20 days prior to testing, a duration that did not affect mechanical properties, such as hardness [13].
The selection of upper incisors in this study is significant due to their aesthetic and restorative importance in a patient's oral cavity, coupled with the limited number of studies addressing the use of the endocrown technique for upper incisors [7]. Traditional restorative treatments for compromised teeth often require substantial removal of dental tissue during crown and post-preparation procedures. This can complicate retreatment in the event of endodontic failure, as it increases the risk of root fracture, particularly in single-canal teeth [14].
Additionally, we frequently encounter restorative cases where there is insufficient vertical dimension to accommodate a post and traditional crown [15]. This context gave rise to the idea of using the endocrown technique for compromised upper incisors. While the use of posts in restoring compromised teeth can enhance their resistance to fracture, the preparation of the canal to accept the post may weaken the walls and increase the risk of potential root fractures [16].
The endocrown is a conservative and aesthetic restoration that typically requires lower costs and less clinical time compared to traditional techniques. Additionally, it provides an effective seal for the root canals, thereby reducing bacterial infiltration and improving the long-term prognosis of endodontically treated teeth. In cases of endodontic failure, retreatment is also more straightforward with endocrown restorations.
Moreover, the endocrown exhibited greater stability, increased resistance, and reduced susceptibility to fracture, as it minimizes stress levels applied to the dentin when compared to glass fiber and metal posts [16].
A depth of 3 mm was selected for endocrown preparation in the first group, as this represents a conservative approach aimed at preserving dental tissues. A previous study confirmed that there are no significant differences in fracture resistance between endocrown restorations made from e.max ceramic prepared at depths of 3 mm and 6 mm [17]. The roots were prepared to accommodate fiber posts at a depth estimated to be two-thirds the length of the canal, as supported by several prior studies [18].
The IPS e.max ceramic (lithium disilicate glass-ceramic), developed by Ivoclar Vivadent in 2005, was chosen for its superior mechanical and aesthetic properties compared to earlier systems [19]. The dimensions of the composite core in the second group were selected to adhere to the manufacturer’s recommended minimum thickness for IPS e.max press ceramics. A shoulder width of approximately 1 mm was established in both groups, following the manufacturer’s guidelines for the effective distribution of occlusal stresses.
This research is significant as it is the only study at the university level in the country to employ the endocrown technique for upper incisors. The CAD/CAM technique was utilized for wax milling to ensure dimensional accuracy, offering a high degree of precision in modeling and creating restorations [20]. Given the small and precisely dimensioned shape prepared within the chamber, the lost wax technique was adopted to standardize the manufacturing process, as it is the most commonly used method in our country.
The teeth were finalized in acrylic molds, as the elastic modulus of acrylic is comparable to that of alveolar bone [21]. The bases were designed to fit onto the specifically designated platform of the mechanical testing apparatus. No periodontal ligament simulator material was utilized, given that previous studies have indicated that this material does not influence the test results [21].
4.1. Fracture Resistance Testing
The experimental sample was designed to compare the endocrown technique made from e.max ceramic with traditional crowns constructed from IPS e.max press ceramic, supported by a composite core and fiber post. A universal mechanical testing machine was utilized for the tests, applying forces at a 45-degree angle to the palatal surface of the incisors, positioned 4 mm below the incisal edge. It is well-established that fracture resistance is influenced by the angle of force application, with oblique forces presenting a greater risk than vertical ones [22]. Therefore, a 45-degree angle relative to the longitudinal axis of the tooth on the palatal surface was selected.
The results of this experiment indicated that the forces responsible for failure in the endocrown technique were 383 ± 68.65 N, which is greater than the physiological forces naturally present in the upper incisor region, i.e., 138 Newtons in adult males and 97 Newtons in adult females [23]. Additionally, this force exceeded the fracture resistance of natural incisors, which is 261.5 Newtons [24]. These values were also higher than those observed in the group of porcelain crowns, which recorded a force of 333 ± 55.79 N.
This superior fracture resistance may be attributed to the fixed design of the endocrown and the greater thickness of the IPS e.max press ceramic, both of which contribute to enhanced fracture resistance. However, these differences were not statistically significant, with a p-value of 0.09.
Several previous studies investigated fracture resistance in compromised teeth treated endodontically and restored with e.max endocrowns, finding this technique to be a viable alternative to traditional methods. For instance, a study by Forberger and Göhring (2008) reported no statistically significant differences in fracture resistance between the endocrown and traditional methods in lower premolars [25].
Meanwhile, a study by Ramin Atash et al. in 2017 [26] demonstrated that the endocrown offers higher fracture resistance than the traditional method when used for lower premolar restorations. This difference may be attributed to variations in the speed of force application. A study by Atash utilized a speed of 1 mm/min, while our study employed a speed of 0.5 mm/min. Differences in tooth type (premolars) may have played a role.
Additionally, Biacchi et al. (2012) found that the endocrown technique provides higher fracture resistance when compared to traditional methods for lower molar restoration [27]. Conversely, Bankoğlu et al. (2017) found that the e.max endocrown demonstrated higher fracture resistance in upper incisors compared to the traditional technique [28], which may be due to the use of IPS e.max CAD while we used IPS e.max press in this study.
CONCLUSION
It was found that the endocrown technique offers greater fracture resistance compared to traditional methods for lower molar restorations. Conversely, several other studies reported that the e.max endocrown exhibited higher fracture resistance in upper incisors compared to the traditional technique. This discrepancy may be attributed to the use of IPS e.max CAD in their study, while we utilized IPS e.max press in our research.
AUTHORS’ CONTRIBUTION
A.K., R.S.: Study conception and design; A.K. and R.S.: Data collection; A.K. and R.S.: Analysis and interpretation of results; A.K., R.S., N.B., and Z.A.: Draft manuscript. All authors reviewed the results and approved the final version of the manuscript.
ETHICS APPROVAL AND CONSENT TO PARTICIPATE
This study was approved by the ethics committee (ethical permission No. 2379 on 25-10-2021) at Tishreen University, Faculty of Dentistry, Lattakia, Syrian Arab Republic.
HUMAN AND ANIMAL RIGHTS
All human research procedures followed were in accordance with the ethical standards of the committee responsible for human experimentation (institutional and national), and with the Helsinki Declaration of 1975, as revised in 2013.
AVAILABILITY OF DATA AND MATERIALS
All corresponding data used to support the findings of this study were included in the article.
ACKNOWLEDGEMENTS
Declared none.