All published articles of this journal are available on ScienceDirect.
Methodological Stumbles in the Culture of Stem Cells from the Dental Pulp: An In vitro Study
Abstract
Background
Human dental pulp is a valuable source of multipotent stem cells with considerable regenerative cell potential. The protocol for isolating dental pulp stem cells involves extracting healthy teeth, dissecting the pulp, enzymatically digesting it with collagenase, and culturing the cells in a specialized medium. Cell growth is monitored using microscopy and staining to assess viability and contamination.
Objective
This study aimed to describe methodological complications in culturing dental pulp stem cells.
Material and Methods
A sample of eight healthy third molars were extracted: group 1 (n=4) included molars, group 2 (n=3) comprised partially erupted molars, and group 3 (n=1) included molars with pericoronitis. Extracted molars were dissected, and the pulp was enzymatically digested with collagenase placed in Dulbecco's modified Eagle's medium-low glucose culture medium, fetal bovine serum, porcine skin gelatin, reduced L-glutathione, penicillin-streptomycin, and amphotericin-B. Observation under inverted microscopy using a 40X lens and gram and trypan blue staining was performed.
Results
Undissolved particles were observed in the medium, possibly related to the addition of gelatin or L-glutathione at the start of the culture, negatively affecting cell growth and observation. In the initial days of the experiment, there were floating cells in groups 1 and 2, but no cells were found adhering to the container surfaces. In group 3, there was an absence of cells, and particles and undigested tissue remnants were observed. Gram staining revealed the presence of Gram-positive bacteria in groups 1 and 2, and trypan blue staining did not allow the observation of cells in the Neubauer chamber.
Conclusion
Common difficulties include issues related to medium manipulation, pH regulation, presence of undissolved particles, lack of cell adherence, bacterial contamination, and difficulty in cell reproduction. Therefore, standardization of protocols and careful selection of reagents used are necessary.
1. INTRODUCTION
Regenerative medicine is based on scientific advances concerning mesenchymal stem cells (MSC) and their ability to transform themselves into cells from different types of tissue [1]. These cells are multipotent, and they are found in most postnatal organs [2]. It is possible to obtain MSCs from different sources: bone marrow, blood from the umbilical cord, adipose tissue, pancreas, liver, skeletal muscle, dermis, and synovial membrane [3]. In the oral cavity, the MSCs are found in the dental pulp, periodontal ligament, dental follicle, gingival connective tissue, and other oral regions [4]. Cell diversity found in the dental pulp is due to the complexity of the development process, which indicates the potential of the dental pulp stem cells to become different types of cells: odontoblasts, osteoblasts, cementoblasts, chondrocytes, neural cells, myoblasts, endothelial cells, and adipocytes [5, 6]. Dental pulp stem cells (DPSCs) are found inside the teeth and contain a heterogeneous cell population represented by fibroblasts, endothelial cells, perivascular cells, neural cells, osteo-odonto progenitors, and inflammatory and immune cells [7, 8]. The DPSCs’ initial discovery was attributed to the innovative work of Gronthos et al. [9]. From an embryologist's point of view, the origin of these cells is located in the neural crest, which implies an ectodermal origin [10]. Moreover, their plastic adherence and clonogenic properties have been proven [9]. In the literature, there have been reported two fundamental methods for isolating DPSCs: (a) the explant method (DPSC-OG) and (b) the enzymatic dissociation of pulp tissue method (DPSC-ED). The explant method consists of the surgical extraction of pulp tissue, and cells are cultivated from tissue fragments. In contrast, the enzymatic dissociation method uses enzymes, like collagenase and dipase, which are used to digest the dental pulp releasing individual cells. Both methods aim to isolate DPSCs, but they differ in how the tissue is processed and the conditions under which cells are harvested [11-13]. Subsequently, cells are cultivated in culture plates, allowing them to multiply. During this process, the DPSCs are identified and classified using flow cytometry, which is based on the dying of specific markers for their examination [14, 15]. Currently, there are several isolation, expansion, and conservation protocols for DPSCs [12, 14-17]. Suchánek et al. proved that DPSCs managed to make 60 population duplicates in a culture medium designed for the bone marrow [18]. El Alami et al. described that culture cells at 3% O2 had a much lower oxidation level than those at 21% O2, i.e., DPSCs proliferation was higher in cultures with 3% O2 compared to those with 21% O2 [19]. In the DPSCs culture protocols, fetal bovine serum (FBS) is used, which complicates the experiment’s reproducibility, as the composition varies between the sets [20]. In this sense, the use of non-human supplements continues to be a subject of debate due to the risk of contamination with human pathogens, such as viruses, mycoplasma, prions, or other toxic or immunogenic agents [21]. There is limited evidence that low-level laser irradiation (LLLI) (660/810/980 nm with an energy density of 0.1-3 J/cm2) can increase Dental mesenchymal stem cells proliferation. However, due to the limited number of studies and methodological variability, further research is needed to determine the optimal LLLI parameters and assess their clinical feasibility [22]. The objective of this study was to evaluate the impact of different isolation protocols on the yield and viability of DPSCs derived from various tooth groups. By analyzing the methodological variations in these protocols, this research study aimed to determine how specific procedural differences influence the quality and quantity of DPSCs obtained. The study sought to answer the following research question: How do variations in isolation protocols affect the efficiency and characteristics of DPSCs harvested from different tooth types?
2. MATERIALS AND METHODS
This pilot in vitro study was conducted in accordance with the Declaration of Helsinki and approved by the Ethics Committee of Santiago-Lugo (CEI-SL) (protocol code 2023/484). It also followed STROBE standards.
2.1. Sample Selection
Third molars were collected from individuals of different ages and genders (19 – 21 years old) who did not exhibit any type of systemic pathology in a private clinic (ASA I patients): group 1 included molars (n=4), group 2 comprised partially erupted molars (n=3), and group 3 included molars with pericoronitis (n=1) [23]. All the teeth had closed apexes. Study objectives were thoroughly explained to the participating patients, who subsequently provided their informed consent by signing the required forms. The nomenclature for the groups is defined as follows: group 1 - pulp extracted from included third molars, group 1A - cells and suspended particles, group 1B - cells and adherent particles; group 2 - pulp extracted from partially erupted third molars, group 2A - cells and suspended particles, group 2A-1-cells and suspended particles, group 2A-2-cells and adherent particles, group 2B-cells and adherent particles; group 3-pulp extracted from third molars with pericoronitis. After the surgical extraction of teeth, they were kept in 50 ml conical tubes, and the means of transport was prepared, which was composed of 3 ml of trypsin-EDTA (Sigma-Aldrich, Madrid, Spain; ref.: T3924), 3 ml of penicillin-streptomycin (Sigma-Aldrich, Madrid, Spain; ref.: P0781), and 15 ml of Dulbecco's phosphate-buffered saline (PBS) D8537 (Sigma-Aldrich, Madrid, Spain; ref.: P0781) (Fig. 1).
2.2. Sample Preparation
Molar dissection was carried out using a class II type A2 biological biosafety cabinet (Thermo Scientific). A marathon electric motor (Handy 702 model) and a diamond-edged disc were also used. All instruments were sterilized prior to obtaining the sample. A longitudinal section was made under refrigeration of sterile distilled water at 4ºC, constantly dosed with hypodermic syringes; a disc and an individual sterile mandrel were used for each molar dissection. A superficial canal began to be formed by means of a cut in an occluso-proximal or buccal direction depending on the morphology of each molar, at a speed of 20 rpm with intermittent pressure under constant irrigation. After deepening 1 ml in the said groove, the motor speed was decreased to 15 rpm, with intermittent cuts and pressure, until it deepened approximately 1 additional mm. The groove was abundantly irrigated with the purpose of clearing or eliminating the remains of the smear layer accumulated in the groove. A leverage force was generated with a cement spatula or fine straight elevator (2mm 301), introducing the instruments through the carved channel. The pulp tissue was then removed with a sterile curette and transferred to a Petri dish with several cuts made with scissors. Three 50 ml conical tubes were prepared with 15 ml of low-dose Dulbecco's modified Eagle's medium (DMEM) obtained from Sigma Aldrich to place the dental pulps of the 3 groups. They were centrifuged at 1800 rpm for 5 minutes at 4 degrees and the supernatant was removed. We immersed that pellet in collagenase taken from 6 mg clostridium histolyticum
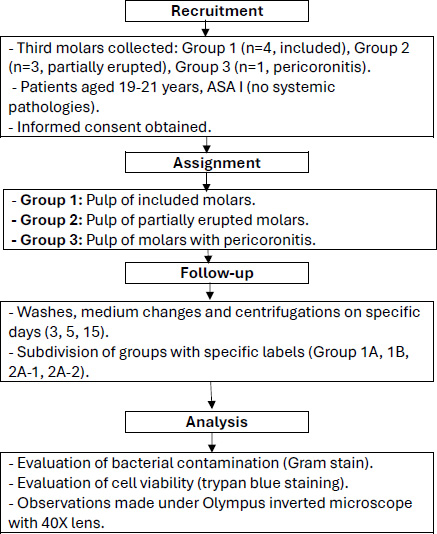
A summary of the study’s workflow.
C0130 (Sigma Aldrich) mixed with 6 ml Dulbecco's phosphate buffered saline (PBS D8537; Sigma Aldrich) in a water bath and stirring at 37 degrees continued for 45 minutes. Afterward, the supernatant was removed, and with DMEM FG0415 (Sigma Aldrich), it was placed at 37 degrees and passed through different filters of 100 um Falcon CS10 cell sterile strainer and 35 um cell strainers. Then it was immediately centrifuged at 1800 rpm for 10 minutes at 4 degrees. In the next step, the supernatant was removed and PBS D8537 was placed and centrifuged at 2000 rpm at 4 degrees for 5 minutes.
2.3. Initial Isolation Medium for Stem Cells
The initial isolation medium consisted of DMEM (Sigma-Aldrich, ref.: D6046), 10% FBS (Sigma-Aldrich, ref.: F244), 50 ug/ml pork skin jelly (Sigma Aldrich, ref.: G1890), 2mM reduced L-glutathione (Sigma-Aldrich, ref.: G6013), 10 mg/L penicillin-streptomycin (Sigma-Aldrich, ref.: P0781), and 2.5 ug/ml amphotericin-B (Sigma-Aldrich, ref.: A2942) [9, 11, 13].
2.4. Applied Treatments
2.4.1. Day 3
Groups 1 and 2: a wash was performed with PBS and the concentration of the antibiotic and antifungal was increased by 5% to the medium that was already in the cell culture bottle, T-25 (Sarstedt, Nümbrecht, Germany). Group 3: A wash was performed with PBS, the medium was changed, and the medium used was the initial isolation medium with an increase of 20% FBS.
2.4.2. Day 5
Group 1: from the T25 bottle, 10 ml of the cell culture was transferred to a sterile conical tube and centrifuged at 500 g for 5 minutes. Then the supernatant was removed and 5 ml of the initial isolation medium previously filtered with a 0.22 um syringe filter was added to the conical tube. The pH was adjusted to 7. The contents of the tube were homogenized by pipetting and 5 ml of them were subsequently transferred to a well of a sterile Costar brand plate (6-well cell culture cluster flat bottom). Group 2: from the T25 bottle containing 10 ml of medium with cells, 7 ml of the medium was transferred to a conical tube, which was followed by centrifugation at 500 g x 5 min. The supernatant was removed, and 5 ml of the previously filtered initial isolation medium was added to the conical tube using a 0.22 um syringe filter. The pH was adjusted to 7. The contents of the tube were homogenized by pipetting and 5 ml of them were subsequently transferred to a well of a sterile 6-well plate [9]. This well was labelled “group 2A” and the remaining 3 ml content of the T25 flask was transferred directly to a new well of the plate. This well was labelled “group 2B”. In group 3, no changes were made.
2.4.3. Day 15
Group 1: the non-adhered cells and particles contained in the medium of this well were transferred to a conical tube and centrifuged at 500 g for 5 min. The supernatant was removed, and the new filtered initial medium was added. The pH was adjusted to 7 and the supernatant was transferred to a new 6-well plate. This well was labelled “group 1A”. On the other hand, 5 ml of the initial isolation medium was added to the cells and particles adhered to the surface of the well. Afterward, the bottom of the well was scraped and the remaining content was transferred to a new well. This new well was labelled “group 1B”. Group 2A: the non-adhered cells and particles contained in the medium of the previous well were transferred to a conical tube and centrifuged at 500 g for 5 min. The supernatant was removed, and the new filtered initial medium was added. The pH was adjusted to 7 and the supernatant was transferred to a new 6-well plate, labelled “group 2A-1”. On the other hand, 5 ml of the initial isolation medium was added to the cells and the particles adhered to the surface of the well; the bottom of the well was scraped and the remaining content was transferred to a new well, labelled “group 2A-2”. Group 2B and group 3: no interventions were made in these groups.
3. RESULTS
The pH of the DMEM medium used for the isolation of mesenchymal cells was 8.26. Additionally, undissolved particles were observed in the medium with the naked eye, which could be due to the jelly or L-glutathione that was added at the beginning of the culture.
3.1. Day 3
The following observations were made using the inverted microscope with a 40X objective lens:
3.1.1. Group 1
Round cells and cells with irregular edges were observed. All these cells were floating in the medium. There were no fixed cells on the surface of the bottle. There were many small particles suspended, and others were fixed at the bottom of the bottle. Pieces of tissue that were not initially digested were also observed (Figs. 2A-C).
3.1.2. Group 2
Round cells and cells with irregular edges were observed floating in the medium. There were no fixed cells on the surface of the bottle. The presence of small translucent spherical structures was noted. Small particles in a large number were suspended, and others were fixed at the bottom of the bottle (Figs. 2D-F).
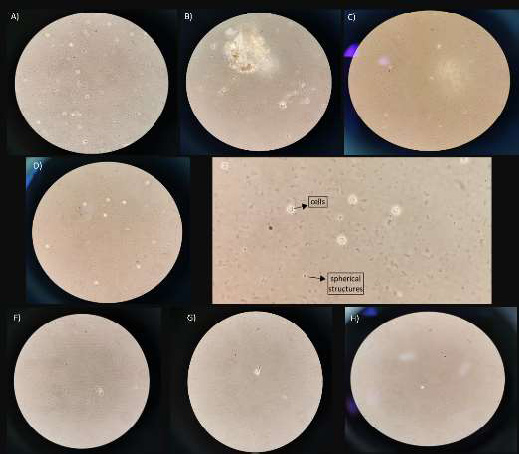
Day 3. A, B, and C belong to group 1; D, E, and F belong to group 2, and G, H belong to group 3.
3.2. Day 5
The following observations were made at this stage:
3.2.1. Group 1
Cells were observed with irregular edges, and a small number of cells were found to float in the medium. There were no fixed or adhered cells (Figs. 3A, B).
3.2.2. Group 2A
Cells with non-uniform contours could be seen. A reduced quantity was suspended in the medium, without fixed or adhered cells (Figs. 3C, D).
3.3. Day 10
The following observations were made at this stage:
3.3.1. Group 1
Some round cells and others with irregular edges were observed. Likewise, small particles and materials that were not digested were present in the bottle (Figs. 4A, B).
3.3.2. Group 2A
Rounder cells in a greater number were observed, as well as undigested particles (Figs. 4C, D).
3.3.3. Group 2B
A clear reproduction of cells was evident in the medium, with a rounder morphology. Some cells presented irregularities, but they were found in a higher quantity (Figs. 4E, F).
3.3.4. Group 3
Small particles in a large number were fixed at the bottom of the bottle. Pieces of tissue that were not initially digested were observed (Fig. 4G).
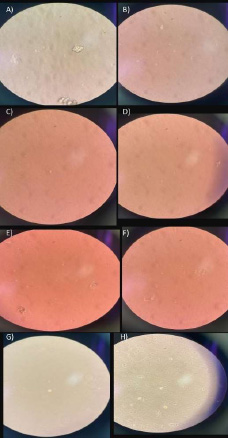
Day 5. A, B) group 1; C, D) group 2A; E, F) group 2B; G, H) group 3.
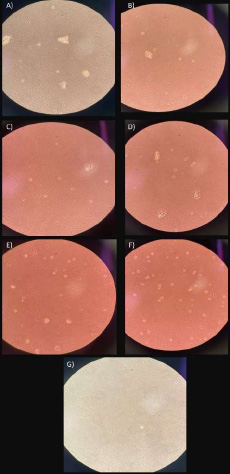
Day 10. A, B) group 1; C, D) group 2A; E, F) group 2B; G) group 3.
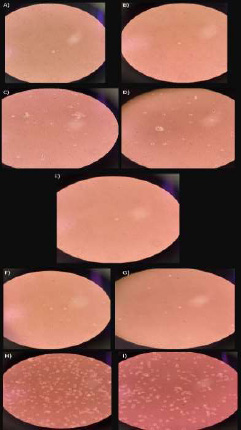
Day 16. A, B) group 1A; C, D) group 1B; E) group 2A-1; F, G) group 2A-2; H, I) group 2B.
3.4. Day 16
The following aspects were noted at this stage:
3.4.1. Group 1A
Only two round cells could be seen in the medium, without any reproduction (Figs. 5A, B).
3.4.2. Group 1B
Bacterial activity was observed in this medium sample, with few cells, which were irregular and with poor morphology, and several particles varied in size and shape (Figs. 5C, D).
3.4.4. Group 2A-2
Round cells and cells with irregular edges were observed floating in the medium. There were small particles suspended, and others were fixed at the bottom of the bottle (Figs. 5F, G).
3.4.5. Group 2B
Several round cells and cells with irregular edges, with some fixed and others floating, were observed. However, an important reproduction of the cells was evident in this sample. The presence of small translucent spherical structures was noted. There were also small particles observed (Figs. 5H, I).
3.5. Day 20
Gram staining results were obtained as follows:
3.5.1. Group 1A
No presence of bacteria was observed, but a large number of particles were with irregular shapes in the medium (Figs. 6A, B).
3.5.3. Group 2A-1
No presence of bacteria was observed, but a large number of particles were with irregular shapes in the medium (Fig. 6D).
3.6. Trypan Blue Staining Results
Trypan blue staining was performed by taking an aliquot of the following samples: group 1B, group 1A, group 2A-2, and group 2A-1. It was not possible to observe cells in the Neubauer chamber; therefore, cell viability could not be evaluated; this may be due to the low cell density in the wells. Only some conglomerate filamentous structures that were not stained blue were observed (Figs. 6F-H).
3.7. Day 22
The following observations were made at this stage:
3.7.1. Group 1A
A greater density of cells was observed in the center of the well, and towards the ends of the well, no cells were observed (Figs. 7A, B).
3.7.3. Group 2A-1
No cells were observed, and there was only the presence of particles with elongated morphology (Fig. 7D).
3.7.4. Group 2A-2
Particles with a rounded morphology were observed; they did not correspond to cells (Fig. 7E).
3.7.5. Group 2B
Particles with a rounded morphology were observed, and they did not correspond to cells (Fig. 7F).
3.7.6. Group 3
Particles of different sizes and morphology were observed; the exact type of particles found was unknown, and the presence of cells was ruled out (Fig. 7G).
4. DISCUSSION
It is essential to have a standardized protocol for the isolation and characterization of stem cells from the dental pulp, which can be able to guarantee transparency, reliability, and adequate interpretation of the results. However, it is widely recognized that negative results are not always disclosed, which could often lead to considerable bias in the results.
The protocol for isolating DPSCs described by S. Gronthos et al. involved the collection of impacted third molars, followed by the extraction of pulp tissue through incisions at the cementoenamel junction. The tissue was then enzymatically digested using type I collagenase and dispase, filtered through a 70 µm sieve to obtain single cells, and cultured in a medium enriched with α-MEM, 20% FBS, ascorbic acid-2-phosphate, L-glutamine, penicillin, and streptomycin [9, 24].
A more recent protocol used by Gronthos et al. for the isolation and expansion of DPSCs included the use of α-MEM supplemented with 10–20% FBS, 2 mM L-glutamine, 100 mM L-ascorbate-2-phosphate, 50 U/ml penicillin, 50 µg/ml streptomycin, and 5 × 10−5 M β-mercaptoethanol, providing an optimal medium for cell viability and proliferation [25].
On the other hand, Qiao X et al. developed a protocol to isolate and culture DPSCs and periodontal ligament stem cells (PDLSCs) from third molars of healthy donors. Both cell lines were cultured in α-MEM medium enriched with 10% FBS and antibiotics, maintaining the conditions at 37°C in a 5% CO2 atmosphere [26].
It was proved that tissue related to teeth, such as dental pulp and dental follicles, could represent an alternative source for mesenchymal stem cells [13, 27, 28]. Research showed that the extraction of third molars can generate sufficient dental pulp tissue to effectively isolate DPSCs [28, 29]. This is why obtaining the dental pulp has become a crucial point since it can lead to contamination if a correct protocol for the isolation of stem cells is not carried out.
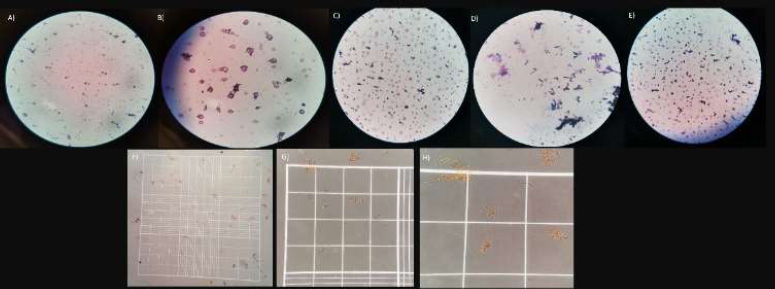
Gram staining. A, B) group 1A; C) group 1B; D) group 2A-1; E) group 2A-2; F, G, H) trypan blue staining.
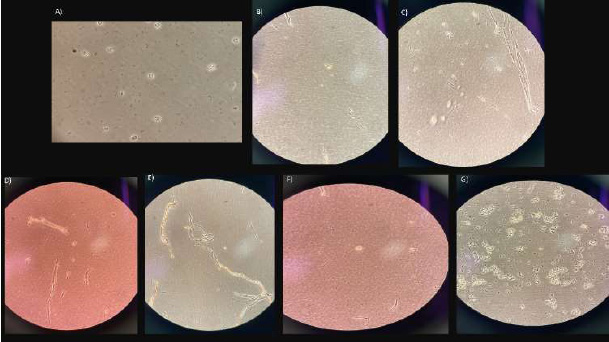
Day 22. A, B) group 1 A; C) group 1B; D) group 2A-1; E) group 2A-2; F) group 2B; G) group 3.
DPSCs could be obtained through the explant method or the enzymatic decomposition technique [30]. Ferrúa et al. [31], in a systematic review, described that 56.3% of the research studies have used the enzymatic technique, followed by 33.5%, which have used enzymatic and mechanical techniques. The least frequent have been found to be explant techniques with 9.8% and mechanical techniques with 0.5%, implying the most convenient method to be enzymatic digestion. The enzymatic digestion process of the dental pulp refers to the use of enzymes, such as collagenase type 1, dispase, and trypsin, to decompose the dental pulp tissue in order to obtain stem cells. Such a method involves the use of specific enzymes that could degrade the structures of the pulp tissue, thus releasing the stem cells present in it [32, 33]. Ebrahimi Dastgurdi et al. [34] used collagenase type 1/dispase or collagenase type 1 alone, concluding different enzyme solutions to give rise to different populations of DPSCs. The lack of homogeneity in the type of enzyme and the amount used can put the viability and function of DPSCs at risk, further complicating the stem cell isolation process [31].
After enzymatic disintegration, DPSCs were passed through a 100 μm Falcon-type cell sieve. This procedure was carried out with the purpose of eliminating any cell grouping [35]. Another study demonstrated the usefulness of 40, 70, and 100 μm Falcon tubes for filtering primary cells [31]. That is why the samples were passed through different filters, with the 100 μm Falcon tubes used to retain larger particles and allow the dental pulp stem cells to pass through the filter. However, there could have been a loss of some cells during the filtration process, which could have affected the culture efficiency and cell recovery. During this filtration, the cells might have experienced some mechanical stress due to the pressure exerted to pass through the filter, and this could have damaged the cells or affected their viability. In contrast, 35 μm Falcon tubes had smaller pores and were used to retain smaller particles; therefore, DPSCs could have become trapped in the filter, limiting cell recovery.
Although the importance of pH is clear, there are no consensus guidelines on best practices for pH management in cell culture [36]. The pH of the DMEM medium used for mesenchymal cell isolation was measured to be 8.26, indicating a more basic environment compared to the optimal pH of 7.4 typically required for the growth of most mammalian cells. This deviation from the ideal pH may have influenced cell growth.
The use of antibiotics in stem cell culture medium is a common practice in scientific research and cell culture to prevent microbial contamination and maintain a sterile environment for stem cell growth and expansion. Varghese et al. [37] determined that a combination of antibiotics, gentamicin and penicillin-streptomycin (pen-strep), had a negative impact on cell viability during the differentiation process of human embryonic stem cells. In contrast, Llobet et al. [38] used a mixture of penicillin 100 U/ml-streptomycin 100 μg/ml-amphotericin B 0.25 μg/ml, or gentamicin 5 μg/ml, proving that these antibiotics affected cell differentiation. On the other hand, Raoof et al. [30] used 100 U/ml of penicillin G, 100 μg/ml of streptomycin, and 1 μg/ml of amphotericin B, obtaining favorable results in the isolation of stem cells from the dental pulp. Several bacterial strains were also found to be resistant to pen-strep, for which other broad-spectrum antibiotics, such as normocin and gentamicin, were used [39]. The use of pen-strep in dental pulp stem cell culture medium is a common practice to prevent bacterial infections and maintain a clean culture environment; however, it is important to evaluate whether DPSCs are sensitive to these antibiotics. In the present study, 5% pen-strep (ref. P0781; Sigma Aldrich) was used in all groups. Although penicillin and streptomycin are effective antibiotics against many bacteria, they could also be toxic to the stem cells of the dental pulp in high concentrations or with prolonged exposure. This could negatively affect the viability and function of dental pulp stem cells.
The use of an antifungal drug, such as amphotericin B, which is a broad-spectrum antifungal, has become important in cell culture [30]. However, it has been reported that the use of amphotericin B is limited by its strong nephrotoxic properties [40]. A study combined amphotericin B with copper (II) ions, which exhibited strong antifungal properties and was less harmful to cells compared to amphotericin B alone [41, 42]. Utumi et al. [43] determined that fluconazole in canine DPSCs did not significantly interfere with the replication of these cells, but in the cytotoxicity analysis, there was a loss of viability and cell death as the concentration of fluconazole was increased. On the other hand, Skubis et al. [44] proved that the use of amphotericin B led to a decrease in cell viability and a significant increase in cellular mitochondrial oxidative activity. Therefore, amphotericin B could be toxic to cells due to its action mechanism, reducing cell viability and function, and negatively affecting the usefulness of cell cultures.
The protocols for DPSCs’ culture usually use FBS, although an increasing number of studies are now exploring non-FBS-based culture systems. Current culture methods for the isolation and expansion of adult stem cells rely heavily on the use of significant quantities of animal sera; however, animal-derived culture components raise concerns due to the real possibility of infections and serious immune reactions [45]. Ferro et al. [46] showed that it was possible to replace FBS with a complete chemically defined culture medium containing reduced concentrations of human serum (HS), obtaining good results. Eubanks et al. concluded that DPSCs could be isolated and expanded to clinical scale numbers in media without FBS and they still maintained their phenotypic properties, but the in vivo regenerative capacity of these DPSCs has not yet been determined [47]. Piva et al. [48] reported the use of HS to have a greater capacity for angiogenesis in vivo. Several studies have reported the use of FBS in different concentrations (at 20% [9, 30, 49] and 10% [50-52]), obtaining good results in the isolation of DPSCs. In the present study, 10% FBS was used due to its ability to provide essential nutrients and growth factors. However, ethical issues and serum quality should be considered, and alternatives without animal-based components could be explored if necessary.
Another reagent used in stem cell culture was L-glutathione since it is an antioxidant and an amino acid that plays an important role in protecting cells against oxidative damage and regulating cellular function [53]. Hee Cho et al. [54] described the importance of cellular glutathione (GSH) levels in regulating MSCs’ functions and presented FreSHtracer as an effective tool to evaluate and select highly functional MSCs. In our results, glutathione could have been a useful tool in DPSCs cell culture, especially to protect them against oxidative stress, improve viability, and regulate cell differentiation. However, it was important to optimize concentrations and monitor GSH levels to ensure they were in the appropriate range for the type of culture.
The present study has addressed several important considerations in the isolation and culture of DPSCs and highlighted some limitations and challenges associated with these processes, such as cellular contamination. Additionally, the lack of matching between groups represents another limitation of the study, which may have influenced the outcomes. This underscores the necessity of having a standardized protocol for the isolation and characterization of DPSCs to guarantee reproducible and reliable results.
The absence of cells in group 3 can be attributed to several factors. First, pericoronitis, as an inflammatory condition, likely compromised the initial viability of the pulp tissue, leading to a reduced number of viable stem cells. Additionally, microbial contamination associated with the infected tissues may have interfered with the culture process. It is also possible that the protocol used was not suitable for addressing the specific characteristics of such compromised tissue, such as adjustments in pH or reagents. Finally, undigested tissue fragments may have limited the release of viable cells. These observations underscore the need to optimize protocols to address adverse clinical conditions effectively.
The use of explant or enzymatic decomposition techniques is common, and it is important to follow sterile procedures to avoid bacterial or fungal contamination; the method selection may vary depending on the research objectives and laboratory preferences. Additionally, the use of cell strainers, such as 100 μm Falcon tubes, could have removed cell aggregates and ensured purity in cell populations, generating variable results. Also, the use of antibiotics and antifungals in the culture medium is common to prevent infections, but their choice and concentration have to be carefully evaluated. Some studies have found certain antibiotics to affect cell viability or differentiation, so it is important to consider these factors.
Another most commonly used and studied reagent in cell culture is FBS, but its animal origin raises ethical and quality concerns. Alternatives, such as chemically defined culture media or HS, are being explored to reduce reliance on animal-derived components.
The use of L-glutathione as an antioxidant in DPSCs cell culture may be a practice that could have helped protect cells from oxidative stress and improve their viability. However, the concentration and levels had to be optimized and monitored to avoid adverse effects.
CONCLUSION
In the culture of dental pulp stem cells, common challenges have been identified, including issues with medium handling, pH regulation, the presence of undissolved particles, cell adherence, and bacterial contamination. These findings emphasize the need to standardize protocols to maximize their therapeutic potential and clinical applications.
AUTHORS’ CONTRIBUTION
L.C.B.: Study conception and design; A.P.J.: Data collection; E.P.I., X.A.V.L.: Analysis and interpretation of results; B.V.R.: Drafting of the manuscript; E.Q.P.: Investigation; M.P.S.: Validation. All authors have reviewed the results and approved the final version of the manuscript.
LIST OF ABBREVIATIONS
PBS D8537 | = Dulbecco's phosphate buffered saline |
DPSCs | = Dental pulp stem cells |
LLLI | = Low-level laser irradiation |
FBS | = Fetal bovine serum |
MSCs | = Mesenchymal stem cells |
PDLSCs | = Periodontal ligament stem cells |
ETHICS APPROVAL AND CONSENT TO PARTICIPATE
The study was approved by the ethics committee of Santiago-Lugo (CEI-SL) (protocol code: 2023/484), Spain.
HUMAN AND ANIMAL RIGHTS
All human research procedures followed were in accordance with the ethical standards of the committee responsible for human experimentation (institutional and national), and with the Helsinki Declaration of 1975, as revised in 2013.
AVAILABILITY OF DATA AND MATERIALS
The data and supportive information are available within the article.
ACKNOWLEDGEMENTS
The authors would like to thank the University of the Americas (UDLA), the University of Santiago de Compostela, and the University of Guayaquil for providing the opportunity to conduct this research.