All published articles of this journal are available on ScienceDirect.
Antibacterial Activity of Plectranthus scutellarioides Leaf Against Actinomyces viscosus and Biocompatibility Testing on hFOB 1.19 Cell Line
Abstract
Background
Actinomyces spp. is a G+ facultative anaerobic branching bacilli that has been implicated in infections of the craniofacial, chest, and abdomen districts. Actinomyces viscosus is one of the early colonisers in the root surface and is associated with the development of caries. This study investigates the use of Plectranthus scutellarioides (L.) R.Br. leaf extract for its antibacterial properties against A. viscosus and its biocompatibility with the human foetal osteoblasts cell line (hFOB 1.19).
Methods
Activity of the lyophilized ethanolic leaf extract of P. scutellarioides was tested using the disc diffusion method followed by the determination of minimum inhibitory concentration (MIC) and minimum bactericidal concentration (MBC). Bacterial morphologic changes were observed using a scanning electron microscope (SEM). In vitro, the biocompatibility of the plant extract with the hFOB 1.19 cells was investigated using the MTT assay.
Results
An initial zone of inhibition of A. viscosus was observed with a P. scutellarioides extract concentration of 100 mg/mL and increased at an extract concentration of 200 mg/mL. The MIC50 is 1.56 mg/mL with a percentage of inhibition of 52%. At 12.5 mg/mL, the plant extract demonstrated a total bactericidal effect (MBC), whereby SEM images depicted bacterial cell wall destruction. The ethanolic extract did not exert any cytotoxic effect on the hFOB 1.19 cells at all concentrations.
Conclusion
Results suggest that P. scutellarioides leaf extract is a potential antibacterial agent against A. viscosus. The in vitro biocompatibility test revealed no cytotoxicity towards hFOB 1.19 cells. However, further testing is required to establish P. scutellarioides as an antibacterial agent for the prevention or treatment of periodontal disease or peri-implantitis.
1. INTRODUCTION
Actinomyces viscosus is a gram-positive, anaerobic bacillus bacterium, a commensal organism in the oral cavity in up to 70% of individuals. Pathogenicity is generally low, with only a small fraction of cases associated with dental infections [1]. The bacterium is mostly isolated from plaque-overlying root lesions. However, the onset of the infection is difficult to ascertain because they are often detected on the sound root surfaces in both subjects experiencing and those resistant to root caries [2]. Early colonization of the tooth surface begins with the adhesion of salivary bacteria onto the pellicle [3]. Actinomyces species are among the primary colonizers in this early plaque formation on the tooth and they may also be found on mucosal surfaces [4]. They provide a substrate for the adherence of more fastidious plaque microbes, which includes gram-negative obligate anaerobes through co-aggregation [5, 6]. Hence, the composition of the mature dental plaque, which often includes potential oral pathogens, is dependent on the primary binding of these pioneer bacteria [7]. The same phenomenon can be observed on the transmucosal abutment of dental implants, where microbial colonization occurs on the implant surface [8-10]. The initial attachment of these early colonizers on the implant surface allows for adherence of the polymicrobes that may lead to implant-associated infections [11].
Actinomyces viscosus is the leading cause of actinomycosis, a chronic subcutaneous infection depicted by abscesses, fibrosis and draining sinuses [12]. Damage to the skin or mucous membrane and reduced immunity leads to their pathogenicity from a commensal state [13]. Intraoral and extraoral actinomycotic infections occur, namely in the cervico-facial, pulmonary, or pulmono-thoracic and abdomino-pelvic regions. Cervico-facial actinomycosis accounts for 50 to 70% of the cases [14]. The main risk factors are complications of an oral procedure and poor oral hygiene. However, this disease is often misdiagnosed because of its ability to mimic other infections or malignancies, which leads to inaccurate management or treatment [15]. The treatment is a high dose of parenteral and oral β-lactam antibiotics for an extended period. Additionally, surgical debridement may be needed in some cases [16, 17].
Actinomyces spp. are susceptible to many types of antibiotics. Nonetheless, there are growing concerns within the scientific community regarding the increased resistance patterns seen in Actinomyces spp [18, 19]. Wolff et al., in 2022, conducted antimicrobial susceptibility testing on oral isolates of Actinomyces spp. and demonstrated low to high levels of resistance towards benzylpenicillin, meropenem, moxifloxacin, clindamycin, ampicillin-sulbactam and daptomycin [20]. Hence, there is a clear need to investigate alternative anti-infective agents to treat Actinomyces spp. related infections to avoid antibiotic resistance.
The common source of alternative medicine for treating diseases is derived from nature and includes herbal plants. The present study utilizes Plectranthus scutellarioides (L.) R.Br. (syn. Coleus blumei), a herbal plant that belongs to the Lamiaceae family with the genus Plectranthus and species P. scutellarioides. The main objective of the present study was to investigate the plant’s antibacterial effect against Actinomyces viscosus, a form of early colonizing species found in healthy implant sites. Whereby in our previous study, P. scutellarioides was tested against early and late colonizing bacteria that cause peri-implantitis [21]. Peri-implantitis is inflammation of the mucosa around dental implants with progressive loss of the supporting peri-implant bone [22-24]. The second objective of this study is to assess the plant extract's biocompatibility using a human foetal osteoblastic cell line (hFOB 1.19). This aims to test the plant’s ability to perform without inducing toxic or injurious effects on a representative biological system.
2. MATERIALS AND METHODS
2.1. Identification and Preparation of Plant Extract
Leaves of the purple variant of P. scutellarioides were harvested from Taiping, Perak, and identified at the Institute of Bioscience, Universiti Putra Malaysia (UPM), with a voucher specimen (MFI 0063/19) deposited at the herbarium. The leaves were washed with distilled water, air-dried at room temperature, and ground into a fine powder using a mechanical blender. During the extraction process, 100g of the powdered plant material was soaked in 200 mL of 70% ethanol. This process was carried out in a sonicator for 30 minutes. The extract was then concentrated using a rotary evaporator at 45°C under reduced pressure and subsequently lyophilized using a freeze dryer to yield 15g of crystallized extract.
2.2. Microorganisms and Growth Conditions
Actinomyces viscosus (ATCC®15987TM) (ATTC, Virginia, USA) was cultured on Brain Heart Infusion Agar (BHI) and incubated at 37oC under anaerobic conditions for 72 hours by using an anaerobic gas jar with OxoidTM AnaeroGenTM 2.5 L, anaerobic gas pack (Thermo Fisher Scientific, Waltham, Massachusettes).
2.3. Bacterial Suspension
Following the ISO 20776-1(2019) standard from the International Organization for Standardization [25, 26], the bacterial suspension was prepared using similar colonies obtained from an overnight culture on the BHI agar. The cell density of the inoculum to be treated with subsequent dilutions with the plant extract was set at 1x108 cells/mL for the antibacterial screening and 1x106 cells/mL for Minimum Inhibitory Concentration (MIC). The required density equal to 0.5 McFarland standard was determined using a spectrophotometer at a wavelength of 625nm and absorbance in the range of 0.08-0.1. Following this, the suspension was diluted twice using 0.1 mL of bacterial suspension in 9.9 mL BHI broth to achieve a bacterial suspension with a cell density of 1x106 cells/mL.
2.4. Anti-bacterial Test
2.4.1. Anti-bacterial Screenings
The antibacterial screening was done using the disc diffusion method. Oxoid discs were saturated with 10 µL of P. scutellarioides extract at concentrations of 100 mg/mL and 200 mg/mL. Positive controls included antibiotic discs with 10 mg of penicillin and discs with 10 µL of 0.12% chlorhexidine digluconate mouth rinse. Negative controls were discs impregnated with 10 µL of distilled water. The discs were placed on BHI agar pre-inoculated with A. viscosus and incubated at 37°C under anaerobic conditions for 72 hours. The diameter of the inhibition zones around all the discs was then measured and recorded.
2.5. Determination of Minimum Inhibitory Concentration (MIC)
2.5.1. Broth Dilution Method
The minimum inhibitory concentration (MIC) was determined using the microdilution method in a 96-well microplate, following the Clinical and Laboratory Standards Institute (CLSI) guidelines with minor modifications. A 100 mg/mL extract solution was diluted in Brain Heart Infusion (BHI) broth in two-fold serial dilutions to obtain concentrations from 0.1 mg/mL to 100 mg/mL, with each well containing 100 µL of bacterial suspension. Negative control wells (NC) contained only the broth and bacteria suspension.
A separate series of the same dilutions of the plant extract, with the same concentration, was prepared and their absorbance was measured and then subtracted from the test wells to eliminate any possible interference from the extract’s colour. All the plates were then incubated for 72 h at 37°C. Subsequently, the absorbance of each plate was measured at an optical density of 600 nm (OD600). In this study, the MIC represents the percentage of inhibition, notably known as MIC50. MIC50 is the required plant extract concentration to inhibit 50% of the growth of the organisms. The MIC50 was calculated by using the formula below:
OD600 of NC wells - OD600 of the test wells/ OD600 of the NC wells x 100
2.6. Determination of Minimum Bactericidal Concentration (MBC)
Minimum bactericidal concentration (MBC) is characterized as the lowest concentration of the plant extract at which bacterial growth is completely inhibited. 10 µL from the wells with the plant extract concentration displaying MIC50 and higher were plated on agar and incubated at 37°C in appropriate conditions. The plant extract was considered bactericidal if no bacterial growth was observed on the agar plates. When bacterial growth was detected, the extract was considered bacteriostatic.
2.7. Scanning Electron Microscopy (SEM) Evaluation
The SEM analysis preparation followed protocols reported by Yenugu et al., (2004) and Agizzio et al., (2006) with minor modifications [27, 28]. A. viscosus was cultured on BHI agar at 37 °C under anaerobic conditions for 72 h. Subsequently, one or two colonies were transferred into BHI broth and incubated for another 72 h. Following this, the plant extract at MIC50 concentration was added to the suspension and was further incubated for 72 h. After centrifugation of the bacterial suspension at 10000g for 10 min, the obtained bacteria pellet was fixed with 3% glutaraldehyde in 0.1 M phosphate buffer (pH 7.2) for a minimum of 1 h or overnight. Subsequently, the pellet underwent two rounds of centrifugation and was then fixed with 1% osmium tetroxide prepared in phosphate buffer for 1 h at room temperature. After a final round of centrifugation, the pellet was re-suspended in saline and dehydrated using two rounds of 50%, 75%, 95%, and 100% ethanol and finally hexamethyldisilane (HMDS) for 10 minutes each. The bacteria pellet was air-dried at room temperature before being mounted onto an SEM stub and sputter-coated with gold. The samples were then examined under the SEM at 20000× magnification (Quanta 450 FEG, FEI, Oregon, United States).
2.8. HFOB 1.19 Osteoblast Culture
A single osteoblast cell line, hFOB 1.19 cells, ATCC CRL11372 (ATTC, Virginia, USA) was cultured in complete media containing Dulbecco's Modified Eagle Medium (DMEM/F-12) without phenol red (Thermo Fisher, Massachusettes, USA) supplemented with 10 mL of foetal bovine serum (FBS) (ScienCell, California, USA) and 5 mL of penicillin/streptomycin solution (P/S) (ScienCell, California, USA). The cells were incubated in a 5% CO2 incubator at 37°C with a humidified atmosphere. During the passaging, Dulbecco’s phosphate buffer saline (DPBS) (ScienCell, California, USA) was used to wash the cells before trypsinization. Trypsinization was carried out using 3 mL of Trypsin-EDTA for Primary Cells, ATCC® PCS-999-003TM (ATTC, Virginia, USA). The resulting cell suspension was centrifuged at 1500 rpm for 5 minutes and neutralised with complete media. Following dilution in 1000 μL of complete medium, the cell concentration was determined using a haemocytometer.
2.9. In vitro Biocompatibility Test
2.9.1. MTT Cytotoxicity Assay
The hFOB 1.19 cells were seeded into a 96-well microplate at a cellular density of 1x105 cells/well at 37°C for 24 h in 5% CO2. Subsequently, 100 mL of P. scutellarioides extract with a concentration ranging from 0.20 to 200.00 mg/mL were added to the test wells and the cells were further incubated for 72 h. Following the removal of the culture medium, the cells were washed with 100 mL of phosphate-buffered saline (PBS). MTT assay [3-(4, 5-dimethylthiazol-2-yl)-2,5-diphenyl tetrazolium bromide] was performed by adding MTT solution to each well and incubating for 4 h at 37 °C in 5% CO2. Following incubation, the formazan crystals formed by viable cells were solubilised using DMSO and the plate was further incubated for another 30 minutes at 37 °C. Cell viability was determined using a microplate reader (Infinite® M1000 PRO, Tecan, Männedorf, Switzerland) at an absorbance of λ= 595 nm. The data retrieved were converted into a percentage of cell viability using the following formula:
Cell viability (%) = 1- OD of test wells/ OD of control wells x 100
The IC50 values were determined from the cell viability results. An inhibitory concentration of 50% (IC50) is defined as the drug concentration that results in 50% cell viability reduction and is commonly used to assess the toxicity of a drug in vitro. A drug is considered toxic if its IC50 value is greater than 50% (>50%), and non-toxic if the IC50 value is less than 50% (<50%).
2.10. Statistical Analysis
The experiments were conducted in triplicate and repeated three times (n = 9). The results are presented as mean values ± standard deviations. A repeated measures ANOVA model was employed to assess the mean differences between the variables of interest in the antibacterial screening and cell viability testing, and the significance value was accepted at p < 0.05. The statistical analysis was performed using SPSS Statistics Version 26 (IBM, New York, USA). A summary of the research methodology is given by the flowchart in Fig. (1).
3. RESULTS
3.1. Antibacterial Screening
P. scutellarioides exhibited antibacterial activity at the tested concentrations, namely 100 mg/mL and 200 mg/mL (Table 1). The findings suggest a positive correlation between the concentration of the plant extract and the zone of inhibition. A statistically significant difference between the groups can be seen as determined by the repeated measures ANOVA Model in Table 2.
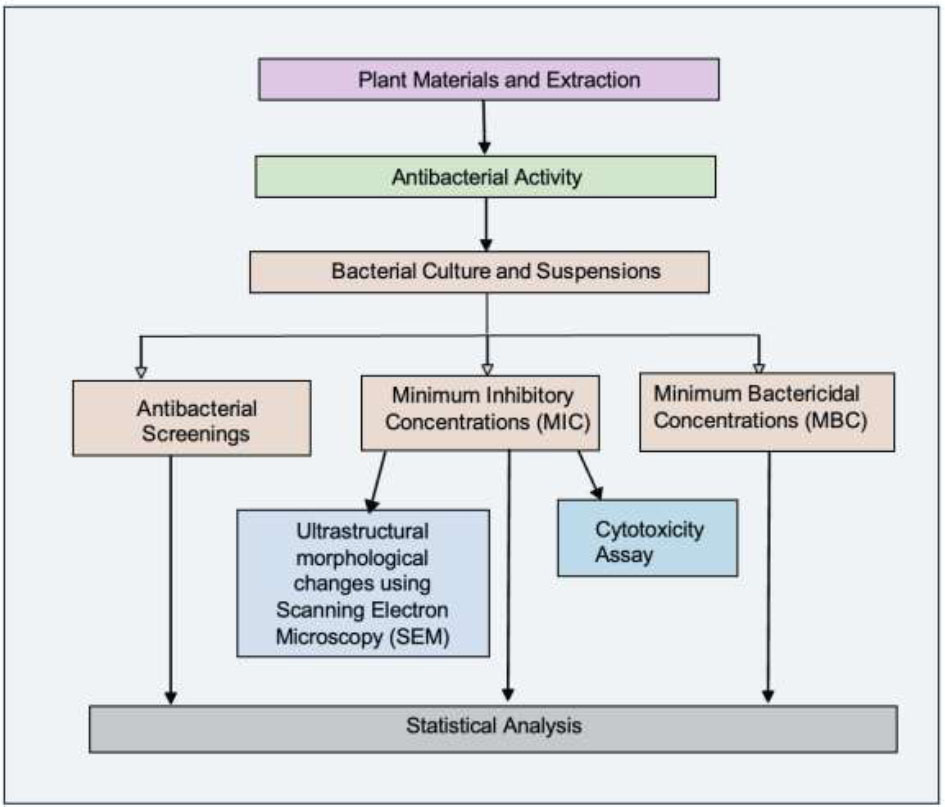
Research methodology flowchart.
- | Extract (100 mg/mL) | Extract (200 mg/mL) | Oradex | Penicillin (10 µg) | Distilled Water |
---|---|---|---|---|---|
- | - | Zone of Inhibition (mm) | - | - | - |
A. viscosus | 17.0 ± 2.2 | 21.0 ± 2.2 | 23.0 ± 2.6 | 30.0 ± 3.0 | 0.0 |
Comparison between Groups | A. viscosus |
---|---|
p-value (<0.05) | |
Extract 100 mg/mL vs Extract 200 mg/mL | 0.000* |
Extract 100 mg/mL vs Oradex | 0.000* |
Extract 100 mg/mL vs Antibiotic | 0.000* |
Extract 200 mg/mL vs Oradex | 0.000* |
Extract 200 mg/mL vs Antibiotic | 0.000* |
Wells | Concentration (mg/ml) | OD of Test Well | OD of Blank Well | Percentage of Inhibition (MIC and MIC50) |
---|---|---|---|---|
1 | 100 | 3.6433 ± 0.177 | 3.6151 ± 0.3572 | 95% |
2 | 50 | 2.7000 ± 0.3620 | 2.6244 ± 0.3034 | 85% |
3 | 25 | 2.171 ± 0.3384 | 2.0553 ± 0.0664 | 80% |
4 | 12.5 | 1.7549 ± 0.6762 | 1.6123 ± 0.2037 | 75% |
5 | 6.25 | 1.3948 ± 0.3837 | 1.2403 ± 0.2364 | 73% |
6 | 3.13 | 1.2077 ± 0.4319 | 1.0046 ± 0.0606 | 54% |
7 | 1.56 | 1.1712 ± 0.5335 | 0.8976 ± 0.0512 | 52% |
8 | 0.78 | 0.8422 ± 0.1087 | 0.4839 ± 0.0608 | 37% |
9 | 0.39 | 0.7921 ± 0.1458 | 0.3701 ± 0.0812 | 26% |
10 | 0.20 | 0.7057 ± 0.1781 | 0.2316 ± 0.1122 | 16% |
12 | NC | 0.5685 ± 0.2075 | - | - |
Bacteria | Concentration (mg/ml) | Results |
---|---|---|
Actinomyces viscosus | 100 | Bactericidal |
50 | Bactericidal | |
25 | Bactericidal | |
12.5 | Bactericidal | |
6.25 | Bacteriostatic | |
3.13 | Bacteriostatic | |
1.56 | Bacteriostatic |
3.2. Determination of MIC and MBC
Based on Table 3, MIC values were detected at the concentration of 100 mg/mL (Percentage inhibition= 95%), 50 mg/mL (Percentage inhibition= 85%), 25 mg/mL (Percentage inhibition= 80%), 12.5 mg/mL (Percentage inhibition= 75%), 6.25 mg/mL (Percentage inhibition= 73%), 3.13 mg/mL (Percentage inhibition= 54%) and 1.56 mg/mL (Percentage inhibition= 52%). Therefore, the MIC50 for P. scutellarioides plant extract was 1.56 mg/mL.
As seen in Table 4, the plant extract at MIC of 100 mg/mL, 50 mg/mL, 25 mg/mL, and 12.5 mg/mL were bactericidal. The MBC for P. scutellarioides plant extract was 12.5 mg/mL, or the lowest MIC concentration that exhibited no bacterial growth. The remaining plant extract with a MIC of 6.25 mg/mL, 3.13 mg/mL and 1.56 mg/ml were bacteriostatic.
3.3. SEM Evaluation of the Bacterial Morphological Changes
Before exposure to the P. scutellarioides plant extract, the bacteria exhibited an intact and well-defined cell wall membrane, maintaining their characteristic rod and filamentous forms. A. viscosus was treated with an MIC50 concentration of 1.56 mg/mL, and the photomicrograph revealed a scarcity of the bacteria cells and disruption in shape (Fig. 2).
3.4. Biocompatibility Test
3.4.1. In Vitro Cytotoxicity Analysis
In the present study, the hFOB 1.19 cells exposed to P. scutellarioides leaf extract at exposure times of 24, 48 and 72 hours showed markedly reduced viability of hFOB 1.19 cells in a dose-dependent manner with viable cell percentages ranging from 169% to 51% (Table 5). P. scutellarioides leaf extract elicited a high inhibitory effect on hFOB throughout the incubation period markedly at a concentration of 200, 100 and 50 mg/mL (Fig. 3). However, it is notable that the percentages of the cell’s viability were still above the IC50, revealing that the extract was non-cytotoxic even at high concentration levels.
Table 6 indicates that the cell viability of hFOB 1.19 differed when exposed to different concentrations of the plant extract at the various periods of observation. Cell viabilities differed significantly between the tested concentrations and time points except for 100 mg/mL (24-48 hours and 48-72 hours group) and 50 mg/mL (24-72 hours and 48-72 hours group).
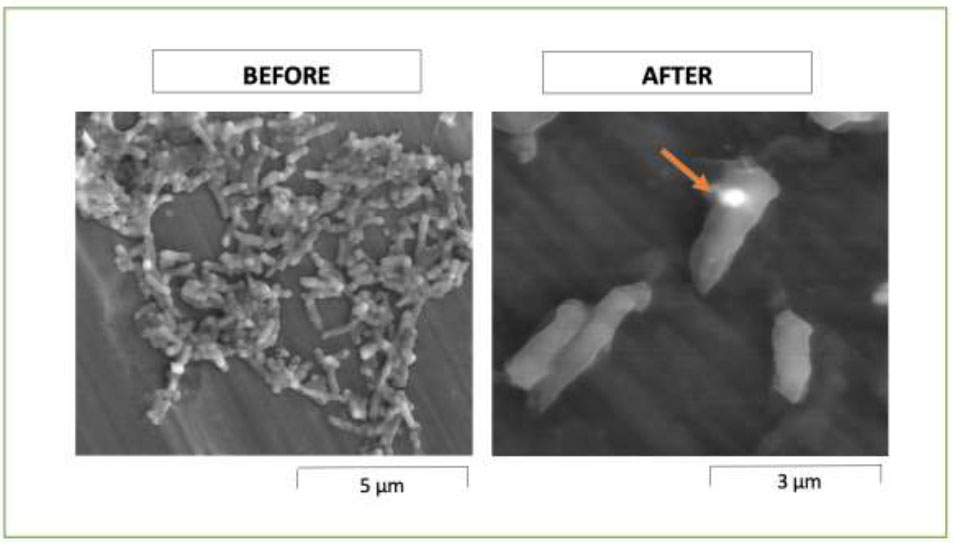
SEM photomicrograph of A. viscosus before and after treatment with P. scutellarioides extract at 20000× magnification, arrow points to disruption in shape.
Concentrations (mg/ml) |
- | Percentage of Cell Viability (%) | - |
---|---|---|---|
- | 24 Hours | 48 Hours | 72 Hours |
200 | 55 | 56 | 51 |
100 | 55 | 56 | 52 |
50 | 57 | 60 | 52 |
25 | 73 | 61 | 54 |
12.5 | 83 | 68 | 56 |
6.25 | 105 | 86 | 61 |
3.13 | 105 | 93 | 62 |
1.56 | 106 | 97 | 70 |
0.78 | 114 | 102 | 91 |
0.39 | 119 | 110 | 92 |
0.2 | 169 | 163 | 140 |
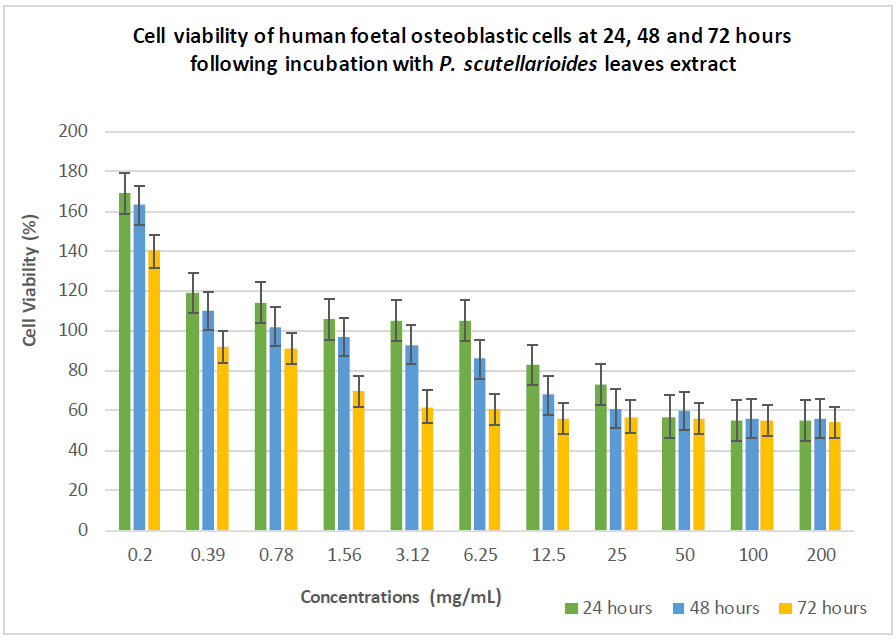
The effect of different extract concentrations of Plectranthus scutellarioides (L.) R.Br. leaf extract on the cell viability of hFOB 1.19 cells at 24, 48 and 72 hours.
Concentrations (mg/ml) |
- |
Time Observation (p˂0.05) |
- |
---|---|---|---|
- | 24-48 Hours | 24-72 Hours | 48-72 Hours |
200 | 0.011* | 0.018* | 0.011* |
100 | 0.08 | 0.02* | 0.09 |
50 | 0.007* | 0.05 | 0.06 |
25 | 0.004* | 0.002* | 0.031* |
12.5 | 0.003* | 0.001* | 0.002* |
6.25 | 0.001* | 0.007* | 0.015* |
3.13 | 0.001* | 0.02* | 0.009* |
1.56 | 0.003* | 0.001* | 0.008* |
0.78 | 0.001* | 0.002* | 0.003* |
0.39 | 0.003* | 0.003* | 0.006* |
0.2 | 0.001* | 0.001* | 0.002* |
4. DISCUSSION
P. scutellarioides exhibited antibacterial activity against Actinomyces viscosus. The inhibition zones increased when the concentration of the plant extract was elevated from 100 mg/mL to 200 mg/mL, indicating a concentration-dependant relationship. It was observed that the inhibition zone of Oradex slightly exceeded that of the 200 mg/mL plant extract, indicating comparable effectiveness between the plant extract and the mouthwash. In this study, chlorhexidine gluconate mouth rinse was chosen as it is a commonly used antiseptic agent whereby expert consensus has recommended that it may be considered for a limited period following periodontal surgery. However, in vivo investigation has shown that usage of chlorhexidine led to a decrease in microbial diversity in plaque and saliva, favouring a streptococci or caries predisposing bacterial community in addition to promoting antibiotic resistance gene, namely to tigecycline, tetracycline, doxycycline and minocycline antibiotics. Thus, it was suggested that chlorhexidine must be prescribed only when indicated and its availability as an over-the-counter product must be reconsidered [29]. As a commercial product, Oradex contains additional ingredients, such as polysorbate 20 and poloxamer 407, which may influence its antibacterial properties. These substances serve as emulsifiers, stabilizing emulsions and aiding the solubilization of hydrophobic molecules in a polar media. Research by Nielsen et al., (2016) [30] suggested that these chemicals effectively inhibit the growth and adherence of bacteria in biofilms and biomaterial substances. Thus, to avoid potential interference from substances other than chlorhexidine gluconate, it is recommended that future studies investigate 100% pure chlorhexidine gluconate.
Penicillin (10 µg) demonstrated significantly greater effectiveness compared to other substances, evident by the largest inhibition zones observed. Its superiority can be attributed to the fact that antibiotics are meticulously developed based on a comprehensive understanding of bacterial physiology and biochemistry, enabling the targeted disruption of specific bacterial processes. Furthermore, antibiotics are manufactured as pure compounds, allowing precise control over their properties. In contrast, plant extracts may encompass a broader range of compounds with diverse mechanisms of action. This complexity makes it more challenging to attain the same level of specificity and potency as antibiotics. Despite this, the P. scutellarioides extract exhibited effects comparable to both the mouthwash and antibiotics, highlighting its potential as an antibacterial agent. Quercetin has been elucidated as the main phytochemical compound of P. scutellarioides ethanolic leaves extract [21]. Quercetin is a naturally occurring flavonoid found mostly in vegetables and fruits and has been identified for its therapeutic potential in preventing and treating various diseases, including cardiovascular diseases, cancers, and neurodegenerative conditions [31]. It is crucial to emphasize that isolating and purifying the active components could likely reveal a higher level of antibacterial activity. Therefore, further work is required in the isolation and purification of the quercetin derivatives identified and further anti-bacterial testing to assess their effectiveness. Negative control (distilled water) did not show any zone of inhibition as anticipated, which indicated that its application in dissolving the dried crude extract neither increased nor decreased the antimicrobial properties of the plant extracts.
A profound finding was that bacterial inhibition, MIC50 and bacterial death MIB were observed at extract concentrations as low as 1.56 mg/mL and 12.5 mg/mL, respectively. The present findings are an extension of a study by Bismellah et al., 2022 where it was found that P. scutellarioides leaf extract demonstrated significant antibacterial activity on both Gram-positive as well Gram-negative bacteria implicated in peri-implant infections [21]. In that study, the bacteria were categorized into early and late colonizers: early colonizers included viridans streptococcus species, while late colonizers comprised facultative anaerobes such as Aggregatibacter actinomycetemcomitans and obligate Gram-negative anaerobes including Porphyromonas gingivalis, Tannerella forsythia, Prevotella intermedia, and Treponema denticola. In biofilm development around dental implants, Actinomyces spp. including A. viscosus plays a vital role in the initiation of adhesion and accumulation of multispecies pathogens to form a complex multi-species biofilm around dental implants [32]. Removing A. viscosus-associated biofilm may be essential in the prevention and regeneration of bone lost around implants associated with peri-implant disease.
The presence of phytochemicals in a plant extract is highly dependent on the type of solvent used in the extraction procedure. Many studies have found that extracts in organic solvents demonstrated more reliable antimicrobial activity when compared to water extraction [33]. The quality of an extract is frequently assessed by the polarity of the solvent employed, to ensure the extraction of all the present phytochemicals, including potentially novel compounds. Additionally, their capability to dissolve or diffuse in the various solvents also contributes to the bioactivity of the plant extract [34]. It is well established that methanol and ethanol extracts contain higher polar compounds than water [35]. Despite the high extraction yield when using water as a solvent, the extract produced may contain a high level of impurities, such as organic acids, sugars, and soluble proteins, along with other polar compounds that can interfere with the identification and quantification process. Conversely, pure alcohol solvents produce a lower extraction yield. Hence, the combination of water with other organic solvents can establish a moderately polar medium with optimal conditions for extraction.
Ethanol, known for its efficacy as a solvent for polyphenol extraction, is also deemed safe for human consumption. The ethanol-to-water ratio plays a crucial role in swelling the plant parts, thereby increasing the surface-to-contact area between the plant matrix and the solvent. This, in turn, improves the extraction yield. Saifuddin et al., (2011) [36] stated that the ethanol-to-water ratio of 70:30 was considered the best proportion for extraction due to the ability of the medium to attract compounds over a wide polarity range from polar to non-polar compounds. Furthermore, the ratio of 70:30 solvent evaporates easily compared to water and thus prevents contamination of microbes. Nevertheless, there are no specific ratios for the combination of water and alcohol, as different plants have different chemical compounds with different characteristics and polarities.
Besides the choice of solvent, the extraction method also plays an important role in determining the quality of the extract. The present study utilizes ultrasonic-assisted extraction (UAE). UAE employs ultrasound waves to enhance the extraction of compounds through mechanical actions such as cavitation, microstreaming, and sonoporation. These effects help to improve the mass transfer of solvents into plant cells and facilitate the extraction of specific target compounds from the botanical material [37, 38]. Yingngam et al., (2014) observed a higher efficacy of phenolic compounds in Cratoxylum formosum extraction by UAE at 45 kHz, 50.33% ethanol v/v, at 65°C for 15 minutes [39]. The only drawback of this extraction method is the potential formation of free radicals when subjected to a frequency exceeding 20 kHz. This side effect was carefully avoided during the extraction of P. scutellarioides by limiting the ultrasound energy used to less than 20 kHz.
The antibacterial effect of P. scutellarioides is evident through observed morphological changes under the Scanning Electron Microscope (SEM). A. viscosus exposed to the MIC concentration of 1.56 mg/ml exhibited morphological disruption characterised by sparcity and bacterial cells appearing shorter and more compact with evident surface perforation. It is likely that the plant extract might have intervened with the cell division process and resulted in distorted and irregular cell damage to both the peptidoglycan layer and the cell membrane (CM). Cell lysis is frequently linked to damage to the peptidoglycan, the main component of the cell wall, leading to its destruction when subjected to mutations or external stresses [40]. The antibacterial activity of P. scutellarioides against A. viscosus aligns with previous research on plant-derived extracts, such as pomegranate peel extract, which attenuates the virulence of Pseudomonas aeruginosa by disrupting bacterial functions. These findings underscore the potential of plant extracts in combating bacterial pathogens and addressing the growing concern of antibiotic resistance [41].
Biocompatibility in the context of a potential antiseptic agent is its ability to elicit microbial death without triggering any unwanted toxic or immunological response to the recipient or host that receives it. Cytotoxicity tests have been used to determine the biocompatibility of medical devices in contact with the body [42, 43]. The present study utilized an in vitro cytotoxicity test using human foetal osteoblastic (hFOB 1.19) to determine the plant extract biocompatibility for future use in dental applications. Human osteoblasts have been extensively utilized for investigating bone metabolism, bone abnormalities, and tissue responses to implant biomaterials due to their rapid and homogeneous proliferation with a doubling time of approximately 36 hours when cultured at 33.5°C [44-46].
Osteoblasts are specialized cells responsible for synthesizing and secreting bone matrix, a substance that undergoes mineralization that transforms proteins into newly formed tissue, thereby contributing to bone growth, repair, and maintenance. During the resorption phase, osteoclasts secrete enzymes and acids that dissolve the mineralized bone matrix, creating cavities or pits on the bone surface. During the formation phase, osteoblasts synthesize new cell bone matrix by depositing collagen and other minerals. The freshly formed bone gradually fills the cavities left by the osteoclasts, thus restoring the bone's structure and strength [47, 48]. The hFOB 1.19 is recognized as immortalized yet non-transformed osteoblast cells with minimal chromosomal abnormalities and extracellular matrix synthesis activity, comparable to primary osteoblasts. Hence, Subramaniam et al., (2002) and Yen et al., (2017) suggested that this cell line is an excellent model for investigating osteoblast's biology in vitro [46, 49]. Based on the cytotoxicity results, the plant extract was non-toxic towards the hFOB 1.19 cells, even at higher concentrations for all the observed periods. At lower concentrations, the extract elicited high cell proliferation. The presence of flavonoids in the plant extract may have contributed to cellular proliferation as they have been found to promote bone formation and reduce bone resorption by regulating different cell signalling pathways [50].
The presence of quercetin in P. scutellarioides may influence the proliferation of hFOB 1.19 cells. The mechanism by which quercetin promotes bone formation at a cellular level is through the enhancement of signalling pathways involved in bone marrow mesenchymal stem cells (BMST) differentiation into osteoblasts (OB) such as the Wnt/β-catenin and the BMP-2/SMADs/RUNX2, by downregulating reactive oxygen species (ROS) and upregulating anti-oxidant genes (Nrf2, CAT, SOD-1, and SOD-2) thus maintaining the viability of BMST and OB, and by upregulating formation of non-collagenous proteins such as osteopontin and osteocalcin which leads to bone formation and mineralization [51]. Braun et al., 2011 [52] also mention that quercetin exerted a protective effect on osteoblasts cultured under cigarette smoke medium by increasing the expression of various antioxidant enzymes HO-1 and SOD-1. These beneficial effects of quercetin on bone metabolism may warrant further investigation of quercetin derivatives in P. scutellarioides on their potential role in the osteointegration of dental implants.
Besides hFOB 1.19 cells, Bismelah et al., (2022) also reported that P. scutellarioides leaves extract did not exhibit cytotoxicity on normal human gingival fibroblast cells (HGnF) at 72 hours of exposure [21]. A recent study by Kowalczyk et al., (2024) focused on the ariel and roots of the plant. One of the objectives of the study was to investigate the cytotoxicity of ariel and roots part of P. scutellarioides against breast cancer cells (MCF-7), human lung adenocarcinoma cells (A549) and normal human gingival fibroblasts (HGF-1) [53]. The findings indicated that both the aerial and root components of the plant exhibited cytotoxic effects on the cancer cell lines. Furthermore, it was observed that the plant was not cytotoxic towards normal cell lines at concentrations that displayed toxicity towards the cancer cell lines following 24 hours of exposure. Nevertheless, the limited number of studies carried out on the effects of P. scutellarioides on normal cells requires further in-depth investigations for completeness in its biocompatibility profile.
CONCLUSION
To conclude, the ethanolic extract of P. scutellarioides leaf successfully demonstrated antibacterial effects against A. viscosus in low concentrations. The antibacterial screening results revealed that the antibacterial activity increased with the increase in the concentrations of the plant extract. The SEM analysis indicated that the extract has the potential to alter the morphological structure of bacterial cells by inducing damage to the cell wall. More bacterial destruction is expected if treated with higher extract concentrations. The extract was non-toxic towards hFOB 1.19 cells, suggesting its biocompatibility with the cells.
AUTHORS' CONTRIBUTIONS
N.A.B.: Was responsible for conceptualization, methodology, and formal analysis, as well as writing the original draft; R.A. and Z.H.K.: Contributed to conceptualization and supervision; R.A., Z.H.K., and N.D.A.: reviewed and edited the manuscript. All authors read and approved the final version of the manuscript.
LIST OF ABBREVIATIONS
ANOVA | = Analysis of Variance |
ATCC | = American Type Culture Collection |
BHI | = Brain Heart Infusion Broth |
CLSI | = Clinical and Laboratory Standards Institute |
DMEM | = Dulbecco’s Modified Eagle Medium |
DMSO | = Dimethyl sulfoxide |
hFOB 1.19 | = Human fetal osteoblast cells |
HGnF | = Human gingival fibroblasts |
HMDS | = Hexamethyldisilazane |
IC50 | = Half-maximal inhibitory concentration |
MBC | = Minimum bactericidal concentration |
MIC | = Minimum inhibitory concentration |
MTT | = 3-(4,5-dimethylthiazol-2-yl)-2,5-diphenyltetrazolium bromide |
NC | = Negative control |
OD | = Optical density |
PBS | = Phosphate-buffered saline |
SEM | = Scanning Electron Microscopy |
SPSS | = Statistical Package for the Social Sciences |
UPM | = Universiti Putra Malaysia |
DATA AVAILABILITY
Data sharing is not applicable to this article as no new data were created or analysed in this study. All results were presented in this article.
FUNDING
This work was supported by Exploratory Research Grant 600- RMI/ERGS 5/3 (39/2013), Malaysia.
ACKNOWLEDGEMENTS
Declared none.