All published articles of this journal are available on ScienceDirect.
The Effect of Two Different MTA (Mineral Trioxide Aggregate) On Thermal Insulation
Abstract
Background
The heat generated due to polymerization in deep cavities can lead to irreversible damage to the pulp. The aim is to evaluate the effect of two different Mineral Trioxide Aggregate (MTA) on the insulation of heat transmitted to the pulp in-vitro.
Methods
This study utilized an apparatus consisting of Teflon molds connected to a digital thermometer with a thermal probe (ENDA-ET4420®-PID-SISEL-TURKEY-ISTANBUL). The temperature difference during the polymerization process (20 and 40 seconds) with light-cured glass ionomer (Fusion-i-Seal®-Prevest-DenPro) was recorded using light-curing devices Elipar (Elipar-S10/3M-ESPE, St.Paul, MN, USA), WoodPecker-LEDB, and WoodPecker-LEDB/Discrete (KejuMed.Prod., Foshan, China) as the control group (non-MTA group). The same procedure was also conducted for MTA Group1 (MTA-FORTE, PyraxPolymers, India) and MTA Group2 (Biofactor MTA, Imicryl, Turkey) by placing them underneath light-cured glass ionomer. The data were analyzed using IBM-SPSS-Statistics-22 software.
Results
There was no significant temperature difference between MTA1-MTA2 groups in the Elipar used groups, and the temperature differences in all groups were above 5.5°C. In MTA1, the temperature difference at 20,40 seconds, and in MTA2, the temperature difference at 20 seconds with the Woodpecker/Discrete light-curing device was significantly higher. In MTA2, the temperature difference at 40 seconds with the Woodpecker light-curing device was significantly higher.
Conclusion
MTA, regardless of the type and usage of the light device used during polymerization in deep cavities, serves as an important insulator to protect the pulp from heat.
1. INTRODUCTION
Teeth, as part of nature, have also developed defense mechanisms to protect themselves from decay. Tooth decay, in cases where defense proves ineffective, can progress into the pulp tissue of the tooth, thereby affecting its vitality. According to World Health Organization (WHO), dental caries are the most common non-communicable disease worldwide [1]. Tooth decay does not only reduce people's quality of life but also negatively affects growth and development during childhood. Therefore, tooth caries should be treated. There are many treatment methods for tooth caries depending on the depth of the decay and vitality of the pulp [2]. The causes of pulp exposure can be decay or mechanical trauma. Depending on the extent of pulp exposure, treatments such as direct pulp capping and pulpotomy are applied for vital pulp therapy. To promote reparative dentin formation and maintain pulp vitality, various capping materials are used with the aim of performing these procedures [3]. The aim is to cover the pulp with a tissue-friendly material to maintain its vitality. Calcium hydroxide (CH), mineral trioxide aggregate (MTA), biodentin, Theracal, light-cured glass ionomer cements are generally used as a coating material in order to close the pulp in accordance with the indication [4, 5]. The most frequently used material in the clinical treatment of CH, has been “gold standard” of direct pulp capping materials for several decades [6-8]. CH exhibits some obvious disadvantages, including pulp surface inflammation and necrosis after pulp capping, and the presence of tunnel defects in the dentin bridge. It fails to provide a hermetic seal to the underlying pulp against recurring infection because of microleakage, high solubility in oral fluids, lack of adhesion and degradation over time [6, 7]. For that reason, researchers are still working on new materials. MTA was first introduced in the dental literature by researcher Torabinejad in 1993 and received FDA approval in 1998. MTA is a bioactive material that induces hard tissue formation based on tricalcium silicate. MTA, a material with a wide range of indications, has recently been available in pre-mixed forms in addition to the traditional powder-liquid formulations [9]. Despite all its good qualities, MTA requires an intermediate material between itself and the filling material due to its long setting time and low resistance to forces. Due to these characteristics, MTA is not used as a restoration material. After the capping procedure, many materials are used to protect MTA [10]. Glass ionomer cements are restorative materials that can chemically adhere to enamel and dentin, release fluoride, and are used by mixing a powder and a liquid. To enhance and facilitate their clinical characteristics, resin components have been added. In this way, it has led to improved mechanical strength, better adhesion to enamel and dentin, reduced early moisture sensitivity, and extended working time [11]. Due to the addition of resin to the composition of this material developed for clinical use, it undergoes polymerization with light. The most frequently used polymerization sources today are light emitting diodes (LED), plasma arc lamps and argon ion lasers [12, 13]. The light from curing devices can cause heat transfer to the pulp. According to a study conducted in 1965, a temperature increase of 5.5 °C in the pulp was found to result in irreversible pulp damage. It was concluded that high-power light-curing sources may pose a potential danger to teeth, such as pulpal trauma [14, 15]. Dentin tissue acts as a barrier protecting the pulp against external stimuli [4]. Depending on the depth and width of the cavity formed, the temperature released during the polymerization of the restorative materials used is transmitted to the pulp. This temperature may be one of the main causes of pathological conditions occurring [16]. This present study is an in-vitro study that evaluates the temperature changes in the pulp depending on different MTA types used in vital pulp treatments. In the literature, there is no other study that investigates the insulation effect of different types of MTA on the pulp. The aim of this study is to evaluate the extent to which MTA insulates the heat transmitted to the pulp. To manifest this, it compared the temperature changes during the polymerization of light-cured glass ionomer cement on different MTA types that are applied to the cavity floor with two different LED light sources and three different application methods. The hypothesis is “The coverage of pulp surface with MTA in deep cavities where light-cured glass ionomer cement is used as a base material does not affect the temperature change occurring in the pulp.”
2. MATERIALS AND METHODS
2.1. Temperature Measurement Device
The temperature measurement device (ENDA ET 4420® PID Istanbul, Turkey) consists of a temperature-sensing metal probe (Thermistor NTC 3950 100K, Hictop, Japan) (Fig. 1) and a digital display. The temperature-sensing metal probe has been positioned at the center of a plastic disc (3 x 3 cm). The metal probe measures the instantaneous temperature and displays it on the digital thermometer. In this study, standardized Teflon molds measuring 2x2 cm in size and 2 mm in thickness were used, which were designed to fit snugly into the plastic disc. In the center of the Teflon molds, a hole with a diameter of 3.5 mm was created to represent the cavity (Fig. 2).
2.2. The Materials Used
As a base material, light-cured glass ionomer (Fusion i-Seal-Prevest, DenPro, India), for pulp capping, two different MTAs (MTA-Forte, Pyrax Polymers, India and Biofactor MTA, Imicryl, Turkey), and two different LED light sources; Elipar (S10/3M-ESPE, St. Paul, MN, USA) and WoodPecker (LEDB/KEJU Met. Prod., Foshan, China) devices were used. The utilized light sources were calibrated in accordance with the manufacturers' guidelines.
2.3. Determination of Study Groups
The group using MTA-Forte was named the “MTA1 Group”, and the group using Biofactor MTA was named the “MTA2 Group”. The group in which only light-cured glass ionomer is used and MTA is not applied underneath is referred to as the “Group of non-MTA”.
2.4. Preparation of Samples
MTAs were prepared in accordance with the manufacturer's suggestion. MTA-forte is prepared as 3 parts powder to 1 part liquid. Biofactor MTA, on the other hand, is prepared with 2 drops of liquid for 0,1 g of the powder.
2.5. Application Phase
In the group of non-MTAS, the Teflon mold was placed directly onto the guide groove on the plastic slab. With the guidance of these Teflon molds, a light-cured glass ionomer material, 2mm in height and 3.5mm in diameter was applied. It was polymerized for 20 seconds, a commonly used time in clinical applications, and following the manufacturer's recommended duration of 40 seconds. The wavelength is the same for both groups. In the MTA groups, the Teflon mold was placed onto the guide groove on the plastic slab and fully filled with MTA1 or MTA2. And another Teflon mold was placed and filled with glass ionomer cement. And then polymerized with light curing device. Temperature was recorded after the 20th and 40th seconds of polymerization.
2.6. LED Light Sources
Two different LED light sources were used as light curing units: Elipar (S10/3M-ESPE, St. Paul, MN, USA) and WoodPecker (LEDB/KEJU Met. Prod., Foshan, China) devices. The WoodPecker light device was used in two different modes: continuous light and discrete light. The light sources used were calibrated according to the manufacturers' guidelines.
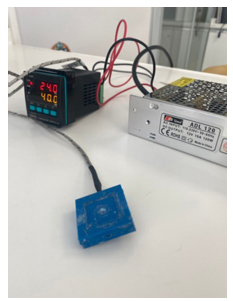
The temperature measurement device -temperature-sensing metal probe- digital display.
2.7. Statistical Analyses
While evaluating the findings in the study, IBM SPSS Statistics 22 programme was used. The conformity of the parameters to the normal distribution was evaluated by the Kolmogorov-Smirnov test, and it was found that the parameters did not show a normal distribution. Kruskal Wallis test was used for the intergroup comparisons of the parameters and the Dunn's test was used for the determination of the group that caused the difference. The Mann-Whitney U test was used for comparisons between the two groups. It was evaluated at a significance level of p < 0.05 (Tables 1 and 2).
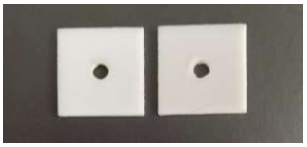
Teflon molds.
3. RESULTS
3.1. MTA1 Group
As seen in Fig. (3), there is a statistically significant difference between the light devices in terms of the temperature differences in the 20th sec (p:0.000; p<0.05). For the determination of significance between the device in which light, post hoc Dunn's test result; Elipar temperature increment of the device, Woodpecker (p:0.000) and Woodpecker/Discrete (p:0.030) devices were significantly higher (P<0.05). The temperature increase in the Woodpecker/Discrete device was also found to be significantly higher than in the Woodpecker group (p:0.018; p<0.05). There is a statistically significant difference between the light devices in terms of the temperature differences in the 40th sec (p:0.000; p<0.05). For the determination of significance between the device in which light, post-hoc Dunn's test result, Elipar temperature increment of the device, Woodpecker (p:0.000) and Woodpecker/Descrete (p:0.012) devices were significantly higher (P<0.05). The temperature increase in the Woodpecker/Descrete device was also found to be significantly higher than in the Woodpecker group (p:0.023; p<0.05).
- | - | Woodpecker | Elipar |
Woodpecker/ discrete |
- |
---|---|---|---|---|---|
MTA | Temperature differences(°C) |
Avg±SD (median)°C |
Avg±SD (median) °C |
Avg±SD (median) °C |
p |
MTA1 | 20th sec | 2,49±0,67 (2,4) | 5,92±1,60 (6,5) | 3,47±0,45 (3,4) | 0,000* |
- | 40th sec | 2,50±0,65 (2,5) | 7,55±1,80 (8,3) | 3,58±0,46 (3,6) | 0,000* |
MTA2 | 20th sec | 4,76±0,69 (4,6) | 5,65±1,10 (6) | 2,82±1,00 (3) | 0,000* |
- | 40th sec | 4,41±0,62 (4,5) | 5,82±0,79 (6,1) | 2,51±0,88 (2,6) | 0,000* |
NonMTA | 20th sec | 7,24±0,69 (7,4) | 9,14±0,72 (9,3) | - | +0,000* |
- | 40th sec | 6,33±0,35 (6,4) | 9,47±0,51 (9,4) | - | +0,000* |
- | - | MTA1 | MTA2 | Non-MTA | - |
---|---|---|---|---|---|
- | - | Avg±SD (median) °C | Avg±SD (median) °C | Avg±SD (median) °C | p |
Woodpecker | 20th sec | 2,49±0,67 (2,4) | 4,76±0,69 (4,6) | 7,24±0,69 (7,4) | 0,000* |
- | 40th sec | 2,50±0,65 (2,5) | 4,41±0,62 (4,5) | 6,33±0,35 (6,4) | 0,000* |
Elipar | 20th sec | 5,92±1,60 (6,5) | 5,65±1,10 (6) | 9,14±0,72 (9,3) | 0,000* |
- | 40th sec | 7,55±1,80 (8,3) | 5,82±0,79 (6,1) | 9,47±0,51 (9,4) | 0,000* |
Woodpecker/ discrete | 20th sec | 3,47±0,45 (3,4) | 2,82±1,00 (3) | - | +0,010* |
40th sec | 3,58±0,46 (3,6) | 2,51±0,88 (2,6) | - | +0,001* |
3.2. MTA2 Group
As seen in Fig. (4), there is a statistically significant difference between the light devices in terms of the temperature differences in the 20th seconds (p:0.000; p<0.05). For the determination of significance between the device in which light, post hoc Dunn's test result, Woodpecker/Descrete devices, Woodpecker (p:0.005) and Elipar (p:0.000 of the device) were significantly lower (P<0.05). There is no statistically significant difference between WoodPecker and Elipar devices (p>0.05). There is a statistically significant difference between the light devices in terms of the temperature differences in the 40th sec (p:0.000; p<0.05). For the determination of significance between the device in which light, post-hoc Dunn's test result, Elipar temperature increment of the device, Woodpecker (p:0.031) and Woodpecker/Descrete (p:0.000) devices were significantly higher (P<0.05). The temperature increase in the Woodpecker device was also found to be significantly higher than in the Woodpecker/Descrete group (p:0.012; p<0.05).
3.3. Group of Non-MTAS
As seen in Fig. (5), the average temperature difference of the Elipar light device in the 20th seconds is statistically significantly higher than the Woodpecker device (p:0.000; p<0.05). The average temperature difference of the Elipar light device in the 40th seconds is statistically significantly higher than the Woodpecker device (p:0.000; p<0.05).
3.4. When Woodpecker Light Device is Used
There is a statistically significant difference between the MTA groups in terms of temperature differences in the 20th seconds (p:0.000; p<0.05). The average temperature difference of the group non-MTA in the first 20th seconds, MTA1 (p:0.000) and MTA2 (p:0.030), are statistically significantly higher than that of their groups (p<0.05). The temperature increase of the MTA2 group is statistically significantly higher than that of the MTA 1 group (p:0.020; p<0.05). There is a statistically significant difference between the MTA groups in terms of temperature differences in the 40th seconds (p:0.000; p<0.05). The average temperature difference of the group made up of non-MTA in the 20th second, MTA1 (p:0.000) and MTA2 (p:0.024), are statistically significantly higher than their groups (p<0.05). The temperature increase of the MTA2 group is statistically significantly higher than that of the MTA 1 group (p:0.024; p<0.05) (Fig. 6).
3.5. When Elipar Light Device is Used
There is a statistically significant difference between the MTA groups in terms of the temperature differences in the 20th seconds (Dec.p:0.000; p<0.05). The average temperature difference of the group made up of non-MTA in the first 20th seconds, MTA1 (p:0.000) and MTA2 (p:0.000) are statistically significantly higher than that of their groups (p<0.05). There is no statistically significant difference between MTA 1 and MTA2 in terms of temperature increase (p>0.05). There is a statistically significant difference between the MTA groups in terms of temperature differences in the 40th seconds (Dec.p:0.000; p<0.05). The average temperature difference of the group made up of non-MTA in 20 seconds, MTA1 (p:0.027) and MTA2 (p:0.000) are statistically significantly higher than that of their groups (p<0.05). There is no statistically significant difference between MTA 1 and MTA2 in terms of temperature increase (p>0.05) (Fig. 7).
3.6. When Woodpecker/discrete Light Device is Used
The temperature increase of the MTA1 group in the 20th seconds is statistically significantly higher than that of the MTA2 group (p:0.010; p<0.05). The temperature increases of the MTA1 group in the 40th seconds is statistically significantly higher than that of the MTA2 group (p:0.001; p<0.05) (Fig. 8).
4. DISCUSSION
This present study was conducted as in vitro to investigate the effect of two different MTA brands as a base material on pulpal temperature changes during the polymerization of light-cured glass ionomer material used for pulp capping.
MTA Forte (MTA1) and Biofactor MTA (MTA2) were used to compare their insulation effect on pulp in deep cavities. The reason for selecting these two MTA brands is their high affordability in terms of cost, and differences in preparation methods and application techniques, according to the manufacturer’s guide. For this purpose, while MTA1 was mixed as 3 parts powder to 1 part liquid, MTA2 was mixed as 0,1 g powder with 2 drops of liquid.
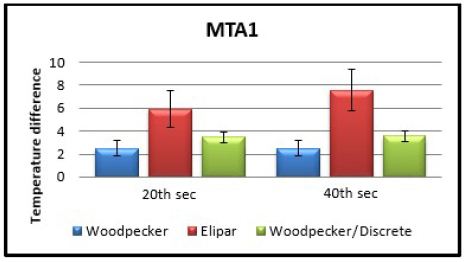
The temperature conveyed by MTA1 underneath as light-cured glass ionomer sets through various light devices.
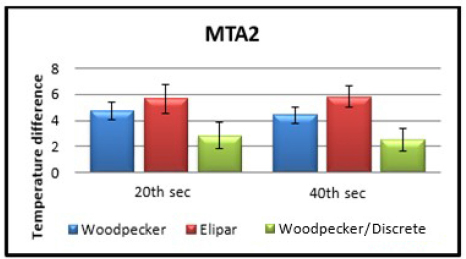
The temperature conveyed by MTA2 underneath as light-cured glass ionomer sets through various light devices.
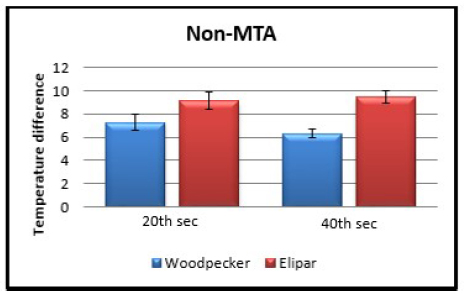
Temperatures measured during polymerization of light-cured glass ionomer using different light devices in the group without MTA application.
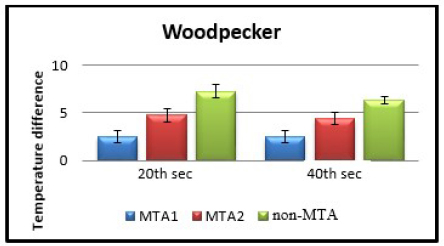
Temperatures measured in the groups with MTA application and without MTA application using the Woodpecker light device.
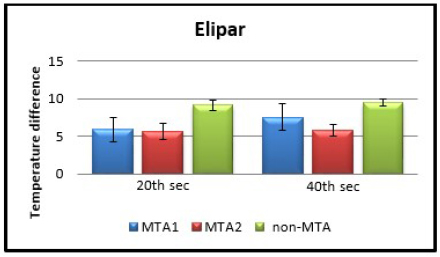
Temperatures measured in the groups with MTA application and without MTA application using the Elipar light device.
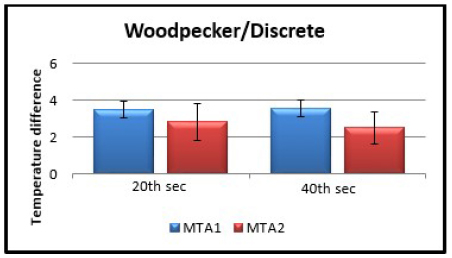
Temperatures measured in the groups with MTA application and without MTA application using the Woodpecker/Discrete light device.
When the contents of the two MTA were evaluated, there was no information about the content of MTA1 (MTA-Forte, Pyrax). Only the manufacturer’s recommendation about mixing procedures that distilled water can be used as a liquid was stated. However, in MTA2 (Biofactor MTA, Imicryl), the powder is composed of tricalcium dicalcium silicate, tricalcium aluminate, and ytterbium oxide. The liquid consists of 0.5%–3% hydrosoluble carboxylated polymer and demineralized water [17].
In clinical practice, like in pulp-capping cases, MTA is frequently used under light-cured glass ionomer. Glass ionomer cement can create an additional layer on MTA to enhance their integrity and durability. They can also be used to prevent potential microleakage. However, there are few studies in the current literature on the interactions between glass ionomer cement and MTA [18]. The injectible form of glass ionomer cement is provided for use in clinical applications to facilitate ease of manipulation [19, 20].
In cases where the degree of polymerization of composite resins is not sufficient, the emission of toxic and carcinogenic substances can cause pulpal reactions. It has been reported that in order to reduce the emitted substances in resin or composite resins, they must be well polymerized [21]. When the edge leakage, mechanical strength and surface hardness levels are compared in the polymerization with LED and halogen light sources, LED light source gives much more successful results than others [22]. It is reported that the temperature increase during polymerization with an LED light source was less than that of halogen light source. Additionally, they have advantages such as longevity, practical usability, and short application time [23, 24]. For that reason, LED light sources were used in this study.
In LED light sources, there are different application modes [24, 25]. Two of them are continuous light and discrete light. In the continuous light mode, the LED light source produces a continuous flow of light. In the discrete mode, the LED light source flashes on and off at specific intervals. The light undergoes periodic interruptions.
Light exposure time is one of the most important factors affecting polymerization [26, 27]. The manufacturer recommends polymerization of light-cured glass ionomer with 30-40 seconds of light exposure, but in clinical practice, it is commonly polymerized with 20 seconds of light exposure. Therefore, in this study, the temperature was measured after polymerization with 20 and 40 seconds of light exposure.
In this study, when all samples polymerized with Woodpecker, Woodpecker/Discrete and Elipar light devices are compared, different MTA types, the amount of temperature transmitted to the pulp decreases significantly in the 20th and 40th seconds. It was observed that the thickness of light-cured glass ionomer over MTA applied at the same thickness was also effective. The temperature difference in the samples with direct light-cured glass ionomer application non-MTA application was found to be significantly higher than the samples applied with MTA. It was observed that the number of temperature increases in both light devices used in the samples applied directly to light-cured glass ionomer was higher than 5.5 °C.
Zach and Cohen reported that temperatures higher than 5.5 °C that may occur in the pulp cause 15-60% irreversible pulp damage [28]. In the samples where Elipar light device was used, it was determined that the temperature difference between the environment and the 40th second was 8,3°C on MTA1, 6,1°C on MTA2 and 9,4°C on non-MTA samples.
In this study, it was observed that when the woodpecker light device was used at the 20th and 40th sec, MTA2 transmitted temperature more than MTA1. There was no difference between MTA1 and MTA2 when the Elipar light device was used. When the woodpecker/ discrete light device was used, it was observed that MTA1 transmitted more temperature than MTA2. That difference may be considered the liquid and powder contents of both MTAs.
The limitations of this study include working with fixed diameter and depth molds, using only 2 brands of MTA and not incorporating different brands, using a single brand of light-cured glass ionomer cement, and not utilizing different modes of light-curing. It may be beneficial to perform a similar study with more examples of different MTA types.
CONCLUSION
According to the results of the study, the hypothesis that “The coverage of pulp surface with MTA in deep cavities where light-cured glass ionomer cement is used as a base material does not affect the temperature change occurring in the pulp” has been rejected.
In this study, regardless of the brand of MTA, it provides insulation in varying degrees for the heat transmitted to the pulp. Another factor affecting the amount of transmitted heat is the type of light-curing devices used. According to the results of this study, MTA2 provides more insulation against heat when used with Woodpecker/Discrete devices. However, in cases where Woodpecker devices are used, MTA1 has been shown to provide more insulation against heat. In situations where no MTA is used alongside glass ionomer, regardless of the light-curing device, a significant increase in temperature on pulp has been observed.
As a result, it was observed that the types of base materials used to cover the pulp in deep cavities and restore it close to the pulp had a significant effect on the temperature transmitted. However, when choosing the light source to be used for the polymerization, attention should be paid to the power of the device, and the device that generates the least temperature during the polymerization should be preferred.
In addition to the areas where MTA is used, if it is used under the light-cured glass ionomer material at the cavity floor very close to the pulp, it increases the temperature insulation that occurs during polymerization and ensures that the pulp remains alive and healing.
In addition to MTA’s, well-known positive characteristics, it can be said that MTA provides superior thermal insulation properties in cases close to the pulp. The effect of different MTA types on temperature conductivity is also different. Therefore, the selection type of MTA is also important in the treatments to be performed.