All published articles of this journal are available on ScienceDirect.
The Impact of Azadirachta Indica Extract on the Expression Profile of Esp Gene in Treated Enterococcus faecalis
Abstract
Background and Objectives
Enterococcus faecalis is a bacterium often associated with persistent endodontic infections, posing challenges for effective root canal disinfection and treatment outcomes due to its resilience in adverse root canal conditions and resistance to antimicrobial therapies. This study aimed to investigate the effects of Azadirachtaindica's active plant compounds at subinhibitory concentrations on the expression of the virulence gene (esp) in Enterococcus faecalis strains (ATCC 29212).
Materials and Methods
Azadirachta indica (neem) specimens were obtained in Erbil, Northern Iraq, and the active compounds from the dried plant samples were extracted. Dry extracts were dissolved in Dimethylsulphoxide (DMSO) until reaching 100 mg/ml. Bacterial samples were treated with the extracted plant material and incubated. The assessment of relative esp gene expression in treated Enterococcus faecalis strains (ATCC 29212) began with total RNA extraction using the RNXplus kit, followed by a Quantitative RT-PCR assay.
Results
The minimum concentration of Azadirachta indica's ethyl acetate fraction 3 (30 mg/ml) inhibited the growth of the targeted bacteria. In contrast, the highest concentration inhibiting the target bacteria was 50 mg/ml of fraction 6 (Azadirachta indica-water). All fractions of Azadirachta indica decreased the expression of the target gene, with fraction 5 (ethyl alcohol) showing the highest fold change.
Conclusion
The ethyl alcohol extraction of Azadirachta indica inhibited the expression of the virulence gene (esp) by 2.84-fold, suggesting a potential decrease in (esp) gene expression, which could be considered as an alternative to classical irrigants.
1. INTRODUCTION
The main reason for the failure of teeth treated with endodontics is the prolonged inflammatory response within the intricate root canal system [1, 2]. This inflammatory cascade ensues when recalcitrant bacteria from the initial infection withstand the exigencies of chemo-mechanical treatment or when a novel, transmissible pathogen emerges, either intraoperatively or postoperatively [3-5].
Enterococci, prevalent in the oral cavity, gastrointestinal tract, and vagina of humans and animals, were once considered nonvirulent but are now acknowledged as significant contributors to nosocomial infections worldwide [6, 7]. Enterococci, Gram-positive cocci found singly, in pairs, or in short chains, are facultative anaerobes that can grow with or without oxygen [8, 9]. Enterococcus, a prominent contributor to endodontic infections, is recognized for its resilience in diverse environmental conditions [10-12]. Furthermore, its survival mechanisms involve genetic variations and the ability to attach to dentin, penetrate dentinal tubules, and withstand periods of starvation [13, 14]. Another study enables us to speculate about a connection between enamel permeability, susceptibility to cavities, and the failure of adhesive bonds in primary teeth [15].
In endodontics, particularly Enterococcus faecalis, is linked to chronic periodontitis and unsuccessful root canal treatments involving chronic apical periodontitis [16-18]. Enterococcus faecalis intricately links to primary, secondary, and persistent infections associated with periradicular disease [19-21]. Notably, Enterococcus faecalis predominantly surface in secondary endodontic infections, with its prevalence ranging from 30% to 90% [22]. These infections typically manifest in previously filled root canals. In such instances, Enterococcus faecalis exhibits a remarkable capacity to form biofilms, thereby exacerbating the complexities of infection resolution [23]. Bacteria deploy virulence factors to invade and persist in the host during infections [24]. Cytolysin, a toxin from Enterococcus faecalis, directly lyses host cells, intensifying bacterial virulence [25]. Gelatinase contributes to pathogenesis by hydrolyzing various host proteins and aiding in adhesion to dentin [26, 27]. Hyaluronidase promotes tissue destruction by breaking down hyaluronic acid [28]. Aggregation Substance (AS) enhances bacterial resistance to macrophages and facilitates adhesion to host cells [29, 30]. Enterococcal surface protein (esp) is linked to infective endocarditis and biofilm formation, impacting E. faecalis virulence [31]. The Enterococcal surface protein (Esp) is a substantial, 1873-amino-acid, Bap-orthologous protein with distinct modules. These include the N-terminal domain (26% sequence identity with Bap), the central core region with domains A and B (23% sequence identity with C-repeats of Bap), and the C-terminal domain containing the LPXTG-like motif found in surface-associated proteins. Esp is integral to biofilm formation by E. faecalis, with its N-terminal domain identified as the minimal region enhancing this process [32]. A comprehensive understanding of the prevalence and pathogenicity of Enterococcus faecalis in these endodontic scenarios is essential for formulating precise and customized therapeutic strategies to address the challenges posed by persistent infections within the root canal system [33]. Biochemical and structural studies revealed that the ~75 kDa N-terminal region of Esp enhances enterococcal biofilms against disruption, particularly under low pH conditions (≤ 4.3), forming an amyloid-like structure. A ~20 kDa fragment, EspDDDK, derived from the very N-terminus, is sufficient for biofilm strengthening at pH ≤ 5.0, suggesting Esp's role in reinforcing biofilms in acidic environments [34].
In the realm of endodontic disinfection, multiple options are available, each with its unique set of advantages and drawbacks [35, 36]. Sodium hypochlorite (NaOCl) stands out as a potent disinfectant effective against a broad spectrum of bacteria, including Enterococcus faecalis, and possesses the ability to dissolve tissues [37] readily. However, its application is not without challenges, as it exhibits cytotoxicity, can cause tissue burns, imparts an unpleasant taste and odor, and has the potential to stain the patient's clothing [38].
On a contrasting note, chlorhexidine has demonstrated notable efficacy in disinfection [39, 40]. Yet, being a synthetic compound, it has been reported to be toxic to various cell types, including fibroblasts, Polymor- phonuclear leukocytes (PMNs), other leukocytes, macrophages, and erythrocytes [41]. To explore alternative methods, diode laser irradiation has emerged as an additional antibacterial disinfection approach in dentistry. However, it is crucial to underline that further research is imperative to confirm the biological safety of employing diode lasers in this context [42].
Due to the increasing worry about antibiotic resistance stemming from excessive usage, it's prudent to exercise caution when prescribing them. Many endodontic infections, which are typically confined within the tooth, can be efficiently addressed through established local treatment methods [40]. Consequently, for conditions such as symptomatic irreversible pulpitis, pulp necrosis, symptomatic apical periodontitis, chronic apical abscess, and acute apical abscess without systemic complications, it is advisable to refrain from the unnecessary administration of additional systemic antibiotics alongside standard endodontic procedures [43]. This sophisticated methodology underscores the significance of customizing therapeutic interventions based on distinct clinical contexts, thereby optimizing the balance between efficacy and patient safety [44, 45].
Diverse irrigants have been enlisted for the management of endodontic infections [46]. Presently, there is a discernible inclination towards contemporary methodologies that prioritize natural remedies over conventional bactericidal medications [46]. Azadirachta indica (A. indica), colloquially known as neem and belonging to the Meliaceae botanical family, has garnered significant attention in this regard, owing to its rich repository of medicinal compounds [47-49]. Traditionally employed in medicinal practices, various components of A. indica, including leaves, bark, and seeds, manifest notable antibacterial and antifungal properties against a spectrum of harmful microorganisms. Furthermore, these components exhibit antiviral effects against vaccinia, chikungunya, measles, and Coxsackie B viruses [50].
The pharmacological prowess of Neem extends beyond its antimicrobial attributes, encompassing a diverse array of therapeutic effects [51]. Notably, its antioxidant [52], antimalarial [53], antimutagenic [54], anticarcinogenic [55, 56], anti-inflammatory [57], antihyperglycemic [58], antiulcer [57, 59], and anti-diabetic capabilities have been extensively demonstrated across various segments of the plant [60, 61], including the leaf, bark, and seeds [62]. Thus, Neem has multiple medicinal qualities that can be useful in endodontic therapy, providing not just protection against microbes but also offering additional therapeutic effects.
The objective of this study was to explore the impact of subinhibitory concentrations of active compounds derived from the neem plant on the expression of the virulence gene (esp) in strains of Enterococcus faecalis (ATCC 29212). The research aimed to examine and understand the effects of these specific neem-derived compounds at concentrations that do not completely inhibit bacterial growth but may still influence the expression of virulence genes in the targeted E. faecalis strains.
2. MATERIALS AND METHODS
2.1. Samples
The process of creating plant extracts involves several crucial steps, including collecting plant material, grinding it to the desired consistency, weighing the plant material, evaporating solvents, filtering the remnants, and calculating the yield percentage. The selection of solvents for extraction was based on the polarity of the solute used in this study, ranging from low to high polarity: Chloroform, Butanol, Ethylacetate, Hexane, Ethanol, and Water. All solvents used in the extraction process served as blanks and controlled in every trial throughout the investigation to entirelysolvent-related influences entirely. For the extraction of plant samples (Azadirachta indica) (50g), a mixture with hexane (100ml) was incubated for 1.5 hours at 37°C. The resultant mixture was filtered to obtain the first extract part (fraction 1), and the solvent was removed using a rotary evaporator. The remaining material was then air-dried at room temperature and used for subsequent solvent extractions. The same method, as employed for other solvents, was used to obtain various extract fractions (fraction 2 from chloroform, fraction 3 from ethylacetate, fraction 4 from butanol, fraction 5 from ethanol, and fraction 6 from water). Dry extracts were dissolved in Dimethylsulphoxide (DMSO) to reach a concentration of 100 mg/ml. The active compounds extracted were utilized in the treatment of Enterococcus faecalis (ATCC 29212), sourced from the Media Center in Erbil, Iraq. Bacterial samples were streaked onto two BHI agar plates (Scharlau Chemie S.A., Barcelona, Spain) and incubated for 24 hours at 37°C. Subsequently, purity was assessed through Gram staining and the examination of colony morphology [63].
2.2. Bacteriology
The process of determining the Minimum Inhibitory Concentration (MIC) was initiated by preparing stock solutions in sterile microcentrifuge tubes provided by Eppendorf. In order to assess the antibacterial effectiveness, a series of dilutions spanning a concentration range from 5 to 100 mg/ml were executed in 96-well microplates. The activation of bacterial suspensions involved achieving a concentration of 1.5 x 10^8 CFU/ml, equivalent to 0.5 on the MacFarland scale, through the utilization of Müeller-Hinton broth. Subsequently, each well of the microplate was inoculated with 10 ml of the reactivated bacterial suspension, and the entire plate was then incubated at 37 °C for a duration of 24 hours to facilitate optimal bacterial growth. This comprehensive methodology ensured a systematic evaluation of the antimicrobial properties across varying concentrations of the neem-derived compounds.
2.3. RNA Extraction, Quality Assessment, and RT-PCR Analysis
RNA extraction was performed on both treated and untreated Enterococcus faecalis (ATCC 29,212) samples, harvested at 37°C for 24 hours, utilizing the RNXplus kit. The RNA was extracted from 60 treated samples (10 samples per fraction) and 10 untreated samples. Following extraction, the quality of the RNA was verified using Nanodrop. After purification, gene expression was evaluated through Reverse Transcription-Polymerase Chain Reaction (RT-PCR). This comprehensive approach ensured the thorough analysis of RNA from diverse treated and untreated samples, providing valuable insights into gene expression patterns. This gene expression analysis involved the use of specific primers and a one-step quantitative RT-PCR kit, as detailed in Tables 1 and 2. A comparative critical threshold real-time PCR method was employed to gauge the impact of plant extracts on the gene of interest. It's noteworthy that all experimental calculations were meticulously executed twice to ensure the reliability and consistency of the results.
Component | Volume (μl) |
---|---|
Primers (F and R) | 1 each |
2X Reaction Buffer | 2 |
Enzyme Solution | 1 |
RNA Extract | 3 |
Nuclease-free wate | 12 |
TOTAL | 20 |
Step | Temperature (°C) | Time |
---|---|---|
cDNA Synthesis at 50°C | 50 | 20 minutes |
Initial Activation Phase | 94 | 5 minutes |
Denaturation (40 cycles) | 94 | 25 seconds |
Annealing (40 cycles) | 63 | 60 seconds |
Extension (40 cycles) | 68 | 60 seconds |
Table 1 details the precise composition of the comprehensive reaction mixture employed in the gene expression analysis through reverse transcription-polymerase chain reaction (RT-PCR). The table provides specific volumes for components, including primers, 2X reaction buffer, enzyme solution, RNA extract, and nuclease-free water, totaling 20 μl.
In Table 2, the RT-PCR procedure steps and parameters are succinctly outlined. The cDNA synthesis occurs at 50°C for 20 minutes, followed by an initial activation phase at 94°C for 5 minutes. The denaturation step, consisting of 40 cycles, operates at 94°C for 25 seconds, while the annealing step at 63°C lasts for 60 seconds during each cycle. Finally, the extension step, also comprising 40 cycles, occurs at 68°C for 60 seconds per cycle. These procedural details provide a comprehensive guide for conducting the RT-PCR experiment.
2.4. Ethics
The corresponding author confirms that ethical considerations were taken into account in conducting this research, and they assert the article's originality with unpublished content. The research adhered to ethical principles rooted in the Declaration of Helsinki. The study protocol, along with the subject information and consent form, underwent review and approval by a local ethics committee, as indicated by document number DNE.151 (1/2/2023), to secure ethical clearance.
This study focused on the development of a plant-based solution for potential future use in treating human endodontic infections. While this study primarily dealt with experimental work on plant extracts and their effects on bacterial gene expression, it is crucial to note that this project aligns with broader ethical and regulatory considerations.
In this context, this study not only adhered to Helsinki guidelines but also obtained confirmation of compliance with the International Union for Conservation of Nature (IUCN) guidelines. These measures ensure the ethical conduct of research and the responsible use of natural resources.
3. RESULTS
3.1. Sub-inhibitory Concentration and Minimum Inhibitory Concentration (MIC)
The investigation into sub-inhibitory concentration and Minimum Inhibitory Concentration (MIC) for various fractions derived from Azadirachta indica yielded compelling results. Each fraction obtained from Azadirachta indica demonstrated notable antimicrobial properties, as evidenced by their ability to inhibit the growth of the target bacteria. Notably, Fraction 3 (Azadirachta indica-ethyl acetate) displayed its Minimal Inhibitory Concentration (MIC) at 30mg/ml, showcasing its effectiveness in inhibiting the growth of the targeted bacteria. On the other end of the spectrum, Fraction 6 (Azadirachta indica-water) exhibited a higher MIC of 50mg/ml, signifying the maximum concentration at which the growth of the target bacteria was significantly inhibited by the antimicrobial properties inherent in this particular fraction. The intricacies of these MIC values, illustrated in Fig. (1), contribute to a comprehensive understanding of the inhibitory capacities of distinct fractions of Azadirachta indica against the target bacteria.
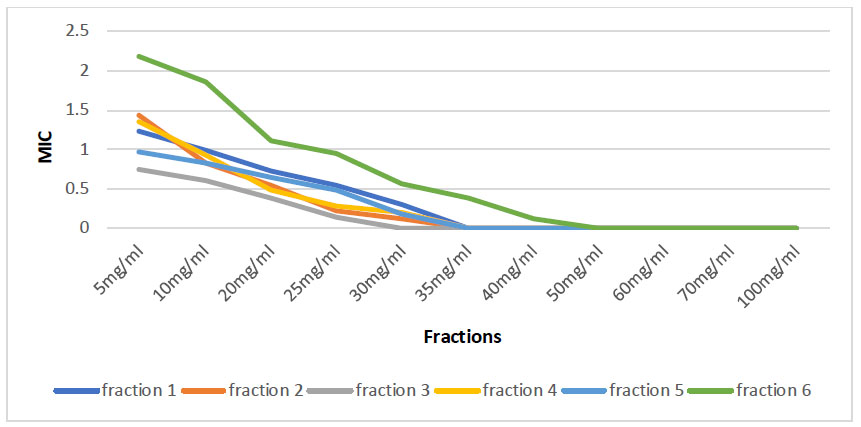
Parameter | F-1 | F-2 | F-3 | F-4 | F-5 | F-6 |
---|---|---|---|---|---|---|
Normalize change value | 0.517632 | 0.888843 | 0.239816 | 0.150726 | 0.139661 | 0.959264 |
Change fold | 0.95 | 0.17 | 2.06 | 2.73 | 2.84 | 0.06 |
3.2. Effect of Azadirachtolide Indica on Expression of esp Gene
Table 3 presents the impact of different Azadirachta indica fractions on the expression of the esp gene, revealing valuable insights into the modulation of gene expression levels. The parameters include Normalize Change Values and Change Fold for each fraction (F-1 to F-6). Notably, all fractions exhibited a significant normalized change value, suggesting a notable alteration in gene expression. Moreover, the change fold values provide a quantifiable measure of the fold change in gene expression, with Fraction 5 (F-5) showing the most substantial fold change at 2.84. Thus, Fraction 5 significantly decreased the expression of the target gene.
4. DISCUSSION
Enterococcus faecalis consistently emerges as the predominant or most frequently detected species in oral infections, playing a significant role in various conditions such as marginal periodontitis, infected root canals, and periradicular abscesses [64, 65]. Furthermore, E. faecalis is intricately linked to both primary, secondary, and persistent infections associated with periradicular disease, as highlighted in previous studies [19, 20]. Recognizing the challenges posed by E. faecalis, extensive research has delved into exploring various endodontic medicaments and irrigants aimed at removing or preventing the bacterium from gaining access to the root canal system during treatment [66, 67]. Notably, contemporary approaches have shifted towards exploring natural remedies over conventional bactericidal drugs, as evidenced by a previous work [68]. This paradigm shift underscores the evolving strategies in endodontic treatment, emphasizing the exploration of alternative, nature-based solutions to address the persistent challenges posed by Enterococcus faecalis.
Several studies have been dedicated to exploring the antimicrobial properties of plant-derived or natural products against Enterococcus faecalisand other species [69-72]. For instance, the study conducted by Kadhim et al. [41] delved into the antimicrobial effects of green tea against E. faecalis strains (ATCC 29212), and a study by Sopandani et al. [73] concluded that M. oleifera extract solution at concentrations of 75% and 100% is as effective as 5.25% NaOCl against Enterococcus faecalis. The findings of these investigations led to the conclusion that the plant extract could serve as a viable alternative to sodium hypochlorite and other solutions commonly employed in endodontic irrigation.
In a distinct approach, some studies have focused on determining the impact of extracted plant active substances on the virulence genes or quorum-sensing genes in pathogenic bacteria. Notably, a study in the northern part of Iraq investigated the effects of Liquorice and Azadirachta indica on various genes in E. faecalis [68]. Additionally, phytochemicals have been explored for their potential as antibiofilm agents against the mentioned bacteria. Another study reported that terpenes, phenylpropenes, flavonoids, curcuminoids, and alkaloids were effective in inhibiting the formation and/or eradicating E. faecalis biofilms [74]. This growing body of research underscores the diverse avenues being explored to harness the antimicrobial potential of natural products in the context of Enterococcus faecalis and suggests promising alternatives to traditional endodontic approaches.
The results of the present study elucidate the inhibitory potential of all fractions derived from Azadirachta indica on the (esp) virulence gene. The concentration range within which these fractions demonstrated inhibitory effects on the growth of the target bacteria varied, with (30mg/ml) of (Azadirachta indica-ethyl acetate) representing the lowest concentration inhibiting bacterial growth and (50 mg/ml) of (Azadirachta indica-water) constituting the highest concentration inhibiting bacterial proliferation. Remarkably, these findings align with prior research, which concluded that plant extracts exhibit inhibitory effects on the expression of virulence and biofilm genes [68, 75, 76]. It is noteworthy that this study stands as the first of its kind to specifically assess the impact of the esp gene in E. faecalis treated with various fractions of Azadirachta indica. In contrast, previous investigations focused on determining the expression of the same gene after treatment with juglone derivatives [77]. This novel contribution expands our understanding of the intricate molecular interactions between Azadirachta indica fractions and the virulence genes of E. faecalis, shedding light on potential paths for targeted therapeutic interventions in endodontic applications.
CONCLUSION
In conclusion, every fraction derived from Azadirachta indica demonstrated antimicrobial properties, with Fraction 3 (Azadirachta indica-ethyl acetate) exhibiting the lowest Minimum Inhibitory Concentration (MIC) at 30mg/ml, and Fraction 6 (Azadirachta indica-water) displaying the highest MIC at 50mg/ml against E. faecalis (ATCC 29,212). Real-time quantitative polymerase chain reaction (RT-qPCR) analysis revealed a consistent decrease in gene expression across all fractions. Fraction 5 showed the most significant fold change, suggesting a potential decrease in (esp) gene expression, which could be considered an alternative to classical irrigants.
LIST OF ABBREVIATIONS
Esp Gene | = Enterococcal Surface Protein |
A. Indica | = Azadirachta Indica |
E. Faecalis | = Enterococcus faecalis |
cDNA | = Complementary DNA |
ETHICAL STATEMENT
The study protocol, along with the subject information and consent form, underwent review and approval by a local ethics committee, as indicated by document number DNE.151 (1/2/2023), to secure ethical clearance.
This study not only adhered to Helsinki guidelines but also obtained confirmation of compliance with the International Union for Conservation of Nature (IUCN) guidelines.