Proportion of Streptococcus mutans, Streptococcus sanguinis, and Candida albicans in Early Childhood Caries: Evaluation by qPCR
Abstract
Background
Early childhood caries (ECC) is one of the most common diseases in children. It is associated with oral microbiome dysbiosis, characterized by a reduced beneficial oral microbiome and an increased pathogenic oral microbiome.
Objective
This study aims to detect and quantify S. mutans, S. sanguinis, and C. albicans in dental plaque samples from caries-free and ECC groups of children using the quantitative polymerase chain reaction (qPCR).
Materials and Methods
Supragingival plaque samples were collected from 30 children aged 2 to 5 divided into three groups: 15 caries-free children, 7 ECC, and 8 S-ECC. The abundance of microorganisms was determined by qPCR. The obtained data were analyzed using one-way ANOVA (post hoc Bonferroni) and the Pearson and Spearman correlation tests.
Results
The S-ECC group had a higher proportion of S. mutans and C. albicans. The proportion of S. sanguinis was higher in the ECC and had a positive correlation (P < 0.05) with S. mutans in this same group.
Conclusion
The proportion of S. mutans, S. sanguinis, and C. albicans differed between the caries-free, ECC, and S-ECC groups, and they correlated with each other in the occurrence of ECC.
1. INTRODUCTION
Early childhood caries (ECC) is the most common non-communicable disease and is a global endemic problem associated with socioeconomic status [1, 2]. The prevalence of ECC varies by country: for example, 21% in the United States, 72% in China, and 25% to 95% in the Southeast Asian countries [3].
According to the ecological plaque hypothesis, caries occur due to an imbalance of the oral microbiome (dysbiosis) in response to changes in environmental conditions in the oral cavity [4]. Oral microbiome dysbiosis is characterized by a loss of diversity, a reduced beneficial oral microbiome, and an increased pathogenic oral microbiome [5, 6].
Previous studies have revealed the role of Mutans streptococci, particularly S. mutans and Streptococcus sobrinus, in ECC's etiology and early pathogenesis [7, 8]. The proportion of S. mutans in the ECC biofilm can exceed 30% of a patient’s total oral bacteria, which indicates a significant S. mutans infection causing aggressive caries [8].
Current research on ECC reveals that Candida albicans (C. albicans) is involved in its etiology, indicating cross-kingdom interactions that are significant in the severity of ECC [7, 9, 10]. These synergistic interactions induce the expression of gtfsB and other genes, which causes an increase in carbohydrate metabolism by establishing an acidic environment (lowering pH) and leading to the proliferation of acidogenic-aciduric bacteria [11, 12].
S. mutans and Streptococcus sanguinis (S. sanguinis) have antagonistic interactions. S. sanguinis lowers S. mutans colonization, and more significant amounts of S. sanguinis have been found in caries-free children [13, 14]. However, other reports show different results, namely that S. sanguinis levels are higher in children with caries [8, 15, 16]. This discrepancy leads us to assume that the proportion of S. mutans, S. sanguinis, and C. albicans may differ between caries-free and ECC children, and the involvement of oral commensal microorganisms in ECC has yet to be fully explored.
The tooth surface is the site of caries occurrence; therefore, studying changes in the oral microbiome in supra-gingival plaque is important to understand the etiology of ECC [17]. This study uses quantitative real-time PCR (qPCR), as this molecular method not only detects the presence of the oral microbiome but can also be used to quantify specific species and bacterial populations [18].
This study aims to detect and quantify S. mutans, S. sanguinis, and C. albicans in dental plaque samples from caries-free, ECC, and S-ECC groups of children and then evaluate the relationship between these bacteria and fungi.
2. MATERIALS AND METHODS
2.1. Subjects and Sample Collection
This observational study was approved by the Dental Research Ethics Committee, Faculty of Dentistry Universitas Indonesia. The parents or caregivers provided written informed consent. The subjects in this study were children aged 2 to 5 years who visited the Integrated Service Post (Posyandu) in Gandaria Selatan, an urban village in Jakarta, Indonesia. The children had no systemic conditions and had not taken antibiotics in 3 months before the study.
One Pediatric Dentist performed the clinical examination. ECC was diagnosed using the American Academy of Pediatric Dentistry criteria [19]. Based on the results of clinical examinations, the children were divided into three groups: caries-free (no history of caries including white spot lesions), ECC (1-4 caries or white spot lesions, missing due caries and filled tooth), and severe early childhood caries (S-ECC) (> 4 caries missing due caries and filled tooth). Data collection was performed from March to May 2023.
2.2. DNA preparation and qPCR
Pooled supragingival plaque samples were collected from the buccal and labial surfaces of the upper and lower teeth with sterile cotton buds and stored in Eppendorf tubes containing 1 mL phosphate-buffer saline (PBS) (pH 7, 4) [20]. The obtained samples were immediately transported in a cooler box to the laboratory and stored at -80°C until undergoing further processing. DNA was extracted from the bacteria and fungi using a Zymo Quick-DNATM Miniprep Plus Kit, according to the manufacturer’s instructions [21]. The DNA’s content and purity were determined using Qubit (Invitrogen, Carlsbad, CA). The DNA was stored at -20 °C until further processing was needed. To quantify the DNA load, qPCR was performed with specific primers, as shown in Table 1.
Each sample was run in duplicate on AB StepOne Real-Time PCR System (Applied Biosystems, Foster City, CA, USA) equipment using HOT FIREPol EvaGreen qPCR Mix Plus (ROX) (Solis Biodyne, Tartu, Estonia). Twenty μL Standard PCR master mixes comprised 4 μL 5 x HOT FIREPol EvaGreen® qPCR Mix Plus, 1 μL forward primer, 1 μL reverse primer, 2 μL DNA template, and 12 μL NFW. The thermal cycling condition consisted of a 12-minute initial denaturation at 95 °C, followed by 40 PCR cycles of 15 s denaturation at 95 °C, annealing for 20 s at 60 °C, and elongation for 20 s at 72 °C [22].
The quantity of the targeted microorganisms in the sample was measured by extrapolating the Ct value of each species and the total bacteria to the log10 of each bacterium and fungi on a standard curve. A serial 10-fold dilution of extracted microorganism genomic DNA overnight culture was used to perform the standard curve [20].
Primers | Sequences | References |
---|---|---|
S. mutans | F: 5’-CCTACGGGAGGCAGCAGTAG-3’ R: 5’-CAACAGAGCTTTACGATCCGAAA-3’ |
[20] |
C. albicans | F: 5’-CACGACGGAGTTTCACAAGA-3’ R: 5’-CGATGGAAGTTTGAGGCAAT-3’ |
[20] |
S. sanguinis | F: 5’-CAAAATTGTTGCAAATCCAAAGG-3’ R: 5’-GCTATCGCTCCCTGTCTTTGA-3’ |
[23] |
Universal bacterial 16S rDNA | F: 5’-GTGSTGCAYGGYTGTCGTCA-3’ R: 5’-ACGTCRTCCMCACCTTCCTC-3’ |
[20] |
2.3. Statistical Analysis
SPSS 23.0 software (Microsoft Corporation, USA) was used to record and evaluate the data. The results of the normality test (Shapiro-Wilk) showed a normal distribution of data, so one-way ANOVA (P < 0.05) and post hoc Bonferroni was performed to compare the amounts of bacteria and fungi in each group. Pearson and Spearman correlation tests (P < 0.05) were performed to determine the linear associations.
3. RESULTS
The study included 30 children (14 boys and 16 girls), with 15 caries-free children, 7 ECC children, and 8 S-ECC children. The ages varied from 2.1 to 4.5 years.
3.1. Quantitative Levels of S. mutans, S. sanguinis, and C. albicans
All the plaque samples detected S. mutans, S. sanguinis, and C. albicans. By comparing the Ct values with the log of each bacterium and fungus, we could plot a standard curve to compute the amount of bacterium and fungus. These quantification findings are shown in Fig. (1). Quantity is the proportion of each bacterium to the total number of bacteria.
The proportion of S. mutans in the S-ECC (95.51%) was higher than in the CF (91.53%) and ECC (91.45%) groups (Fig. 1). However, the difference was not significant. The proportion of S. sanguinis in the ECC group (82.71%) was higher than in the CF (72.73%) and S-ECC (76.77%) groups, and there was a significant difference between the CF and ECC groups (p < 0.05). The highest proportion of C. albicans was found in the S-ECC (65.66%) group than in the CF (58.67%) and ECC groups (61.31%) groups, but it was not statistically significantly different.
3.2. Correlation between Bacteria and Fungi
We then investigated the possibility of a linear relationship between bacteria and fungi in children with CF, ECC, and S-ECC.
S. mutans had a negative correlation with S. sanguinis and a positive correlation with C. albicans in the CF group (Fig. 2A and B). S. sanguinis and C. albicans had a statistically significant moderate positive correlation (r = 0.65, p < 0.01) (Fig. 2C). In the ECC group, S. mutans and S. sanguinis had a significant and very strong positive correlation (r = 0.965, p < 0.05) (Fig. 2D), and C. albicans had a positive correlation with S. mutans and S. sanguinis (Fig. 2E and F). Meanwhile, in the S-ECC group, S. sanguinis positively correlated with S. mutans and C. albicans. A negative, insubstantial (p > 0.05) correlation was observed between S. mutans and C. albicans (Fig. 2G-I).
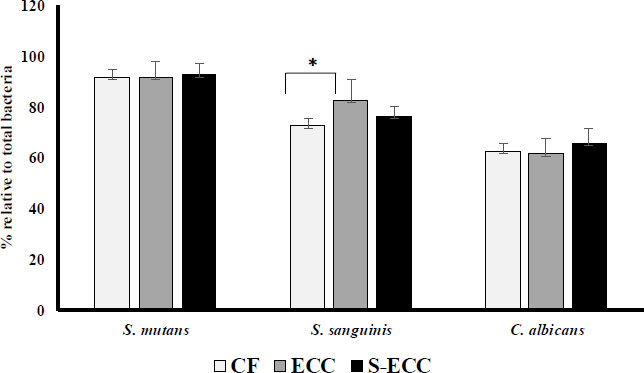
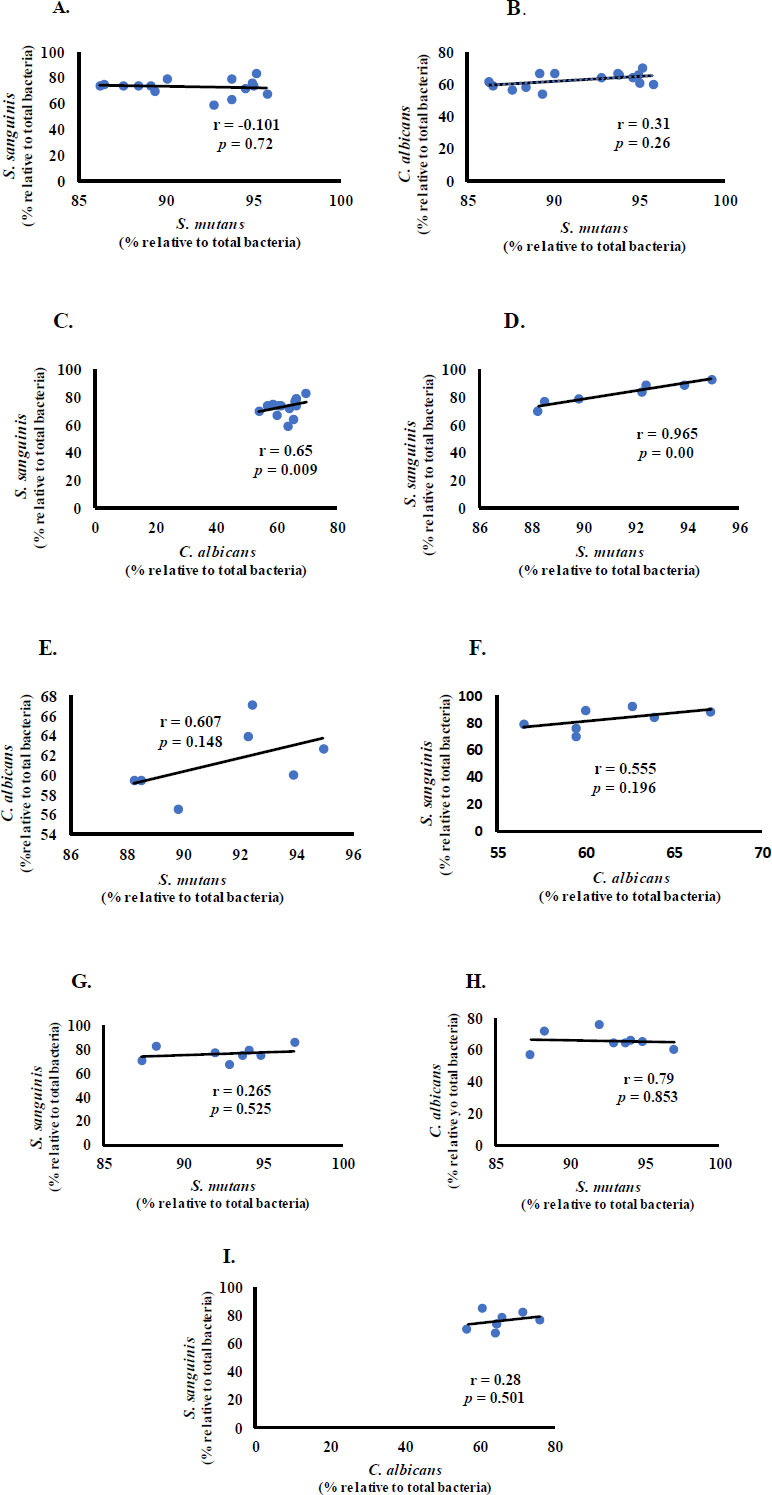
4. DISCUSSION
Our study found a significant difference between the proportion of S. sanguinis in CF and ECC groups. Furthermore, S. sanguinis was observed to have a very strong positive correlation with S. mutans in the ECC group. These commensal bacteria are associated with caries-free, healthy oral cavity conditions but can survive at a low pH, as found in subjects with ECC. However, when the environmental pH falls below the buffer capacity of saliva, the number of S. sanguinis will decrease [18, 24].
Our result is consistent with a previous study by Peterson et al., which revealed that S. sanguinis was associated with caries-free children but was over-represented in caries conditions. However, it is still unknown why S. sanguinis is more prevalent in children with ECC. The first possibility is that S. mutans and S. sanguinis have an antagonistic interaction. S. sanguinis is inhibited by bacteriocin, a product of S. mutans [18]. The high abundance of S. mutans produces more bacteriocin and inhibits the growth of S. sanguinis. This causes S. sanguinis to die in ECC. Since the qPCR method cannot differentiate between live and dead bacteria [18], we assumed that all the bacterium DNA from both live and dead cells might be detected.
Another possibility is that S. sanguinis cannot survive in mature plaque. S. sanguinis is not present in mature plaque because it colonizes early. According to Mitrakul et al., S. sanguinis was detected more frequently in early plaque (under 4 hours) than in mature plaque. Because the bacteria in plaque fluctuate with time, the sampling timing is critical [18].
The proportion of S. mutans in this study was equal between the groups (p > 0.05). This result is the same as in the study by Meriç et al., which reported the same levels of S. mutans between the two groups [8]. However, this differs from other research, indicating a higher level of S. mutans in ECC children than in caries-free [14, 20]. This study's findings support the hypothesis that S. mutans may not be the only indication of caries risk [8].
Although the amount of C. albicans found in the S-ECC group was higher than in the CF and ECC groups, the difference was not statistically significant. This finding is supported by previous research. C. albicans is always associated with S. mutans; their interaction induces gtfB gene expression and produces an environment suitable for ECC development [12, 20, 25]. This cross-kingdom interaction increases the severity of ECC [9, 11, 26, 27].
Discrepancies between the findings of this study and some prior research could be related to variances in geography, population, and diet. Children from different geographic regions and populations have different oral microbiomes due to their different lifestyles, which include dietary variance and genetic influences [28]. Additionally, other variables, such as fluoride, casein phosphopeptide-amorphous calcium phosphate, and biomimetic hydroxyapatite supplements, should be evaluated in future studies [29-31].
This research has limitations; it does not consider the pH of supragingival biofilm. Another limitation is that the qPCR method cannot distinguish live and dead cells, so the results should be confirmed using the plate count method. Despite its limitations, this study provides valuable information regarding caries in children aged 2 to 5 years, at least in our targeted groups, as well as the levels of S. mutans, S. sanguinis, and C. albicans and the involvement of S. sanguinis in cross-kingdom interaction.
CONCLUSION
The proportion of S. mutans, S. sanguinis, and C. albicans differed between the caries-free, ECC, and S-ECC groups, and they correlated with each other in the occurrence of ECC. S. sanguinis, as a commensal bacterium, is involved in the dynamic processes of caries in children due to the finding of a high proportion of S. sanguinis in ECC. Further research is needed that includes other ECC risk factors.
ABBREVIATIONS
ECC | = Early Childhood Caries |
qPCR | = Quantitative Polymerase Chain Reaction |
ETHICS APPROVAL AND CONSENT TO PARTICIPATE
This study obtained ethical approval from The Dental Research Ethics Committee Faculty of Dentistry Universtas Indonesia (Protocol number: 0713410220).
HUMAN AND ANIMAL RIGHTS
No animals were used in this research. All procedures performed in studies involving human participants were by the ethical standards of institutional and/or research committees and with the 1975 Declaration of Helsinki, as revised in 2013.