Bond Strength of Resin Cement Following Biomimetic Remineralization: An in vitro Study
Abstract
Objectives
This research assessed the efficacy of self-assembling peptide P11-4 (Curodont protect) in promoting biomimetic remineralization of dentin, thereby stabilizing the hybrid layer and enhancing the long-lasting of the resin-dentin bond.
Methods
Five premolar teeth were longitudinally sectioned to assess dentin microhardness before and after demineralization, as well as after the application of P11-4 (Curodont protect). For the microtensile test, ten premolar teeth were sectioned perpendicular to their long axes. The teeth were assigned to different groups. Following acid etching and rinsing, samples in control group I received no pretreatment, while the prepared dentin surface of group II was treated with 0.1 mL of P11-4 before the placement of the adhesive restoration. All specimens were stored in distilled water at 37 ± 2 °C for 24 hours and then underwent thermocycling. The microtensile test was measured, and the type of bond failure was evaluated. The obtained information, expressed in megapascals, was analyzed using a one-way analysis of variance (ANOVA), followed by Tukey’s multiple post hoc test.
Results
There was a statistically significant difference in hardness values after demineralization, followed by a significant increase in hardness after the application of P11-4 (Curodont protect) (p < 0.001, effect size = 0.985). No statistically significant difference was found in the microtensile bond strength between the two groups (p = 0.384, effect size = 0.582). Adhesive-type failure was more commonly observed.
Conclusion
The use of self-assembling peptide P11-4 (Curodont protect) on dentin resulted in higher microhardness than the control group without adversely affecting resin cement bond strength.
1. INTRODUCTION
Dentin is composed of many dentinal tubules, which, when exposed, can lead to severe, sharp pain. This issue commonly arises because of the cavity preparation and removal of the protective enamel layer. Dentin hypersensitivity is explained by hydrodynamic theory, which proposes that any external stimuli affect the opened dentinal tubules, causing movement of dentinal fluids and stimulating nerve endings, resulting in pain. Occlusion of open dentinal tubules through the remineralization process can effectively alleviate this problem and provide pain relief [1].
Numerous studies have been conducted to develop an ideal remineralizing agent capable of diffusing into the deep surface or delivering minerals [2]. The self-assembling peptide P11-4 (Curodont Protect) was prepared as a 3D scaffold for tissue engineering. This peptide can trigger biomimetic mineralization and repair mechanisms by diffusing into subsurface micropores and enhancing hydroxyapatite formation [3, 4]. It can be used as a desensitizing agent to occlude the open dentinal tubules and alleviate hypersensitivity [5, 6].
Furthermore, there has been a considerable rise in the need for aesthetic restorations. Adhesive systems have been expanded from bond restorative materials to dental tissues. The efficacy and goodness of these adhesive systems perform a crucial function in stabilizing a stable connection between the resin and dental structure [7].
Resin-based dental materials are widely used for restorative purposes, particularly in adhesive restorations, due to their superior mechanical and aesthetic character [8]. However, the presence of marginal gaps remains a significant challenge associated with resin-based restorative materials, as they can contribute to secondary caries to a greater extent [8].
Currently, there is limited knowledge regarding the impact of dentin remineralization using self-assembled peptide-containing materials on the integrity of resin cement bonds. Hence, this research aimed to examine the influence of self-assembling peptide P11-4 (Curodont protect), as a remineralizing agent, on dentin hardness and its effect on the microtensile bond strength of ceramic restorations cemented with total-etch adhesive resin cement. The null hypothesis assumes that dentin remineralization does not have a considerable influence on the quality of the resin cement bonds.
2. MATERIALS AND METHODS
The current study was performed on extracted premolar teeth for orthodontic reasons, which were inveterate, and they were appraised by the Research Ethical Committee (REC) of the Faculty of Dental Medicine for Girls, AL-Azhar University, under code (REC-PD-23-04). The written consent was obtained from the patients before extraction.
2.1. Sample Size Calculation
To investigate the remineralization potential of self-assembling peptide P11-4 (Curodont protect) on dentin, dentin samples were examined before and after acid etching and after the employment of P11-4. The influence of P11-4 on dentin microtensile bond strength was analyzed using an independent t-test to compare the control and P11-4 groups. To locate the sample size for the research, we referred to Pulidindi et al. (2022) [9] and utilized the G Power statistical power analysis program (version 3.1.9.4) [10]. According to the calculations, a total sample size of 10, divided equally into five participants in each group, was enough to detect a large effect size (d) of 2.14, with an actual power (1-β error) of 0.8 (80%), and a significance level (α error) of 0.05 (5%) for a two-sided hypothesis test.
2.2. Teeth Collection
Fifteen intact premolars, extracted for orthodontic reasons, were used for this research. Teeth with fractures, enamel malformations, or other defects were excluded from this research. Teeth were thoroughly scaled to remove calculus and remnants of periodontal tissue, then polished with pumice and soft rubber cups rotating at low speed under water coolant and stored in distilled water at 37 °C for less than one month until used in the experiment [11].
2.3. Preparation of the Specimens for Microhardness Test
The radicular portion of each premolar (n = 5) was separated, and the coronal portion was longitudinally cut into two equity in the mesiodistal orientation using a 0.3 mm thick diamond disc (Buehler, IL, USA) with water coolant. This process yielded ten dentin specimens. The specimens were then securely mounted in self-curing acrylic resin blocks using a particularly designed rectangular Teflon mold. The mold had a height, width, and length of 8 mm, 2 cm, and 2.8 cm. An acid-resistant nail varnish was used to protect the dentin surface, parting a window of exposed dentin measuring 4 × 6 mm [12].
2.4. Microhardness Test
The microhardness of all dentin samples was assessed at baseline using a Vickers microhardness tester (Wilson TukonTM1102, Buehler, Germany) equipped with a Vickers diamond indenter and 20X lens. The surface of each specimen was subjected to a 100-gram load for 10 seconds. Three indentations were made on the surface of each sample, evenly spaced within a circle with a 0.5 mm gap between the indentations. The lengths of the diagonals formed by indentations were measured using a built-in microscope. The Vickers hardness values were then converted to microhardness values. The Vickers hardness value (HV) was measured using the formula MHV = 1854.4L/d2, where the load L was in gf (grams-force) and the average diagonal length d was in μm [13]. Three readings were recorded for each sample, and the average was measured to obtain the Vickers hardness number (VHN).
The initial microhardness measurements were used as the control set for the samples. To induce demineralization, each sample was subjected to a 37% phosphoric acid gel (37%, Bisco, Schaumburg, IL, USA) for 15 seconds, followed by a 15-second rinse. The microhardness test was conducted again to assess the impact of demineralization on the dentin. Then, the samples underwent biomimetic remineralization by applying 0.1 mL of self-assembling peptide P11-4 (Curodont protect) (Switzerland Lot number 21-725.1). The entire prepared dentin surface was coated with P11-4, agitated using an applicator tip, and left to dry for 5 minutes [14]. Subsequently, a microhardness test was performed to evaluate changes in microhardness resulting from the biomimetic remineralization process [9].
2.5. Preparation of the Specimens for Microtensile Test (μTBS)
The occlusal thirds of the samples (n = 10) were taken off perpendicular to the long axis of the teeth with a diamond disc operating at a low speed with water cooling. Subsequently, the surfaces were smoothed using 600-, 800-, and 1200-grit sandpapers on a polishing machine to achieve flat dentin surfaces. The surfaces were then rinsed with water to remove debris [15]. Next, the dentin surfaces were examined under an optical microscope to confirm the absence of enamel and pulp tissue. The specimens were mounted in self-cure acrylic resin blocks and were randomly divided by stratified random allocation into two groups based on the treatment of the dentin surface:
2.6. Preparation of Ceramic Restoration
Lithium disilicate ceramic discs (E-max blocks) with a thickness of 4 mm were fabricated for each tooth sample to simulate an indirect restoration. The ceramic surfaces were etched with 9.5% hydrofluoric acid (HF) for 90 seconds using a porcelain etchant (Porcelain Etchant; Bisco, Schaumburg, IL, USA), followed by rinsing with water for 20 seconds, then air drying. A silane coupling agent (Porcelain Primer/Bis-Silane; Bisco, Schaumburg, IL, USA) was utilized on the ceramic surface using a brush. The application was conducted for 30 seconds to dry, following the manufacturer’s instructions [16].
2.7. Cementation Procedure
All samples were bonded using adhesive systems according to the instructions provided by the manufacturers.
In group I (the control group), the dentin surfaces (n = 5) were etched using 37% phosphoric acid (37%, Bisco, Schaumburg, IL, USA) for 15 seconds. Subsequently, the surfaces were rinsed with water for 15 seconds and gently air-dried. An adhesive (Bisco, Schaumburg, IL, USA) was applied and agitated for 15 seconds. Subsequently, the adhesive was gently air-dried for 10 seconds to allow for solvent evaporation. The adhesive was then light-cured for 20 seconds using an LED-curing unit (Elipar,3M ESPE, St Paul, Minnesota, USA) at an intensity of 1400mW/cm. Adhesive resin cement (BISCO, Duo-Link Universal U.S.A) was applied to the ceramic blocks, which were then bonded to the dentin surface under a pressure of 1 kg. Excess cement was removed, and light polymerization was performed for 2–5 seconds to ensure proper cement removal. Finally, light polymerization was performed for 20 seconds using an LED-curing unit (Elipar,3M ESPE, St Paul, Minnesota, USA) at an intensity of 1400mW/cm [16].
For group II, following the acid etching and rinsing step (similar to group I), the samples (n = 5) were treated with 0.1 mL of self-assembling peptide P11-4 (Curodont protect) remineralizing agent. The entire prepared dentin surface was coated with Curodont Protect, and agitation was performed using an applicator tip. The samples were dried for 5 minutes [13]. The bonding process and other cementation procedures were conducted in the same way as in group I. Subsequently, all samples were placed in distilled water at 37 ± 2 °C for 24 hours. Subsequently, the specimens underwent 500 cycles of thermocycling between temperatures of 5 °C and 55 °C, with each bath having a dwell time of 30 seconds [17].
2.8. Microtensile Bond Strength Test (μTBS)
Ceramic-dentin slabs with a cross-sectional area of approximately 1 mm2 were prepared by cutting the samples along the buccolingual and mesiodistal planes using a diamond disc in a low-speed micro-slicing machine (Isomet 4000 saw, Buehler, USA). Water cooling was employed during cutting to prevent overheating.
Four slabs were obtained from each tooth sample, and the mean value was calculated for each tooth. The slabs were then securely attached to a universal testing machine (Instron model 3345England) using cyanoacrylate adhesive. Tensile forces were applied to the slabs at a crosshead speed of 1 mm/min until failure. The data obtained from the tensile tests were recorded and calculated using the BlueHill Universal computer software (Instron, England).
2.9. Failure Mode Analysis
The fractured samples were carefully evaluated and documented using a stereomicroscope set at 30x (MA 100 Nikon stereomicroscope, Japan) equipped with Omnimet image analysis software. Photographs of the fractured surfaces were captured for further analysis.
The observed failure modes were categorized as follows:
2.9.1. Adhesive Failures
These failures occur at the interface between the adhesive and the dentin or ceramic. They may involve detachment at the dentin-adhesive or ceramic-adhesive interface.
2.9.2. Cohesive Failures
The term cohesive refers to failures within the adhesive or ceramic material. It also includes failures within dentin and non-specified cohesive failures.
2.9.3. Mixed Failures
Mixed failures are characterized by the presence of adhesive and cohesive failures on the same fractured surface. In other words, both adhesive and cohesive failure patterns were observed for the same specimen.
The different failure modes were carefully assessed and recorded according to these categories for further analysis [18].
2.10. Statistical Analysis
Numerical data were assessed for normality by examining their distribution and utilizing tests of normality, namely the Kolmogorov-Smirnov and Shapiro-Wilk tests. All data exhibited a normal (parametric) distribution. The data were presented as the mean and standard deviation (SD). The Student’s t-test was used to compare the microtensile bond strength between the two groups. A repeated measures ANOVA was conducted to compare the hardness values of Curodont before and after acid etching and fluoride application. In cases where the ANOVA test yielded a significant result, Bonferroni’s post hoc test was performed for pairwise comparisons. When the ANOVA test yielded a significant result, Bonferroni’s post hoc test was conducted for pairwise comparisons. The frequencies and percentages of the failure modes were presented as data. Fisher’s exact test was used to compare failure modes between the two groups. The significance level was set at p ≤ 0.05. Statistical analyses were performed using IBM SPSS Statistics for Windows (version 23.0; IBM Corp., Armonk, NY, USA).
3. RESULTS
3.1. Microhardness of Curodont (VHN)
There was a statistically significant change in the hardness values (p < 0.001, effect size = 0.985). Pairwise comparisons revealed a statistically significant decrease in hardness values after acid etching (demineralization), followed by a statistically significant increase in hardness after the application of Curodont Protect. However, the mean hardness after the application of Curodont was significantly lower than that before acid etching (Table 1, Fig. 1).
Before Acid Etching | After Acid Etching | After Curodont Application | p-value | Effect size (Partial eta squared) | |||
---|---|---|---|---|---|---|---|
Mean | SD | Mean | SD | Mean | SD | ||
50A | 3.8 | 29.6C | 3.7 | 43.4B | 3.7 | < 0.001* | 0.985 |
3.2. Microtensile Bond Strength (MPa)
There was no statistically significant difference in the bond strength of the ceramic discs cemented with resin cement between the control group (without Curodont application) and the remineralizing group (with Curodont application). The highest mean value was observed in the Curodont group (p = 0.384, effect size = 0.582). (Table 2 and Fig. 2).
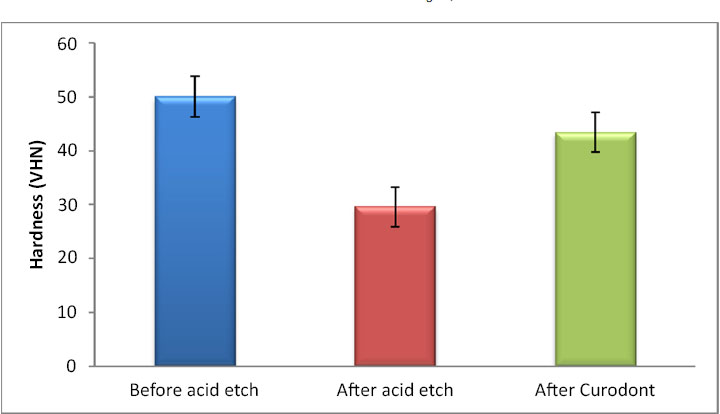
Table 2.
Curodont | Control | p-value | Effect Size (d) | ||
---|---|---|---|---|---|
Mean | SD | Mean | SD | ||
4.92 | 0.2 | 4.45 | 1.13 | 0.384 | 0.582 |
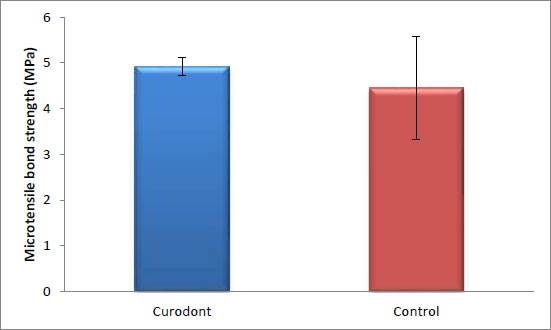
3.3. Failure Mode
There was no statistically significant difference between the failure modes of the two groups (p = 1, effect size = 0.258). Both groups exhibited three types of failures: adhesive, cohesive, and mixed. In the Curodont group, adhesive and cohesive failures were more prominent (40%) than mixed failures (20%). In contrast, in the control group, adhesive and mixed failures were more prominent (40%) than cohesive failures (20%). The percentage of adhesive failure was the same in both the Curodont and control groups (40%). (Table 3, Figs. 3 and 4).
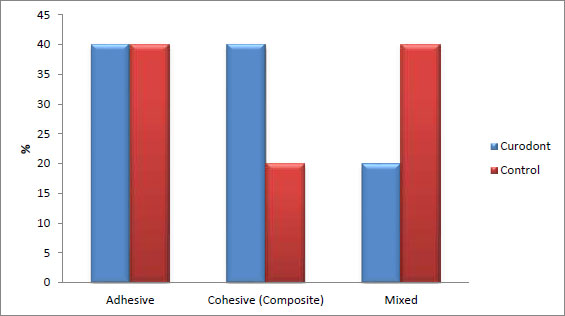
Failure Mode | Curodont | Control | p-value | Effect Size (v) | ||
---|---|---|---|---|---|---|
- | % | - | % | |||
Adhesive | - | 40 | - | 40 | 1 | 0.258 |
Cohesive (Composite) | - | 40 | - | 20 | ||
Mixed | - | 20 | - | 40 |
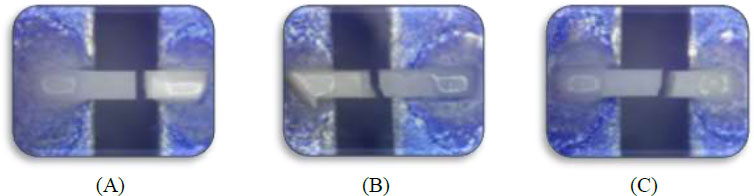
4. DISCUSSION
Management of dentin hypersensitivity, particularly after cavity preparation due to open dentinal tubules, poses a significant challenge in dental practice. The presence of strong chemicals or pressure from restorations can stimulate nerve endings by allowing fluid movement inside dentinal tubules. Such issues can be prevented by narrowing or occluding the dentinal tubules through remineralizing materials. These materials can interact with and seal the dentinal tubules, providing pain relief. An example of such a material is the self-assembly of peptides containing pastes [19].
Biomimetic mineralization is a technique developed to stimulate the formation of a layer of fluorapatite that looks like enamel. This could take place on the mineral substrate and has the ability to enhance remineralization of superficial enamel and demineralized dentin. This technique depends on the diffusion of calcium ions from a solution into a glycerin-enriched gel with phosphate and fluoride ions. When conditioning gel is in direct contact with the exposed tooth surface, a mineral layer firmly adherent to the surface of the tooth could be formed within eight hours [20].
The remineralization of dentin and the formation of a mineral layer inside the dentinal tubules may be a reliable approach for the reduction of dentin permeability to help in the treatment of postoperative hypersensitivity. The microhardness test was used to confirm remineralization [21].
Existing resin-composite restorative materials are inert gap fillings replacing lost tooth structures. Recently available dental materials can be used to repair or replace lost or diseased tissue, but they do not regenerate it [22].
Self-assembling peptide P11-4 (Curodont protect) can undergo self-assembly into three-dimensional (3D) fibrillar scaffolds in response to specific environmental conditions, such as a pH above 7 and variations in ionic strength [23]. The P11-4 peptide possesses an affinity for calcium ions, which triggers the precipitation of new hydroxyapatite, thereby promoting calcium and phosphorus deposition in hard tissues [3, 4].
In the current study, when examining dentin microhardness, there was a significant decrease in hardness values after acid etching, followed by a statistically significant increase in hardness after the application of P11-4 (Curodont protect) (Table 1 and Fig. 1). The observed increase in hardness may be due to the strong ability of the peptide to enhance biomimetic mineralization by nucleating hydroxyapatite crystals [24].
During the cementation process of indirect restorations, luting cement is applied at the interface between the tooth and the restoration, resulting in two distinct interfaces: restoration/cement interface and cement/tooth interface. Resin cement is considered the optimal choice for indirect cementation of ceramic restorations [25, 26]. Bonding between resin cements and indirect restorations is generally reliable in daily practice, as tooth conditioning methods are recommended to enhance bonding [27, 28]. However, the bond between the tooth structure and resin cement is the most crucial step in ensuring the long-term durability of the tooth/ restoration system [25, 26, 29]. Surface conditioning techniques, such as total etching, are commonly employed [30-32].
The effectiveness of the bonding of an adhesive system can be affected by many factors, including the structural, physical, and chemical characteristics of the substrate [33-35]. The substrate used here is not normal, but it is a remineralized dentin surface with a high mineral content. The bond strength between the resin cement and remineralized dentin is controversial.
Many research have reported that the mechanical properties of decalcified dentin can be enhanced by the application of remineralizing agents, which enhance the dentin resin bond strength [36-38]. The µTBS method was used because it provides a more uniform and homogeneous stress distribution during loading, and failure mainly occurs at the adhesive interface due to the small bonded interfaces (approximately 1 mm2) of the specimens used [39].
In the current study, there was no statistically significant difference in the bond strength between the control and self-assembling peptide P11-4 (Curodont protect) groups, with the highest mean value observed in the P11-4 (Curodont protect) (Table 2 and Fig. 2). P11-4, as a remineralizing agent, did not affect dentin resin bonding. It effectively interacted with demineralized dentin, enhancing remineralization and altering hydrophilicity without leaving any debris that could hinder bonding. This improvement in dentin microhardness is expected to positively impact the integrity and longevity of the dentin-resin bond interface [19].
However, it is worth noting that in studies conducted by Barbosa-Martins et al. in 2017 and 2018, self-assembling peptides were found to improve the bond strength of resin composites to both sound and caries-affected dentin. The use of Curodont Protect instead of Curodont Repair, as employed in this research, may have contributed to these differing findings [40, 41]. The difference between both products lies in their composition. Curodont Repair is a monomeric form of self-assembling peptide, which is usually applied clinically, while Curodont Protect is a polymeric form of self-assembling peptide containing 1000 ppm P11-4 and 900 ppm fluoride and calcium phosphate, designed for clinical or home use [40]. Additionally, both products differ in their primary mode of action, formula and concentration of P11-4; the monomeric form diffuses through the body of the lesion when applied to the surface of carious lesions, and the location of action of the polymeric form is primarily the tooth surface protecting the tooth minerals because the fibres are too long to dissipate into the lesion body [42].
The bond failures observed in this study predominantly exhibited adhesive and cohesive types, with fewer instances of mixed failures following dentin pretreatment with P11-4. In contrast, the control group had a higher occurrence of mixed failures and fewer cohesive failures (Table 3, Figs. 3 and 4). These findings differ from those of other studies, which reported an increase in adhesive and mixed failures accompanied by reduced adhesive bond strength after thermocycling [36, 38].
One possible explanation for these discrepancies is that previous studies compared different pretreatment agents with P11-4 rather than P11-4 with a control group. Variations in the pretreatment agents used may have contributed to the differences in bond failure modes and outcomes.
Furthermore, a limitation of this study was that a long-term estimation was not conducted to assess the interaction between the remineralizing agents and adhesive systems on microtensile bond strength (µTBS). Resin cement tested in this study also need to be evaluated in future studies in combination with products of different manufacturers and other remineralizing agents, such as fluoride, casein, phosphopeptide-amorphus calcium phosphate, and biomimetic hydroxyapatite in order to estimate their mutual effects.
Additionally, the use of a water storage medium may not precisely mimic the effects of dentinal fluid, intrapulpal pressure, or the function of endogenous dentinal enzymes in the degradation of resin cement. So, further clinical investigations are important to validate the current findings and to explore combinations of different strategies for durable dentin resin bonding.
The protocols for the application of remineralizing agents used in this research were considered straightforward and practical for clinicians as a preliminary step in adhesive procedures.
CONCLUSION
Under the limitations of this research, pre-treatment of demineralized dentin with self-assembling peptide P11-4 (Curodont protect) has been shown to be a hopeful and feasible treatment for relieving postoperative pain and enhancing dentin microhardness. This suggests that dentin pretreatment with remineralizing agents, such as the self-assembling peptide P11-4 (Curodont protect), maybe a viable option for enhancing the long-term durability of resin-dentin bonds.
LIST OF ABBREVIATIONS
HV | Hardness Value |
VHN | = Vickers Hardness Number |
HF | = Hydrofluoric Acid |
SD | = Standard Deviation |
μTBS | = Microtensile Bond Strength |
ETHICAL STATEMENT
The current study was performed on extracted premolar teeth for orthodontic reasons, which were inveterate, and they were appraised by the Research Ethical Committee (REC) of the Faculty of Dental Medicine for Girls, AL-Azhar University, under code: (REC-PD-23-04).
The Research Ethics Committee of the Faculty of Dental Medicine for Girls, Al-Azhar University, is constituted and operates according to ICH GCP guidelines and applicable local and Institutional regulations and guidelines that govern IRB operation.