Portland Cement: An Overview as a Root Repair Material: Applications and Various Modifications
Abstract
Background:
Portland cement has promisingly been utilized for the reconstruction of root perforation and bone defects, although its key drawbacks, including low mechanical properties and radiopacity as well as long setting time, necessitate pragmatic modifications.
Objective:
The main objective of this review was an overview of portland cement as a root repair material, its applications and various modifications.
Methods:
The electronic search of the literature was done on the Pubmed and Google Scholar databases with the keywords of Portland cement, carbon nanotube, graphene oxide, MTA, pulp capping, and root repair material.
Results:
The first part of this paper presents the data published in the literature on applications of Portland cement in endodontic situations, including vital pulp therapy, root perforation repair, root canal filling and root-end filling following apical endodontic surgery. This bioactive endodontic cement has shown promising success rates compared to mineral trioxide aggregate (MTA), however, considerable modifications are required in order to improve its clinical performance and expand its application scope as a root repair material. Hence, nano-reinforcements (graphene oxide, carbon nanotube, silica and hydroxyapatite) and extensive chemical modifications incorporated into Portland cement composition to produce innovative bio-dental materials with superior rheological properties have been discussed. Moreover, the current knowledge of the microstructure, mechanical properties and durability of nanomaterial-incorporated cement has been summarized. Ultimately, this article outlines the main points of animal and clinical studies on resin-modified Portland cement (TheraCal) as a pulp capping material and suggests further investigations prior to marketing authorization.
Conclusion:
It can be concluded that Portland cement has the potential to be used as an acceptable pulp capping material with the least complaints in the long term.
1. INTRODUCTION
Portland cement is extensively used worldwide as concrete for construction works and as a bioactive endodontic cement in dentistry. It consists of tri and dicalcium silicates, tricalcium aluminate, and tetracalcium alumino ferrite and calcium sulfate as gypsum [1, 2]. The introduction of bioactive cement is a significant breakthrough in the production of dental and bone cement for the reconstruction of root perforations or bone defects. Application of Portland cement in endodontics for vital pulp therapy or as an apical barrier in necrotic teeth has shown successful results compared to MTA and previously used materials such as amalgam, calcium hydroxide and glass ionomer [1, 2]. The first part of this review includes In vitro and in vivo studies conducted on Portland cement as a pulp capping material thus far.
Various Portland cement-based materials are available, although the drawbacks, including long-lasting setting time, low mechanical properties (compressive, tensile and flexural strength), poor resistance to microcracks and not acceptable radiopacity limit their application scope. According to the national standards, the initial setting time of Portland cement is about 1 h, and the final setting time is about 6.5 h [3]. With tremendous scientific effort, improvements have been made in the multi-functional properties of cementitious materials and their durability without compromising the biological properties [4]. Structural reinforcements and rheological modifications can be achieved by modifying cement with the optimum percentage of nanoscale materials such as metal mineral admixtures, metal oxides or carbon-based materials [5]. Several reports expressed a positive view on the inclusion of nanoparticles of alumina, titanium dioxide, nono-silica, graphene and graphene oxide, as well as carbon nanotubes and nanofibers in Portland cement to improve the microstructural behavior, increase the compressive strength and alter its setting time [6-9]. Nano-reinforcements act as a filler, which grants the cement a dense structure and hinders the initiation and propagation of microcracks when used in load-bearing areas [5]. Of note, the radiopacity of Portland cement is not enough to be visualized radiographically, therefore, radiopacifying agents are typically included in it. Bismuth oxide containing Portland cement has improved radiopacity and has been used under the name of MTA, although recent concerns such as cytotoxicity or tooth color alteration enforce using alternative materials, including zirconium oxide, niobium oxide and silver nanoparticles as radiopacifiers [10]. The modified Portland cement by radiopacifiers must meet certain requirements in order to be allowed to enter clinical testing.
Given that there is no comprehensive review of Portland cement and its applications, this paper includes Portland cement publications in the dental field and a cursory glance over material studies from peer-reviewed journals published in English and discusses miscellaneous modifications incorporated into cement's structure to improve its characteristics, microstructure and to facilitate its preparation and handling for dental procedures.
2. METHODS
The data search was completed using the Pubmed and Google Scholar databases with the keywords of Portland cement, carbon nanotube, graphene oxide, MTA, pulp capping, root repair material.
3. RESULTS
- The collected data showed that Portland cement has been applied in endodontic situations, including vital pulp therapy, root perforation repair, root canal filling and root-end filling following apical endodontic surgery with promising success rates compared to mineral trioxide aggregate (MTA).
- Modifications (nano-reinforcements and/or chemical modifications) are essential in order to improve the clinical performance of Portland cement.
- Some animal and clinical studies on resin-modified Portland cement (TheraCal) as a pulp capping material suggest further investigations prior to marketing authorization.
4. DISSCUSSION
4.1. Portland Cement Applications in Endodontics
4.1.1. Repair of Perforations
4.1.1.1. Animal Studies
Application of Portland cement to repair intraradicular perforations in dog premolar teeth showed acceptable sealing capacity due to the mineralized tissue formation, periradicular tissue regeneration and scarce inflammatory response in the perforated area [11]. Repairing perforation with calcium sulfate barrier and white, type ΙΙ or type ΙV Portland cement as an obturation material also resulted in high remineralization, and no difference was found between various materials in terms of newly formed bone [12, 13].
4.1.1.2. Clinical Studies
In a case report using Portland cement for sealing furcation perforation, Burges et al. reported no complaints after 9 years [14].
4.1.2. Root-end Filling
4.1.2.1. Clinical Studies
In a pilot study, Silva SR et al. compared MTA and Portland cement as root-end filling materials after endodontic surgery of maxillary and mandibular anterior teeth. Similar bone formation was obtained in both groups and periradicular regeneration occurred in all cases after six months [15].
4.1.3. Resorption
In a case report, Burges et al. found promising long-term results following the application of Portland cement for the treatment of a tooth with internal resorption and wide granulation tissue [14].
4.1.4. Pulp Capping
4.1.4.1. Animal Studies
Histological analysis of pulp tissues six months after the application of white and gray Portland cement and white and gray MTA for pulp capping of dog premolars showed no acute inflammation and the materials were equally effective in vascularization and fibrous tissue formation [16]. Reparative hard tissue formation following the application of Portland cement as a pulp capping material in baboon's premolar teeth was reported to be similar in thickness and quality to the MTA group, whereas significantly less and more incomplete hard tissue was detectable in the calcium hydroxide group [2]. The newly formed hard tissue seemed to be atubular and contained porosities and defects and bore no resemblance to secondary dentin in all groups evaluated.
In contrast, Shayegan et al. observed the formation of a complete thick layer of hard tissue 3 weeks after pulp capping of primary pig teeth with Portland cement, MTA, β-tricalcium phosphate and calcium hydroxide. Although some specimens in the calcium hydroxide group showed incomplete dentin bridge formation, given the normal pulp reaction, intact odontoblastic layer and absence of inflammatory response and bacteria, this study indicated the biocompatibility and regenerative ability of mentioned endodontic cement [17].
4.1.4.2. Clinical Studies
Short-term histological analysis of the effect of Portland cement as a pulp capping material on human third molars showed an inflammatory response in most cases, and Dentin Bridge was detected in only 10% of teeth. Twenty-one days after treatment, odontoblast-like cells were presented below the pulp tissue [18].
4.1.5. Pulpotomy
4.1.5.1. Animal Studies
Histological analysis of the dog's pulp tissue following pulpotomy using MTA and white and gray Portland cement showed that all materials were equally effective in preserving pulp vitality. After 4 months of follow-up, normal pulp tissue without inflammation or infection was observed [19, 20]. Another study by Holland et al. corroborated these results in a 60-day follow-up [21]. Comparison of Portland cement, MTA, β-TCP, ferric sulfate and formocresol effectiveness for pulpotomy in an animal study on pigs resulted in normal pulp tissue response in Portland cement, MTA and β-TCP groups, while acute inflammatory cells, internal resorption and necrotic areas were detected in ferric sulfate and formocresol groups [22].
4.1.5.2. Clinical Studies
Clinical studies on human primary teeth showed that Portland cement and MTA might serve as effective pulp capping materials [23, 24]. These materials yielded a success rate of 100% in 24-month follow-up clinically and radiographically, whereas the application of calcium hydroxide resulted in internal resorption and frequent necrotic areas [24]. Mineralized material deposition and the beneath healthy pulp tissue were detectable during the 6-month follow-up of teeth treated with Portland cement. Another 24-month follow-up study by Yildirim et al. reported 93.3% and 86.7% clinical and radiographic success rates in primary teeth treated with Portland cement for pulpotomy. Although no significant difference was between the Portland cement and MTA groups, internal resorption, periodontal space widening and furcation radiolucency were observed in the Portland cement group [25]. These results are in agreement with a study by Vilimek et al. [26]. In a similarly designed study, Sakai et al. found a success rate of 100% in teeth treated with Portland cement and MTA during 24-month follow-up. Notwithstanding, during the first 6 months, mineralized material deposition was detected in 100% and 57% of teeth treated with Portland cement and MTA, respectively [23]. Twelve-month follow-up of primary molars following conventional pulpotomy using Portland cement showed satisfactory results in preserving pulp vitality [27, 28]. The long-term success of human primary teeth pulpotomy using Portland cement and formocresol was also evaluated, and 100% of the success rate was achieved by Portland cement [29]. Pulpotomy treatment of human premolar teeth with Portland cement and MTA also showed no significant difference in the inflammatory response, soft tissue organization and dentin bridge formation [30].
Furthermore, the application of radiopaque Portland cement (containing zirconium oxide or iodoform) for pulpotomy of primary teeth showed no adverse effects in terms of clinical, radiographic or histological outcomes [31, 32].
4.1.6. Portland Cement as an Apical Barrier for Nonvital open Apex Teeth
In a case series study, Chakraborty et al. reported successful clinical outcomes after 6-month follow-up of 3 cases with nonvital and open apex conditions following the application of white Portland cement as an apical barrier [33]. Using white Portland cement apical plug with an absorbable collagen sponge barrier for apexification, De-Deus et al. reported clinical success and no signs of periapical rarefaction in a one-year follow-up [34].
4.1.7. Indirect Pulp Treatment
4.1.7.1. Clinical Studies
Application of Portland cement, MTA and calcium hydroxide for indirect pulp treatment showed a 90.3% success rate regardless of the used material. In 6-month follow-up, both Portland cement and MTA were found effective in limiting the infection and treatment of deep carious lesions [35].
4.1.8. Portland Cement as an Intracanal Medicament
4.1.8.1. In-vitro Studies
The intracanal application of calcium silicate-based cement (white Portland cement, white ProRoot MTA and Biodentine) with chlorhexidine as a vehicle showed higher calcium ion release compared to calcium hydroxide during the 14 days period. The addition of 2% chlorhexidine extended the setting time up to 84 days and consequently facilitated the removal of medicament [36].
4.1.9. Treatment of Hypersensitivity
Treatment of dentin hypersensitivity using a calcium silicate paste based on Portland cement occluded dentinal tubules by the formation of a fine crystalline layer and demonstrated the ability of this material to reduce dentin permeability. SEM/EDX analyses revealed the precipitate deposits and formation of a calcium-reach layer on the dentin surface, suggesting the clinical use of Portland cement as a desensitizing agent [37].
4.1.10. Portland Cement in Bone Tissue Engineering
Calcium silicate-based materials are a potential candidate for bone tissue engineering due to their good physical properties, biocompatibility and acceptable bioactivity and osteoconductivity [38]. Portland cement stimulates the expression of bone remodeling markers and the growth of bone tissue with the formation of osteoid and new trabeculla [39, 40]. The behavior of osteoblast lineage cultured on Portland cement has been previously discussed. Three-dimensional porous Portland cement-based scaffolds with high mechanical strength compatible with that of normal bone have been proposed. These scaffolds support human osteoblast cell adhesion and proliferation [41, 42]. Portland cement scaffold modified with polydimethylsiloxane enhanced cell metabolism and alkaline phosphatase activity after 2 weeks [42].
Portland cement is well-suited for bone repairing in load-bearing areas such as vertebrae or mandible. In order to produce injectable bone cement using Portland cement with accelerated setting time, studies introduced liquefying additives into it [43]. Modification of Portland cement with 2 wt% sodium or potassium citrate improved its injectability and reduced the setting time while increasing the compressive strength [44]. It is worth mentioning that at low concentrations, citrate acts as a Portland cement retardant by slowing down the dissolution of alite and aluminate, although, in high concentrations, it accelerates the hydration process [45, 46]. Other additives, such as calcium chloride and calcium nitrate, are also used for modifying Portland cement injectability whilst maintaining its compressive strength [47].
Coating CaCO3 on cement by carbonation is a feasible way to enhance the biocompatibility and bioactivity of Portland cement. Exposure of carbonated Portland cement to calcium phosphate solution resulted in the precipitation of apatite-like crystals (carbonated apatite), which strongly resemble natural bone structure [48].
Polymethyl methacrylate bone cement containing hydrated and anhydrous white Portland cement as a filler also showed satisfying apatite formation ability. The formed apatite layer was denser, and pH value was higher in hydrated Portland cement [49].
5. WHITE PORTLAND CEMENT
Although various characteristics of white Portland cement have been extensively discussed previously, a brief review of the composition of this material would be worthwhile. The grayish color of Portland cement led to the introduction of modified Portland cement named white Portland cement to match the tooth color. The color change from gray to white is due to significantly fewer chromospheres, predominantly iron, manganese, chromium and titanium in white brands [50]. White and gray Portland cement and white and gray MTA are composed of the same major components [51]. White Portland cement is made of high-grade limestone with less than 0.15 wt% Fe2O3 and 0.015 wt% MnO and white clay and other products with less than 1 wt% FeO and 0.8 wt% TiO2 [52]. A comparison of type Ι white and gray Portland cement showed that white cement has more dicalcium silicate and aluminate and trace of tetracalcium aluminoferrite [50]. SEM analysis of white Portland cement showed various amorphous, globular and crystalline particles dispersed over ground with finer particles [53].
6. MODIFICATION OF PORTLAND CEMENT WITH RADIOPACIFIERS
Portland cement is slightly radiopaque (0.86-2.02 mm Al) in the natural state; thus, it does not meet the International Organization for Standardization (ISO 6876) requirements. Literature has introduced various radiopacifiers, including zinc oxide, zirconium oxide, titanium dioxide, barium sulfate, iodoform, bismuth oxide, calcium tungstate and ytterbium trifluoride to allow the distinction of Portland cement from adjacent dentin and anatomical structures, although it is uncertain if favorable properties would be satisfied (Table 1) [54, 55]. Thus far, Portland cement/lead oxide and Portland cement/bismuth oxide have shown the highest radiopacity values, while Portland cement/zinc oxide showed the lowest values of radiopacity. Nevertheless, all of them exhibited higher radiopacity values than dentin, and therefore they can be potentially added to Portland cement as radiopacifier agents [56]. It has been reported that all materials containing Portland cement and radiopacifiers promote pH values similar to pure Portland cement and release calcium ions gradually [55, 57].
Refs. | Study Type | Type of PC | Radiopacifier | Other Groups | Obtained Results |
---|---|---|---|---|---|
Bortoluzzi et al. [181] |
Animal study | WPC | 20% Bi2O3 | MTA + 20% BaSO4 MTA + 20% Bi2O3 MTA ProRoot |
Modified PC showed a higher inflammatory response with no necrosis area during 60 days of implantation, no difference was between groups in reparative tissue |
Li et al. [65] |
In vitro | WPC | 20% Bi2O3 20% ZrO2 |
White PC | Bi2O3 extended initial and final setting times and retarded the hydration degree ZrO2 did not affect the setting time and accelerated the hydration degree |
Sabari et al. [90] |
Animal study | WPC | 20% Bi2O3 20% ZrO2 20% Iodoform |
MTA | Similar tissue reactions were observed among all groups during 60 days |
Souza et al. [87] |
In vitro | PC | 2%, 5%, 10%, 15% (BiO)2CO3 | MTA Angelus | (BiO)2CO3 increased the setting time, all groups had similar solubility, PC+15% (BiO)2CO3 showed radiopacity similar to MTA, acceptable biological properties in all groups were observed |
Mestieri et al. [182] |
In vitro | WPC | Nb2O5 µP Nb2O5 nP |
MTA | Similar or higher cytocompatibility and bioactivity compared to MTA were observed |
Flores et al. [183] |
In vitro | WPC+10,20,30% wallostonite WPC+10,20,30% BG |
20% Bi2O3 | MTA Angelus | Addition of wollastonite and BG reduced the setting time and radiopacity, acceptable solubility and physical properties were observed compared to MTA |
Vazquez et al. [184] |
In vitro | PC | 30% ZrO2 AgNPs |
WMTA WMTA + AgNPs |
Addition of AgNPs favored the physicochemical and mechanical properties and increased the antibacterial activity |
Guerreiro et al. [142] |
In vitro | PC+ 10, 20% nHA | 30% ZrO2 | WMTA | Addition of nHA improved the antibacterial activity and setting time but harmed the mechanical properties and solubility |
Slompo et al. [185] |
In vitro | WPC | 20% ZrO2 | WMTA | Low cytotoxicity was observed |
Antonijevic et al. [81] |
In vitro | PC | 30% YbF3 | CSC+30% YbF3+40%CaCO3 CSC+30% YbF3+35% nHA |
PC+ YbF3 had the most radiopacity, setting time and fluoride release with acceptable biocompatibility and micromechanical properties |
Bosso et al. [55] |
In vitro | Type ΙΙ PC(CSC) Resinous CSC |
ZrO2 nP ZrO2 µP Bi2O3 Nb2O5 CaWO4 |
WMTA | CSC+ ZrO2 µP, Nb2O5 and CaWO4 had similar results to WMTA with shorter setting time |
Tanomaru et al. [83] |
In vitro | PC + 10%, 20% CaO | 30% ZrO2 30% Nb2O5 |
WMTA | PC+ZrO2+ 20%CaO had a setting time similar to WMTA but with lesser compressive strength |
Mestieri et al. [85] |
In vitro | PC | 30% Nb2O5 nP 30% Nb2O5 µP |
WMTA | MTA had more radiopacity, but OPC had greater cell viability |
Guerreiro et al. [76] |
In vitro | PC PC+5%ZnO PC+10%ZnO |
ZrO2 | - | Addition of ZnO decreased the compressive strength, all materials had similar antibacterial activity |
Marciano et al. [186] |
In vitro | PC | 20% ZrO2 20% CaWO4 |
WMTA | Bovine teeth filled with all materials showed color alteration after 60 days |
Viapiana et al. [82] |
In vitro | PC+ epoxy resin | ZrO2 nP, µP Nb2O5 nP, µP |
AH Plus MTA Fillapex |
OPC showed radiopacity similar to MTA and had the highest Ca release and crystalline deposition |
Tanomaru et al. [10] |
In vitro | WPC | ZrO2 nP, µP Nb2O5 nP, µP |
WMTA | MTA had the most radiopacity, all materials had antibacterial activity |
Chen et al. [97] |
In vitro | PC | Bi2O3+0, 15, 30, 100% YSZ | - | PC+ Bi2O3+ 15%YSZ had the most radiopacity but similar cell viability to PC+ Bi2O3 |
Viapiana et al. [187] |
In vitro | PC+ epoxy-based vehicle | ZrO2 nP, µP Nb2O5 nP, µP |
AH Plus | All sealers had great sealing ability, favorable overall characteristics were observed compared to AH Plus |
Antonijevic et al. [67] |
In vitro | PC | 10, 20, 30% ZrO2 10, 20, 30% Bi2O3 10, 20, 30% YbF3 |
ProRoot MTA | Bi2O3 decreased the compressive strength of PC but ZrO2 and YbF3 increased it, Bi2O3 extended the setting time but ZrO2 and YbF3 did not affect it |
Viapiana et al. [86] |
In vitro | PC | ZrO2 nP, µP Nb2O5 nP, µP |
AH Plus MTA Fillapex MTA Sealapex |
PC+ ZrO2 µP and PC+ Nb2O5 nP had setting time similar to Fillapex, AH Plus had the most compressive strength, OPC had acceptable solubility but the least radiopacity |
Weckwerth et al. [71] |
In vitro | WPC | Bi2O3 (BiO)2CO3 Bi5H9N4O22 ZrO2 |
- | Addition of (BiO)2CO3 and Bi5H9N4O22 increased the solubility, no antibacterial activity but acceptable antifungal activity was observed |
Coleman et al. [188] |
In vitro | WPC | ZrO2 | - | ZrO2 reduced the initial and final setting time of PC and had a slight effect on hydration behavior |
Guerreiro et al. [57] |
In vitro | PC | ZrO2 Bi2O3 CaWO4 |
ZOE | All OPC compositions had great antibacterial activity |
Duarte et al. [66] |
In vitro | WPC | ZrO2 Bi2O3 CaWO4 |
WMTA | WMTA had the least setting time, all radiopacifiers increased PC's setting time, all materials had alkaline pH and released Ca |
Formosa et al. [73] |
In vitro | PC | 20% Bi2O3 | TCS TCS+20% Bi2O3 |
Bi2O3 increased the setting time, OPC had acceptable compressive strength when cured in distilled water |
Cutajar et al. [78] |
In vitro | PC | 0-50% ZrO2 | ProRoot MTA | Addition of 30% ZrO2 showed great physicochemical and mechanical properties |
Cornelio et al. [92] |
In vitro | PC | ZrO2 Bi2O3 CaWO4 |
ZOE | High biocompatibility was observed |
Camilleri et al. [91] |
In vitro | PC | BaSO4 Au Ag/Sn alloy |
MTA Intermediate restorative material |
OPC had extended setting time compared to PC, but similar compressive strength was observed PC+Au had setting time and compressive strength similar to MTA |
Vivan et al. [72] |
In vitro | PC clinker | CaSO4 Bi2O3 |
MTA Angelus MTA Bio Sealepox Light-cured MTA |
Radiopacity of all materials was acceptable except for light-cured MTA |
Min et al. [98] |
In vitro | PC | Bi2O3 | Pure PC | No difference was observed in the mineralization of hDPCs in PC and OPC groups |
Bortoluzzi et al. [189] |
In vitro | WPC | Bi2O3 BaSO4 Iodoform |
Pure PC WMTA |
All materials had acceptable radiopacity except for PC and PC+ BaSO4 |
Duarte et al. [56] |
In vitro | PC | (BiO)2CO3, Bi2O3 Bi5H9N4O22 BaSO4, ZnO, PbO ZrO2, CaWO4 Iodoform |
Pure PC | All OPC compositions had acceptable radiopacity |
Saliba et al. [70] |
In vitro | WPC | 10, 15, 20, 25,30% Bi2O3 | WPC | Addition of Bi2O3 did not affect the compressive strength of PC |
Hwang et al. [94] |
In vitro | PC | Bi2O3 | MTA | OPC had radiopacity similar to MTA, MTA had higher cell viability than OPC |
Bueno et al. [63] |
In vitro | WPC | Bi2O3 | WMTA | MTA and PC+15% Bi2O3 had similar radiopacity |
Coutinho et al. [60] |
Animal study | PC | Bi2O3 | ProRoot MTA | All materials were biocompatible during 60 days |
Kim et al. [190] |
In vitro | PC | Bi2O3 | - | PC+20% Bi2O3 had the most radiopacity and similar cell viability to PC+ less concentrations of Bi2O3 |
6.1. Bismuth Oxide
MTA is predominantly composed of Portland cement with the addition of bismuth oxide as a radiopacifier [58]. Addition of various percentages of bismuth oxide up to 50% to Portland cement did not influence the alkaline pH of the cement [59]. Moreover, Portland cement radiopacity has a correlation with the concentration of incorporated bismuth oxide, although the cytotoxicity issues must be considered [60]. Radiopacity of white Portland cement with bismuth oxide (20%) does not show a significant difference with that of aluminum with 4 mm thickness, which is the ideal value according to the American National Standard Institute/American Dental Association (ANSI/ADA) recommendations [56, 61, 62]. Bueno et al. reported that the ideal concentration of bismuth oxide to provide radiopacity similar to MTA is 15% [63].
It is noteworthy that the addition of bismuth oxide seems to compromise the physical properties of the material. The particle size of bismuth oxide also affects these properties as reducing the particle size of bismuth oxide increases the diametral tensile strength significantly [64]. Bismuth oxide increases the porosity of Portland cement and consequently reduces the compressive strength and extends the setting time [65-67]. Comparing the influence of the addition of various radiopacifiers to Portland cement on its compressive strength showed that only the incorporation of bismuth oxide resulted in lower compressive strength [68]. Nevertheless, in this study radiopaque Portland cement had reduced initial and final setting times. Bismuth oxide concentration has a negative correlation with mechanical strength and increases the relative porosity of set material [69]. In contrast, Saliba et al. showed that Portland cement's compressive strength was not affected by the addition of various percentages of bismuth oxide [70]. According to Bosso-Martelo et al., amongst various radiopacifiers only bismuth oxide did not affect the final setting time of Portland cement, although it was significantly more than the setting time of MTA Angelus [55].
Furthermore, there are conflicting results regarding the impact of bismuth oxide on the solubility of Portland cement. According to Weckwerth et al., bismuth oxide affects this property adversely [71], whereas some studies indicated that radiopaque Portland cement exhibits less mass loss following immersion in water compared to MTA [66, 72]. It should be taken into consideration that the curing and experiment conditions affect the physical properties of cement significantly. For instance, bismuth oxide modified Portland cement has higher compressive strength when immersed in distilled water, although it shows lower compressive strength in Hank's balanced salt solution (HBSS) [73].
6.2. Zirconium Oxide
Studies have shown that the fabrication of Portland cement associated with zirconium oxide as a radiopacifier might be preferred to bismuth oxide since no significant adverse effect was detected concerning the physical properties such as compressive strength and solubility and biological activities [66-68]. Some studies reported similar radiopacity for Portland cement containing zirconium oxide micro or nanoparticles and Portland cement containing bismuth oxide [55, 74]. Studies showed that the inclusion of 30 wt% zirconium oxide to Portland cement results in acceptable compressive strength without compromised hydraulic properties, suggesting zirconium oxide as a potential alternative to bismuth oxide [74-77]. Furthermore, a combination of 30 wt% zirconium oxide and Portland cement promotes its radiopacity, setting time and solubility similar to ProRoot MTA [78]. The same results were achieved by Bosso-Martelo et al. [55]. In contrast, a longer setting time was observed by Duarte et al. following the addition of 20 wt% zirconium oxide to white Portland cement with a considerable difference to white MTA, which seems to be related to the amount of calcium sulfate in this material [66]. This study reported limited calcium release from radiopaque Portland cement (containing zirconium oxide, bismuth oxide or calcium tungstate) compared to pure white Portland cement, however, it was not different from the calcium release of white MTA in the first hours. Zirconium oxide does not interfere with hydration by-products of Portland cement and acts as an inert filler. Moreover, it provides efficient nucleation sites for the precipitation and growth of the calcium-silicate-hydrate (C-S-H) gel phase resulting in an increase in hydration degree by 26% [65, 75-79]. SEM evaluation of zirconium oxide modified Portland cement revealed free zirconium oxide particles distributed throughout the cement [75]. In agreement with these studies, Li et al. reported that zirconium oxide did not participate in chemical reactions of Portland cement hydration and decreased the solubility of cement without affecting its calcium release capacity [80].
6.3. Ytterbium Trifluoride
Ytterbium trifluoride seems to be a potential radiopacifier to be incorporated into Portland cement with no significant adverse effect on its mechanical and biological properties [81]. The addition of 20 wt% ytterbium trifluoride resulted in a radiopacity value equal to 3 mm Al cut-off and promoted the compressive strength of Portland cement [67]. Incorporation of fluoride in Portland cement and long-term sustained release of it might have beneficial effects on the prevention of caries and improvement of osteogenesis, dentinogenesis (to enhance apexogenesis and apexification) and mechanical properties of dentin by the formation of fluorapatite. However, it is worth mentioning that, excessive fluoride accumulation interferes with hard tissue formation and might cause cytotoxic issues for root-end filling material. Noticeable release of Yb and F ions from ytterbium trifluoride containing Portland cement leads to an increased risk of cytotoxicity [81]. Notably, the low water solubility of ytterbium trifluoride may limit the long-term degradation of dental cement. This is of particular importance in preserving the structural integrity of the tooth for treatment success.
6.4. Niobium Oxide
Another alternative for bismuth oxide considered in the literature is niobium oxide micro and nanoparticles [10, 82, 83]. Recent researches also showed that niobium might stimulate hydroxyapatite deposition. Addition of microparticulate and nanoparticulate niobium oxide to Portland cement results in a material with satisfactory physicochemical and biological characteristics in terms of setting time and radiopacity and provides an alkaline environment. This study also indicated that the particle size did not have a significant effect on the physicochemical and biological properties of the cement [84]. Although higher radiopacity was demonstrated by MTA compared to Portland cement containing niobium oxide, greater cell viability and alkaline phosphatase activity were detected by this combination [85]. Niobium oxide modified Portland cement showed setting time, flowability, compressive strength and solubility adequate for clinical applications [86].
6.5. Other Radiopacifiers
Other radiopacifiers, such as 15% bismuth carbonate and calcium tungstate, have been incorporated into Portland cement and showed adequate pH level, calcium release and radiopacity similar to MTA [66, 87]. The addition of 15% bismuth carbonate to Portland cement did not influence its solubility but increased the setting time [87]. Studies have demonstrated that Portland cement containing 20% iodoform shows sufficient radiopacity to address the current regulatory standards for root filling materials [88, 89]. Other radiopacifiers, including lead oxide, bismuth subnitrate, tantalum oxide and barium sulfate, have been incorporated into Portland cement with higher radiopacity than dentin or pure Portland cement [56, 77, 90]. Furthermore, addition of barium sulfate or silver alloy exhibited acceptable radiopacity and induced a PH increase. Although these materials extended the setting time, no adverse effect was observed regarding the compressive strength [91].
6.6. Biocompatibility of Portland Cement with Different Radiopacifiers
6.6.1. In vitro Studies
The aforementioned additives might influence the biocompatibility of cement and interfere with the healing of the periapical tissues. In a biological point of view, radiopacifying agents with excellent safety profiles and good biocompatibility are commonly chosen. Cell culture studies, in addition to morphologic analysis, reported no cytotoxicity of white Portland cement containing bismuth oxide, zirconium oxide and calcium tungstate against murine periodontal ligament cells and rat osteosarcoma cells up to a concentration of 100 mg/ml. Although the presence of heavy metals in higher concentrations can promote necrosis cell death due to oxidative stress [92]. White Portland cement containing zirconium oxide or zinc oxide exhibited acceptable cell viability and increased ALP activity of hDPSCs during a 21 days period [93].
Some previous studies found similar bioactivity, cytotoxicity, genotoxicity and tissue reaction among pure Portland cement, bismuth oxide containing Portland cement and MTA [94-96]. The addition of various amounts of bismuth oxide to Portland cement up to 50% does not affect its biocompatibility, although the concentration of 15% bismuth oxide shows a significant decrease in the inflammatory response [59]. A modified Bi2O3-radiopacifier (zirconia doped bismuth oxide) was incorporated into Portland cement by Chen et al. in order to improve the radiopacity of the material to approximately 6 mm Al. The desirable biocompatibility of this compound satisfies the requirements for a potential root filling material [97].
Furthermore, according to Min et al., radiopaque and pure Portland cement have similar effects on the mineralization of hDPCs [98]. This In vitro study observed well-spread hDPSCs with cellular extensions in close contact with radiopaque Portland cement in addition to increased ALP activity and high expression of osteogenic markers (osteonectin and dentin sialophosphoprotein). Evaluation of cytotoxicity of 15% bismuth carbonate-containing Portland cement on human periodontal ligament fibroblasts (hPLFs) showed noticeable biocompatibility [87]. Further assessment of the expression of bone formation genes (ALP, COL1 and RUNX2) and pro-inflammatory cytokines (IL-1A, IL-6, IL-8 and TNF) resulted in similar findings to MTA [87].
Regarding the addition of Ytterbium trifluoride to Portland cement, In vitro studies have reported no adverse effect on the differentiation of bone marrow mesenchymal stem cells and the morphology of osteoblasts and osteoclasts following cell culture. Furthermore, numerous cell extensions were detected, implying appropriate cell spreading and adherence to the substrate [81].
6.6.2. Animal Studies
Although In vitro studies have shown satisfying bioactivity of radiopaque Portland cement, in an animal study, Dreger et al. observed that MTA is more effective in promoting biomineralization in the dentin-cement interface and the intratubular space. This study reported more rapid and intense mineral deposition in MTA cases compared to Portland cement with 20% bismuth oxide [99].
Implantation of Portland cement associated with radiopacifiers, including iodoform or zirconium oxide, into the dorsal connective tissue of rats also showed no significant difference in the inflammatory response from pure Portland cement, however, morphological analyses revealed less inflammation in radiopaque groups [88]. In a similarly designed study, no difference in terms of tissue reaction was detected between Portland cement containing 20% iodoform or zirconium oxide or bismuth oxide and MTA [90]. Adversely, Silva et al. demonstrated that radiopacifier agents induced inflammatory reactions significantly, and this increase was higher in Portland cement containing bismuth oxide in comparison with Portland cement with zirconium oxide [74].
7. MODIFICATION OF PORTLAND CEMENT WITH CALCIUM CHLORIDE
Incorporation of calcium chloride up to 15% changes the surface morphology of white Portland cement and results in a more homogenous surface with less porosity which reduces bacterial contamination and favors biological properties [53]. Furthermore, it reduces the setting time of Portland cement and MTA by altering the kinetics of tricalcium silicate hydration [100], improves the sealing ability [101] and push-out strength [102], increases PH [103], reduces the solubility [103] and does not affect the dimensional stability [104]. Moreover, calcium chloride modified Portland cement possesses adequate compressive strength for orthopedic applications [47]. The calcium silicate cements with calcium chloride release more calcium ions and increase pH immediately, although no difference was observed in the other intervals [105]. The addition of calcium carbonate and calcium chloride combination to MTA (Portland cement containing bismuth oxide) also showed a shorter setting time compared to MTA Angelus and ProRoot MTA [58].
In vitro evaluation of biocompatibility and bioactivity of Portland cement containing calcium chloride has shown no cytotoxicity against stem cells from human exfoliated deciduous teeth at concentrations <12.5 mg/ml [96, 106]. In contrast, fast-set Portland cement has high cytotoxicity against hDPSCs [53]. Implantation of dentin tubes containing Portland cement with 10% calcium chloride in the subcutaneous tissue of rats resulted in biomineralization in the dentin-cement interface [99]. Portland cement containing 3 wt% calcium chloride exhibited the most bioactivity and the highest apatite forming ability when immersed in simulated body fluid (SBF) solution [107].
8. MODIFICATION OF PORTLAND CEMENT WITH GRAPHENE OXIDE
Portland cement reinforced with carbon-based nanomaterials such as carbon nanofibers, multiwalled carbon nanotubes and graphene oxide nanoplatelets has shown improved fracture toughness and water-resistant properties with decreased porosity [108, 109]. Graphene oxide accelerates Portland cement hydration process by improving crystal growth and providing a nucleation effect. Graphene oxide accelerates the formation of C3S, which is responsible for the early strength of the material, although it has been reported to affect the hydration of C3A more perceptibly than C3S [110, 111]. The incorporation of 0.1% graphene oxide increases Portland cement hydration degree up to 10.4% in 28 days [112]. The carboxyl acid groups of graphene oxide can bond covalently with C-S-H and improve the mechanical properties of cement. Furthermore, graphene oxide acts as a reinforcing filler and decreases cement's porosity and permeability, prevents propagation of cracks and presents an abrasion-resistant dense microstructure [6, 110, 113]. Graphene oxide can improve the compressive strength of Portland cement by 33% and its flexural strength by 59% [114]. Moreover, it has been reported to increase the compressive strength of Portland cement by 46% and its tensile strength by 53% [115]. The addition of 0.1 wt% nano-graphene oxide increases the compressive strength of Portland cement from 54.2 MPa to 84.5 MPa, which is about 55.8% [113].
The incorporation of 5% graphene oxide into various bioactive calcium silicate cement improves the mineralization significantly and does not affect the proliferation of hDPSCs [116]. Another study revealed that calcium silicate ceramic composites reinforced with graphene oxide nanoplatelets have acceptable apatite forming ability, biocompatibility and also promote the viability and proliferation of human osteoblast cells [117]. The addition of 1 wt% graphene oxide nanoparticles to Portland cement improves its microhardness without affecting its biocompatibility [118]. On the whole, the addition of graphene oxide to Portland cement exhibits beneficial effects on its workability and durability, however, further investigations on the biocompatibility of this material are required to be applied in clinical studies.
9. MODIFICATION OF PORTLAND CEMENT WITH CARBON NANOTUBES
Single-walled carbon nanotubes (SWCNTs) and multi-walled carbon nanotubes (MWCNTs) are promising reinforcements for tissue engineering and producing cementitious materials due to their multi-functional properties. Provided well dispersion of nanotubes in the cement matrix, CNTs act as a filler and produce a denser material and prevent crack propagation [119]. Moreover, CNTs affect the cement by bridging between hydration products and consequently enhancing composite toughness [120, 121]. Another consideration in this regard is that CNTs act as nucleation sites for hydration products resulting in a high percentage of C-S-H and portlandite [122]. Despite conflicting results in early works on CNTs-modified Portland cement, predominant studies agree on improved flexural [9], compressive [9, 123] and tensile strength [124] and modulus of elasticity [125] following the addition of CNTs to Portland cement [126].
10. MODIFICATION OF PORTLAND CEMENT WITH NANO-SILICA
Another nanoparticle to be introduced into Portland cement to enhance its performance in terms of workability and long-term durability is nano-silica. Nano-silica particles act as a filler in the paste and provide nucleation sites, thus accelerate cement's hydration process. On the other hand, it affects cement characteristics by undertaking a pozzolanic reaction, which further intensifies C-S-H gel growth and densifies the final cement [127-129]. The hydration rate of cement depends on the surface area of added silica particles and the pattern of dispersion. Although, in recent years, attention has been widely paid to the impact of nanoparticles on the hydration of C3S, few studies showed that nano-silica can improve the hydration of C3A with a decrease in the heat release rate during the process [130]. Notwithstanding, further exploration is required for a better understanding of the influence of silica on the hydration mechanism of C3S and C3A. It is well known that nano-silica containing cement presents a shorter setting time, reduced formation of calcium hydroxide, dense and impact microstructure and superior mechanical properties [131]. The addition of nano-silica to Portland cement was found to improve its compressive, flexural and split tensile strength in a concentration-dependent manner [132, 133]. Shih et al. found that the addition of 0.6 wt% nano-silica results in an increase in the compressive strength of Portland cement (water/cement ratio of 0.55) by 43.8% [134]. Furthermore, the addition of nano-silica to MTA was shown to reduce the setting time and increase the compressive and flexural strength in 7 days, although changes were not significant [135]. Despite the wide investigation of the rheological and mechanical properties of nano-silica-contained Portland cement, the biocompatibility and bioactivity of this composition require further studies.
11. MODIFICATION OF PORTLAND CEMENT WITH HYDROXYAPATITE
Calcium silicate cement reinforced with hydroxyapatite are biointeractive materials with apatite forming ability and are great candidates for bone substitute materials [136-138]. Evaluation of biocompatibility and inflammation response in vivo showed more acceptable results for hydroxyapatite-containing cement compared to uncoated ones [137]. The incorporation of hydroxyapatite into tricalcium silicate cement has also been reported to reduce its genotoxicity [139]. The addition of nano-hydroxyapatite to Portland cement affects the hydration process and results in decreased compressive strength, although after immersion in SBF solution for 4 weeks and precipitation of bone-like apatite compressive strength increases [140]. Dasgupta et al. assessed the possessions of Portland cement by adding micro and nanoparticles of hydroxyapatite and observed better physical, mechanical and biological properties for the material with particle sizes of 168 nm. Then, according to their results, the addition of nano-hydroxyapatite could be an option for improving the properties of the calcium silicate-based cement [141]. Furthermore, Tanomaru et al. reported that the addition of hydroxyapatite to Portland cement affected negatively on compressive strength and solubility of the cement, however, the antibacterial property was improved [142].
12. MODIFICATION OF PORTLAND CEMENT WITH NANO-CALCIUM CARBONATES
Nano calcium carbonate has finer particles with more surface area compared to micro calcium carbonate and thus affects more on hydration process and mechanical properties [143]. The addition of calcium carbonate nanoparticles to Portland cement accelerates the setting process and reduces the initial and final setting times and the water needed for cement hydration and also improves mechanical resistance without altering solubility or dimensional stability [143-145]. Calcium carbonate shortens the induction period of the hydration process by nucleation effect and accelerating the formation of ettringite. It has been reported that the incorporation of 1% nano-calcium carbonate into Portland cement results in the optimal performance of cement as it increases the compressive strength of Portland cement by 7% after 56 days and reduces the permeability by 13% and shrinkage by 66% [146, 147]. Further increase in calcium carbonate concentration might affect the compressive strength adversely due to the increased amount of harmful pores [148]. Overall, the modification of cement characteristics depends on the content and particle size of added calcium carbonate [147].
13. RESIN-MODIFIED PORTLAND CEMENT
Due to many drawbacks of MTA and Portland cement, more importantly, their poor bonding to resins, resin-modified Portland cement was introduced in 2011. TheraCal is a hydraulic silicate cement composed of 45% Portland cement type ΙΙΙ, 43% resin and variable amounts of fumed silica and radiopacifiers, including barium sulfate and bismuth oxide [149, 150]. TheraCal releases a significant amount of calcium ions throughout 28 days and alkalinizes its environment up to pH 11, which decreases to pH 8.5 during 14 days. Furthermore, it has significantly less solubility than ProRoot MTA and Dycal [151]. The resin matrix of TheraCal alters its setting mechanism and amount of calcium ion release, however, it is in a concentration range to promote the proliferation and differentiation of hDPCs and the formation of mineralized hard tissue [152, 153]. TheraCal has satisfactory compressive and push-out bond strength to dentin and microshear bond strength to different restorative materials, which were significantly superior to MTA and Dycal [154-156]. In vitro evaluation of biocompatibility and bioactivity of set TheraCal on hDPSCs showed that although TheraCal promoted the mineralization after 21 days, it exhibited low cell proliferation, slower cell migration and ALP activity compared to Biodentin [157-159]. The re-mineralization speed of TheraCal is less than resin-free hydraulic calcium silicate cement [160]. Moreover, the amount of necrotic cells associated with TheraCal is higher compared to other Portland cement-based pulp capping materials [161]. Clinical and animal studies investigating TheraCal are listed in Table 2. Considering all aspects, it seems that resin-free Portland cement have shown more satisfactory results concerning biocompatibility and clinical applications such as vital pulp therapy.
Refs. | Other Groups | Application | Teeth | Treatment Period | Clinical/Radio-Graphical Observations | Histological Evaluations |
---|---|---|---|---|---|---|
Human Studies | ||||||
Bakhtiar et al. [191] |
Biodentin ProRoot MTA |
Partial Pulpotomy | Third molars | 8 weeks | TheraCal had a success rate of 80% | Incomplete dentin bridge formation was detected in the TheraCal group, no pulp inflammation was detected except for one in the TheraCal group Only 11.11% of the TheraCal group had normal pulp organization |
Gurcan et al. [192] |
Dycal ProRoot MTA |
Indirect pulp capping | First Permanent molars/ Second primary molars | 2 years | TheraCal had a success rate of 87.7% (No difference between groups) | - |
Erfanparast et al. [193] |
MTA | Direct pulp capping | Primary molars | 1 year | TheraCal had a 91.8% success rate (No difference between groups) | - |
Cengiz et al. [194] |
Ca(OH)2 | Direct pulp capping | Premolar/molar | 6 months | TheraCal had a 66.6% success (No difference with Ca(OH)2) | - |
Menon et al. [195] |
MTA | Indirect pulp capping | Primary molars | 6 months | No significant difference between groups in dentin forming | - |
Alqahtani et al. [196] |
Ca(OH)2 | Direct/Indirect pulp capping | - | 3 months | TheraCal had a success rate of 85.5% (No difference between groups) | - |
Peskersoy et al. [197] |
Biodentin Dycal MTA |
Direct pulp capping | - | 36 months | TheraCal had 72.1% and 73.6% clinical and radiographical success rate (No difference with dycal) | - |
Sahin et al. [198] |
Dycal Biodentin |
Indirect pulp capping | Primary molars | 24 months | TheraCal had a 93.3% success rate (No difference between groups) | TheraCal formed an incomplete odontoblastic layer and showed pulpitis |
Animal studies | ||||||
Cannon et al. [199] |
Pure PC Resin-based Ca(OH)2 GI |
Pulp capping | - | 4 weeks | Complete hard tissue formation in PC and TheraCal group | TharaCal and PC formed the thickest dentin bridge |
Lee et al. [200] |
ProRoot MTA RetroMTA |
Partial Pulpotomy | - | 4 weeks | - | TheraCal group showed lower dentin bridge formation, extensive inflammation, less osteocalcin and dentin sialoprotein expression |
Li et al. [201] |
ProRoot | Direct pulp capping | - | 70 days | No significant tooth discoloration | Mineralized tissue was detected beneath the exposed pulp with no inflammatory response |
Hinata et al. [202] |
MTA Prototype CSC |
Subcutaneous implantation | - | 28 days | - | Ca and P containing apatite-like surface precipitated with the least thickness compared to other groups |
14. OTHER MODIFICATIONS
Quaternary ammonium compounds are widely used in medicine due to their high antimicrobial efficacy as well as low cytotoxicity and biological effects. The incorporation of quaternary ammonium into dental adhesive systems and endodontic cement has beneficial effects on antimicrobial and mechanical properties and biocompatibility [162]. Light cured resin-modified Portland cement containing quaternary ammonium salt has been introduced as a pulp capping material with acceptable antibacterial activity [163]. This material showed better alkalinizing activity and calcium release ability compared to Dycal during 28 days after curing, although pH value and calcium release amount decreased over time. The modified Portland cement had acceptable cytotoxicity against hDPFs and apatite forming ability in phosphate buffered saline [164]. Furthermore, it enhanced the adhesion and migration of hDPSCs and the expression of osteogenesis-related genes. HDPSCs showed higher alkaline phosphatase activity and calcium deposition when cultured with quaternary ammonium-modified Portland cement [165].
Superplasticizers are fundamental additives in concrete production to control cement performance since they strongly influence concrete rheology properties. Among various generations of superplasticizers, polycarboxylate with spectacular properties has been evaluated for dental applications [166]. The unique structure of polycarboxylate allows applying modifications to its functional group, main chain or side chains in order to achieve the expected performance from Portland cement [167]. Polycarboxylate-modified Portland cement has a short setting time in the range of 4-11 minutes and presents more flowability in a very low water:cement ratio [168]. On the whole, the addition of superplasticizers improves the workability of Portland cement and leads to a rapid increase in the formation of high-strength cement with low permeability [169].
Another modification to the chemical structure of Portland cement is the addition of chitosan, which has been reported to improve the handling properties of cement [170]. It should be noticed that the influence of chitosan derivatives on the behavior of Portland cement strongly depends on the main substitution group of the derivative [171]. Chitosan crystallites spread through the material structure, fill the pores and provide a homogenous morphology, which in turn improves the biological characteristics of the cement by promoting cell adhesion and spreading. Chitosan-modified Portland cement exhibited good mechanical properties with improved solubility and extended setting time. However, compressive strength showed a decrease dependent on the chitosan content [172]. Furthermore, chitosan containing Portland cement exhibited less heavy metal leaching [173]. Carboxymethyl chitosan also acts as an anti-washout additive and extends the setting time of tricalcium silicate cement. This novel bone cement presents good bioactivity with the deposition of crystalline apatite [174]. Chitosan and dicalcium phosphate showed a synergistic effect on the apatite forming ability of Portland cement [170]. Kamali et al. added chitosan and zirconium oxide to Portland cement as a substitute for MTA. In this study, malic acid-containing chitosan composed the liquid phase. Malic acid reacted with free amino groups of chitosan and consequently provided expanded polymer chains, which allowed the enhanced formation of hydration by-products within the polymer chains [175].
Geopolymer is a kind of amorphous aluminosilicate with satisfying physical and mechanical properties and durability due to the polymerization reaction [176]. The apatite formation ability of geopolymer when immersed in SBF solution shows the potential of this material to be used in bioenviroments [177, 178]. Modification of white Portland cement with aluminosilicate materials such as calcined kaolin geopolymer system produces a favorable bone repair material with rapid setting and high compressive strength. Furthermore, this system exhibited good bioactivity when exposed to SBF solution and promoted hydroxyapatite precipitation [179, 180]. Fig. (1) shows various applications of Portland cement in dentistry.
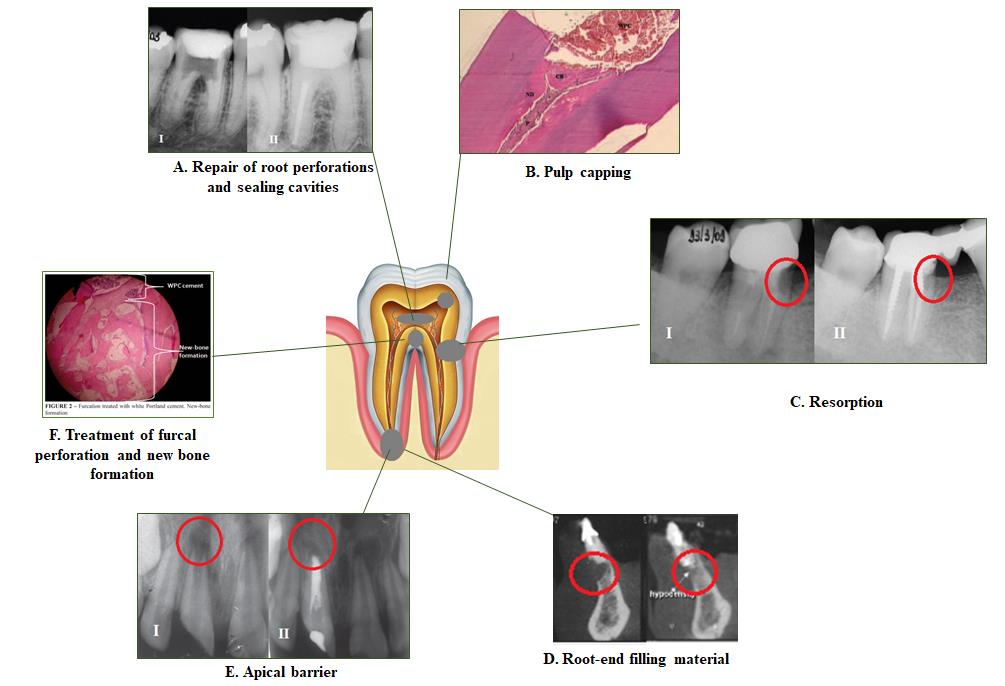
CONCLUSION
As a biocompatible bioactive endodontic cement with favorable characteristics and a wide range of applications, Portland cement and its derivatives have been under multiple investigations, although extensive issues are still open for further research. As reported in this review, a significant attempt has been made to extend the application scope of Portland cement with various modifications, such as the inclusion of polymers, radiopacifiers, metal oxides, superplasticizers and nanomaterials, namely nano-silica, titanium oxide and calcium carbonate. Consequently, various MTA types and resin-modified Portland cement have been introduced. In the dentistry field, Portland cement is widely serviceable in restorative dentistry and particularly in different kinds of endodontic treatments. We can conclude that Portland cement has the potential to be used as an acceptable pulp capping material with the least complaints in the long term. The addition of radiopacifiers can contribute to improve the utilization efficacy of Portland cement. Given the valuable properties of Portland cement and its low price compared to MTA, considering it as an alternative to MTA seems to be beneficial. Nonetheless, the key issue is bringing the various modified Portland cement into clinical practice and evaluating them in the bioenvironment. Besides, it has been reported that some toxic heavy metals such as chromium and lead are released from Portland cement. This can be of concern when Portland cement is in contact with hard and soft tissues and should be considered a subject for future studies.
LIST OF ABBREVIATIONS
MTA | = Mineral Trioxide Aggregate |
HBSS | = Hank's Balanced Salt Solution |
CONSENT FOR PUBLICATION
Not applicable.
FUNDING
The Vice Chancellor for Research at Tabriz University of Medical Sciences provided financial support.
CONFLICT OF INTEREST
Declared none.
ACKNOWLEDGEMENTS
This article was written based on a dataset from a thesis registered at Tabriz University of Medical Sciences . The thesis was supported by the Vice Chancellor for Research at Tabriz University of Medical Sciences that is greatly acknowledged.