In Vitro Fatigue and Fracture Load of Monolithic Ceramic Crowns Supported by Hybrid Abutment
Abstract
Objective:
This study evaluated the performance of zirconia and lithium disilicate crowns supported by implants or cemented to epoxy resin dies.
Methods:
Eigthy zirconia and lithium disilicate crowns each were prepared and assigned in four groups according to the crown material and supporting structure combinations (implant-supported zirconia, die-supported zirconia, implant-supported lithium disilicate, and die-supported lithium disilicate). Ten crowns in each group acted as control while the rest (n=10) underwent thermocycling and fatigue with 100 N loading force for 1.5 million cycles. Specimens were then loaded to fracture in a universal testing machine. Data were analysed using one-way ANOVA and Tukey multiple comparison test with a 95% level of significance.
Results:
No implants or crown failure occurred during fatigue. The mean fracture load values (control, fatigued) in newton were as follows: (4054, 3344) for implant-supported zirconia, (3783, 3477) for die-supported zirconia, (2506, 2207) for implant-supported lithium disilicate, and (2159, 1806) for die-supported lithium disilicate. Comparing the control with the corresponding fatigued subgroup showed a significantly higher fracture load mean of the control group in all cases. Zirconia showed a significantly higher fracture load mean than lithium disilicate (P=0.001, P<0.001). However, comparing crowns made from the same material according to the supporting structure showed no significant difference (P=0.923, P=0.337).
Conclusion:
Zirconia and lithium disilicate posterior crowns have adequate fatigue and fracture resistance required for posterior crowns. However, when heavy fatigue forces are expected, zirconia material is preferable over lithium disilicate. Zirconia and lithium disilicate implant-supported crowns cemented to hybrid abutments should have satisfactory clinical performance.
1. INTRODUCTION
Recent developments in computer-aided design/computer-aided manufacturing (CAD/CAD) have led to the introduction of all-ceramic restorative systems with increased strength, reliability, and aesthetic capabilities [1-3]. The earliest introduced alternatives, such as In-Ceram or Dicor, did not achieve the expected success because of inadequate mechanical properties, unacceptable fitting, or impractical manufacturing processes [4-7]. However, zirconia (Zr) and lithium disilicate (LD) ceramics are materials with desirable properties [8-12].
Currently, both LD and Zr ceramics are used to fabricate monolithic posterior restorations. A study [13] conducted in 2015 reported that monolithic Zr was the most prescribed material for posterior single crowns (32%) while LD was the top choice for anterior single crowns (54%). These preferences are obviously linked to the mechanical properties of Zr and the aesthetic properties of LD. The same survey [13] found that more aesthetically attractive choices such as PFM (31%) and LD (21%) were prescribed for posterior single crowns in high percentages. Lately, the concept of the aesthetic zone has changed, and the demand for highly aesthetic restorations even in the posterior region is evident in the rise of the use of composites in restoring posterior teeth [14] and the development of advanced all-ceramic materials that can withstand heavy occlusal loads in the posterior region. Therefore, the use of more aesthetically pleasing ceramics in the posterior region such as LD and more recently introduced zirconia reinforced lithium silicate (ZLS) restorations is expected.
Considering the present trend toward more conservative dental rehabilitation [15, 16], treatment of a missing tooth with an implant-supported single crown rather than a fixed partial denture should be a more rational treatment option [17, 18]. Implant-supported single crowns showed high survival rates [19, 20]. In addition, the results of a recent systematic review [20] found that the survival of implant-supported single crowns (93% after 5 years and 94.4% after 10 years) were higher than that of tooth‐supported all‐ceramic FPDs (86%–90%) [21], which is considered the traditional treatment option for a single tooth replacement. Although the decision of replacing a missing tooth with an implant crown can be the most reliable and conservative treatment modality, many factors can influence this decision, and in many cases, the relatively high cost of implant treatment is considered a major determinant regardless of oral health status and patient preference [22].
The application of hybrid abutment design using zirconia abutments is expected to provide both strength and aesthetics combined. However, few previous laboratory studies investigated the fracture resistance of ceramic crowns supported by hybrid abutment [23-26]. This study aimed at investigating fatigue survival and fracture load of monolithic Zr and LD crowns when supported by implants or cemented to epoxy resin dies. The study also investigated the in vitro fatigue performance of the hybrid abutment system composed of the all-ceramic crown, all-ceramic abutment and, Ti-Base. This study proposed two hypotheses:
- Survival and fracture resistance of Zr and LD are not significantly affected by chewing simulation (1.5 million cycles) in thermocycled water.
- Fracture resistance of implant-supported crowns is not significantly different from that of crowns cemented to epoxy resin dies within the same ceramic material groups.
2. MATERIALS AND METHODS
A total of 80 crowns were prepared for this study and assigned to four major groups according to crown material and supporting structure combinations
- Zr crowns supported by implants (n=20)
- Zr crowns cemented to epoxy resin dies (n=20)
- LD crowns supported by implants (n=20)
- LD crowns cemented to epoxy resin dies (n=20)
2.1. Implants and Tooth Preparation
For the implant-supported crowns, 40 implants (Ankylos; Dentsply, Mannheim, Germany), Titanium Base (Ti-Base) abutments (Dess; Dental Smart Solutions, Montcada, Spain) with hex screw were used. Half the implants were used with monolithic Zr, and the other half were used with monolithic LD crowns, with all being fabricated using CAD/CAM technology.
For the crowns cemented to epoxy resin dies, a typodont model (A25A; Nissin Dental Products, Kyoto, Japan) of the mandibular first molar was prepared by an experienced prosthodontist according to the preparation guidelines for all-ceramic crowns to achieve 1.5 mm occlusal reduction, 1.0 mm proximal and axial reduction and a 1 mm shoulder with rounded inner edges. Vinyl polysiloxane impression (Imprint; 3M ESPE, MN, USA) of the prepared tooth model was repeated 40 times and poured with epoxy resin material (Exakto-Form; Bredent, Senden, Germany), resulting in 40 replicas of the prepared master die.
At this stage, the implants and epoxy resin dies were prepared and embedded in an acrylic resin base to fit the specimens’ holders of the chewing simulator and the universal testing machine planned to be used for the fracture test. Therefore, the specimen’s holder of the chewing simulator was used to create acrylic resin bases of the implants, and the epoxy resin dies. These bases guaranteed a standardized location of all crowns in the chewing simulator during fatigue testing (Fig. 1).
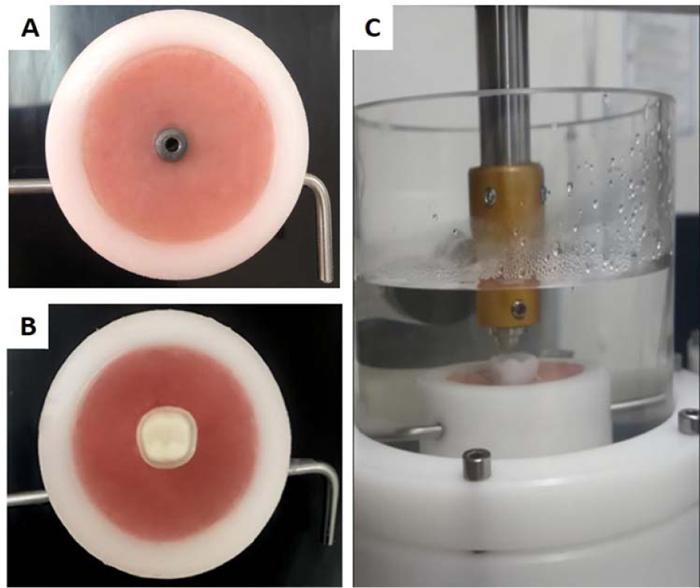
2.2. Zr Abutments and Crowns Preparation
The Ankylos ScanBase was scanned (D1000; 3shape, Copenhagen, Denmark) to obtain the geometry of the Ti-Base required to design Zr abutments. Then, the monolithic Zr and LD crowns were designed with split-file technology. The crown was designed according to the manufacturer's recommendations for full-contour crowns with a circular thickness of 1.0 mm and an occlusal thickness of 1.5 mm. A 5-axis milling machine (Zenotec select; Wieland Dental, Lindenstraße, Germany) was used to produce Zr abutments (n=40) and Zr crowns (n=20) from pre-sintered Zr discs (Zenostar Zr; Wieland Dental, Lindenstraße, Germany). The LD crowns (IPS e.max CAD; Ivoclar Vivadent, Schaan, Liechtenstein)) were wet milled in a wet milling machine (Zenotec select hybrid; Wieland Dental, Lindenstraße, Germany). After the CAM process, Zr components were sintered, and LD crowns were crystallized in the recommended furnaces (Programat S1 and Programat EP 3010; Ivoclar Vivadent, Schaan, Liechtenstein) respectively. Afterward, the fitting accuracy of the abutments and monolithic crowns was confirmed, and all crowns were glazed as recommended by the manufacturer.
To construct the crowns supported by epoxy resin dies, the preparation was firstly scanned with a laboratory scanner (D1000; 3Shape, Copenhagen, Denmark). Crown was designed for full-contour crowns with 1.0 mm circular and 1.5 mm occlusal thickness corresponding to the tooth preparation applied on the typodont model. The CAD file was then sent to the dry milling machine (Zenostar Zr; Wieland Dental, Lindenstraße, Germany) to mill 20 Zr crowns and a wet milling machine (Zenotec select hybrid; Wieland Dental, Lindenstraße, Germany) to produce the LD crowns. Sintering, crystallization, and glazing were carried out according to the manufacturer's instructions in the recommended furnaces (Programat S1 and Programat EP 3010; Ivoclar Vivadent, Schaan, Liechtenstein) respectively.
2.3. Cementation
Ti-Base abutments were firstly screw tightened on the implants with a torque wrench driver (Hex Driver; Dentsply, Mannheim, Germany) to 20 N/cm, and before cementation of the different components of the implant system, a temporary restorative material was used to fill the Ti-Base access hole. Then, Zr abutment and Ti-Base were cemented using self-curing composite (Multilink Hybrid Abutment; Ivoclar Vivadent, Schaan, Liechtenstein), and crowns were adhesively luted to the zirconia abutments (Multilink Automix; Ivoclar Vivadent, Schaan, Liechtenstein). Similarly, crowns supported by epoxy resin replicas were luted to the corresponding dies using the same cementation material (Multilink Automix; Ivoclar Vivadent, Schaan, Liechtenstein). All specimens were stored in water at a constant temperature (37 °C) for 30 days until the commencement of testing.
2.4. Fatigue and Fracture Testing
In each group (n=20), 10 specimens represented the control group while the other 10 underwent fatigue by means of a chewing simulator (CS-4.8; SD Mechatronik, Feldkirchen-Westerham, Germany) for 1.5 million cycles. Fatigue testing protocol involved a loading force of 100 N applied by a 6 mm diameter spherical stainless steel indenter and 1.2 Hz loading frequency. The indenter start point was confirmed at 0.5 mm lingual to the distobuccal cusp tip using articulating paper. A single loading cycle consisted of contact between the indenter and the specimen, load application, a lingual slide for 0.3 mm while the load is applied [27, 28]. Then the indenter leaves with an opening distance of 2 mm. Thermal cycling in controlled thermocycling unit (CS-4.8; SD Mechatronik, Feldkirchen-Westerham, Germany) between 5 °C and 55 °C (dwell time: 60 s, pause time: 15 s) in distilled water was carried out throughout the testing.
Specimens were checked for failure at 20 × magnification (M125; Leica Microsystems, Wetzlar, Germany). Fracture test for all crowns; fatigued and control was then carried out in a universal testing machine (Model LRX; Lloyds Instrument, West Sussex, UK) with a load cell of 5 kN loading capacity and less than 0.005% load resolution. Specimens were adjusted at a standardized location in the universal testing machine to achieve three points of contact with the indenter. The contacts were identified at the triangular ridges of the mesiolingual, distolingual, and distobuccal cusps. Vertical load with an 8 mm diameter stainless steel indenter at 1 mm/min crosshead speed was applied on the crowns until failure. Forces required to fracture the crowns were recorded in Newton.
Statistical analysis of the fracture load data was carried out using a statistical software package (SPSS, Version 23.0; IBM Analytics, Armonk, NY, USA). Normal distribution of data was confirmed using the Shapiro-Wilk test. The data was described descriptively and then analysed using a one-way analysis of variance (ANOVA) and Tukey multiple comparison test. The level of significance applied for all statistical analyses was set at 95%.
The sample size was initially chosen according to previous similar studies [28-40], which indicated that the number of specimens in subgroups ranged from 7 to 15, with a sample size of ten specimens being the most commonly used [28, 33-37]. We also conducted a post hoc power analysis using GPower [41] to ensure that our study design had enough power to detect the effect of chewing simulation and the effect of different material and supporting structure combinations on the fracture load of the crowns. Power calculation determined that a sample size of eight specimens would provide a 95% chance of finding a significant difference between the corresponding groups at the 5% level. The effect size found (1.47) indicates a large difference effect [42]. Therefore, ten crowns in each subgroup should be adequate.
3. RESULTS
No implants or crown failure occurred during fatigue testing in the chewing simulator. However, all crowns showed wear facets at the occlusal indenter contact point, which were more evident in the LD crowns.
The mean fracture load and standard deviations of all groups are presented in Table 1. Fracture load means of LD groups were statistically significantly lower than that of the corresponding Zr groups with P = 0.001 between Zr and LD groups supported by implant and P < 0.001 between Zr and LD groups cemented to epoxy resin dies. Comparing crowns made from the same crown material according to their supporting (implant or epoxy resin) showed no significant difference with P = 0.923 between the two Zr groups and P = 0.337 between the two LD groups. In all groups, chewing simulation caused a statistically significant reduction in the mean fracture load ranging from 7.6 to 16.3%.
Groups ( n=20) | Sub group ( n=10) |
Mean (St Dev) | Reduction in Fracture Load after Fatigue |
Implant-supported Zr | Control | 4054 (367)A | 7.6% |
Fatigued | 3344 (547)a | ||
Die-supported Zr | Control | 3783 (522)A | 8% |
Fatigued | 3477 (426)a | ||
Implant-supported LD | Control | 2506 (426)B | 12% |
Fatigued | 2207 (395)b | ||
Die-supported LD | Control | 2159 (231)B | 16.3% |
Fatigued | 1806 (237)b | ||
Different capital superscripts indicate heterogeneous subsets (P < 0.05). Different small superscripts indicate heterogeneous subsets (P < 0.05). Between control and fatigued subgroups: different case superscripts indicate heterogeneous subsets (P > 0.05) |
Fracture test of the crowns (Zr and LD) resulted in cohesive bulk fractures involving all crown thickness, regardless of being supported by an implant or cemented to epoxy resin dies. Fractures observed can be generally categorized into three patterns; a straight fracture running mesiodistally following the central groove (2 fragments), fracture running mesiodistally, and along the lingual groove (3 fragments), or more catastrophic shuttering of the crowns into several pieces (Fig. 2). There was no specific fracture path for any of the materials; however, as clearly seen in Table 2, the distribution of different fracture patterns in the two materials indicates more catastrophic patterns in the LD crowns.
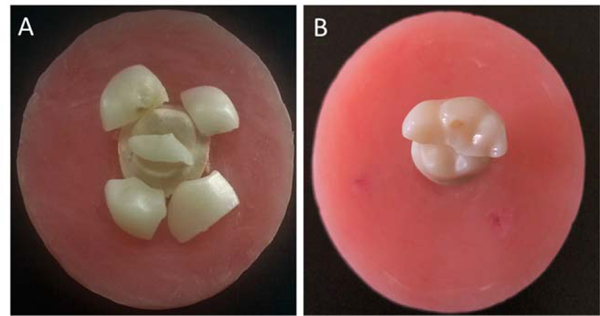
No fracture in the epoxy resin dies was observed during fatigue. Similarly, none of the zirconia abutments neither any of the Ti-Bases or implants were fractured during fatigue or after the fracture test, and no screw loosening was observed.
4. DISCUSSION
Despite the 100% survival of the crowns fatigued in this study, the results showed a statistically significant reduction in mean fracture load values compared to the corresponding control groups (Table 2), which rejects the first null hypothesis. These findings are in agreement with previous studies that tested LD [43-45] and Zr restorations [43, 46]. Reduction in fracture resistance can be explained by the accumulated damage of ceramic materials occurring during fatigue testing, which ultimately weakens the restorations [47]. In addition, specifically for zirconia material, accelerated aging can significantly weaken the restorations [48, 49]. Another study [50], however, reported no significant influence of thermal cycling and mechanical loading (TCML) on the fracture resistance of zirconia crowns. Though, it should be noted that the study [50] used low loading force of 50 N.
Material | - | 2 Fragments (Percentage) | 3 Fragments (Percentage) | 4 or more Fragments (Percentage) |
Zirconia crowns (implant supported and die supported) | Fatigued (n=20) | 16 (80%) | 1 (5 %) | 3 (15%) |
Unfatigued control (n=20) | 18 (90%) | 2 (10%) | 0 | |
Lithium disilicate crowns (implant supported and die supported) | Fatigued (n=20) | 0 | 3 (15%) | 17 (85%) |
Unfatigued control (n=20) | 6 (30%) | 5 (25%) | 9 (45%) |
Comparing the two materials (Zr and LD) as presented in Table 1 shows that the reduction in fracture resistance was more pronounced in the LD groups. Previous studies [30, 51-54] reported a significantly higher fracture load of Zr crowns than that of LD. Additionally, reviewing the fracture patterns after single load to fracture (Table 2) shows that the majority of the zirconia crowns fractured into two pieces (buccal and lingual halves), while LD crowns, especially the fatigued ones, mostly followed more catastrophic fracture pattern into several pieces. Fractures that occurred in LD crowns may indicate the formation of internal cracks during fatigue and reflect the greater effect of chewing simulation on the material. This should be considered clinically as both materials are indicated for posterior crowns. Zirconia appears to be the reasonable choice when considerable fatigue and higher occlusal load are expected.
Nevertheless, none of the LD crowns failed during fatigue testing. The mean fracture load, although significantly lower than that of Zr was well above the fracture load required for posterior restorations [55]. This agrees with previous studies as LD posterior crowns demonstrated good clinical performance with a low prevalence of mechanical failure along different follow-up periods [56-63]. The material also showed excellent in vitro fatigue survival [64-68]. The performance of LD crowns in this study might be linked to its low modulus of elasticity (63.9 GPa) compared to that of Zr abutment. This, according to previous studies [69, 70], allows better load dissipation and enhances fracture resistance of the structure. Therefore, when the expected occlusal loads are within the normal physiologic range, the use of LD material for posterior crowns might be more satisfactory for patients because it combines strength and preferable aesthetics.
The results show that the mean fracture load value of implant-supported crowns was insignificantly higher than that of the crowns supported by epoxy resin dies, confirming the second null hypothesis. Similar results were also reported in previous studies [71, 72], as tooth or implant situations can only partially influence the fracture resistance of ceramic crowns [73]. This indicates that an implant-supported posterior crown can be just as successful as a tooth-supported crown treatment. On the contrary, a recent study [53] found significantly higher fracture load values for implant-supported LD and Zr crowns compared to tooth-supported ones. The study [53] used natural teeth, which might have influenced the results because they cannot be standardized due to inevitable individual differences in shape, quality, and geometry of the human teeth. Epoxy resin was used in this study instead of natural teeth, which might be considered a limitation. However, it was chosen to overcome the inability to standardize natural teeth specimens [74]. The elastic modulus of this material (3 GPa) is close to that of dentine (11-20 GPa) [75], and therefore, it has been previously used as a comparable alternative to simulate natural teeth and to produce standardized specimens [35, 40, 76, 77].
This study used the hybrid abutment design, which was introduced to meet the increased aesthetic demand of the patients. No failure occurred in any of the implants, Ti-Bases, or Zr abutments during fatigue. In addition, failure of the specimens after the fracture test occurred only in the crowns, which proposes a good performance of this implant abutment design. These results disagree with a recent study [25] that found that Zr and LD hybrid implant-supported crowns failed in a significant number (18.8% for Zr and 43.8% for LD) during fatigue testing despite their high fracture strength. Failures in zirconia crowns occurred in the Ti-Bases, which were short (3mm), whereas failures in LD crowns occurred in both the Ti-Bases and ceramic superstructures [25]. Thus, the authors recommended that their use as posterior restorations should be considered critically [25]. In fact, the available data on the performance of this implant design is still insufficient and more laboratory, and clinical studies are required [43, 78].
This study used a testing protocol to simulate the challenging conditions in the oral cavity, including cyclic mechanical loading, wet environment, and fluctuating temperatures [79]. Therefore, fatigue forces were applied with a simultaneous thermocycling in water between 5 °C and 55 °C all through the testing period [80] to produce the effect of these challenging conditions. In addition, a 0.3 mm lateral movement while the load is applied should mimic the lateral movement of the jaw during function and its evident destructive effect on the restorations strength [81, 82]. Nevertheless, the simulation of the periodontal ligaments in the groups cemented to epoxy resin dies was not considered in this study, which might produce some effects on the results. Further, examining the fatigued crowns under 20 × magnification is considered a limitation as Scanning Electronic Microscopy (SEM) could offer a great advantage by detecting micro-cracks and defects. It should also be noted, however, that the results of in vitro investigations have to be interpreted cautiously and cannot be transformed directly to clinical conditions; clinical studies are always crucial to judge materials and designs performance.
CONCLUSION
Within the limitations of this in vitro study, it can be concluded that both Zr and LD posterior crowns have adequate fatigue and fracture resistance required for posterior crowns. However, when heavy fatigue forces are expected, Zr material is preferable over LD. Zirconia and LD implant-supported crowns cemented to hybrid abutments should have satisfactory clinical performance under physiologic masticatory forces. The hybrid-abutment design appears as an adequate approach in implant-supported restorations.
ETHICS APPROVAL AND CONSENT TO PARTICIPATE
Not applicable.
HUMAN AND ANIMAL RIGHTS
Not applicable.
CONSENT FOR PUBLICATION
Not applicable.
AVAILABILITY OF DATA AND MATERIALS
The data supporting the findings of the article is available from corresponding author [N. N] upon reasonable request.
FUNDING
This research was funded by the Deanship of Research, Jordan University of Science and Technology, Jordan and School of Medicine and Dentistry, Griffith University, AUS.
CONFLICT OF INTEREST
The authors declare no conflict of interest, financial or otherwise.
ACKNOWLEDGEMENTS
Declared none.