Comparison of the Mechanical Properties of Temporary Abutments Made of Polyetheretherketone and Photopolymeric Resin
Abstract
Background:
Provisional abutments are widely used in the rehabilitation of dental implants as it allows the use of a provisional crown in order to restore patient aesthetics while the final restoration is being carried out; most of the temporary abutments available on the market are made of titanium alloygrade V (type Ti-6Al-4Va) and polyetheretherketone (PEEK), a material that exhibits very low adhesion to polymethylmethacrylate (PMMA).
Objective:
This research is aimed to compare the mechanical properties of commercially available PEEK abutments and abutments made using an additive technique with photopolymeric resin.
Methods:
Eighteen commercial temporary abutments manufactured in PEEK and eighteen experimental abutments manufactured by 3D printing using photopolymeric resin were used. The two groups of abutments were subjected to compression, bending and adhesion tests using six abutments of each type by test. Statistical analysis was performed with STATA 14 software. The data were analyzed by means of the Wilcoxon Mann-Whitney test, as these were two independent samples of reduced size. Values lower than (p <0.05) were considered statistically significant in all tests and rejected the null hypothesis of equality between the group medians.
Conclusion:
The results indicate that it is possible to make abutments with good mechanical properties in photopolymeric resin (CLEAR FLGP04) using additive techniques to be used as temporary abutments.
INTRODUCTION
Rehabilitation with implant-supported crowns is considered the gold standard for patients with tooth loss due to its high long-term survival rate and the lack of preparation requirements of adjacent teeth as it occurs in conventional techniques [1]; the most frequent cases that appear in the daily work of the dentist are the loss of single teeth for which the treatment with implants shows survival of 97.5% at 5 years [2–4].
Implant-supported restorations, in addition of being functional, demand high aesthetics that compromise the surrounding soft and hard tissues [5]. This is achieved by several factors, including temporary crowns, which allow better healing and give a definitive shape to the tissue for the emergence profile of the crown [6].
This is how the successful preparation of a provisional depends largely on the different materials on the market and their properties, which will determine its survival rate in the mouth [7].
Provisionalization in the oral rehabilitation of a patient is considered a determining phase for oral restoration, as it allows guiding and preserving the aesthetics of the soft tissues, preserving the desired tissular architecture [6], by handling concepts such as critical and subcritical contour [8,9]. Provisionalization is also essential in the healing, function and phonetic processes of the patient during treatment and shows what the definitive rehabilitation will be like [6,10].
Currently, temporary abutments made of materials such aspolyetheretherketone (PEEK) and titanium alloygrade V (type Ti-6Al-4Va) are used in the field of provisionalization on implants, they meet the standards of biocompatibility and resistance [10].
With the development of additive techniques, materials such as photopolymeric resin have recently entered the medical and dental fields, for production of protective splints, surgical guides, retainers and orthodontic aligners, among others [11].
PEEK is a synthetic thermoplastic polymer, which belongs to the polyaryletherketone (PAEK) family, due to its biocompatibility, the elastic modulus of 3-4 GPa [12] similar to the human cortical bone [13] and its melting temperature around 343 ° C [12], it has been considered an alternative in the medical and dental industry.
PEEK has low translucency and a greyish color, which creates the need to cover it with acrylic resins to improve aesthetics [13]. However, due to its low surface energy and resistance to surface modification, it is difficult to achieve a firm bond to materials such as resin, so additional etching with sulfuric acid solutions is required, which significantly increases strength of initial union as reported [13–15].
Historically, different materials have been developed for the manufacture of abutments for provisionalization on implants; initially, definitive abutments made of titanium were used, later in 1988, the University of California Los Angeles released a castable plastic abutment (UCLA) that allowed the component to be customized according to the needs of rehabilitation to be cast and screwed directly onto the implant [16]. The use of these metal abutments led to the observation that, over time, the associated peri-implant tissues had a blue-gray pigmentation that compromised the aesthetics of the restoration [17,18]. In 1993, an attempt was made to provide a solution to the problem of pigmentation associated with metal abutments with the introduction of alumina ceramic abutments and in 2004 zirconium was introduced as a material for the manufacture of abutments which were made with CAD - CAM technology [19].
Currently, most of the abutments for provisionalization are made of PEEK which is a high performance thermoplastic polymer, as having an elastic modulus similar to the bone one important advantage [20].
The most commonly used material for temporary crowns is polymethylmethacrylate (PMMA), which is a hard acrylic and thermoplastic material, which comes from acrylic acid, has high resistance to abrasion, good resistance to aging and weathering. It is transparent, with excellent optical properties with a high refractive index, low toxicity and biocompatibility [21,22].
With the evolution of additive techniques, other materials such as polymeric resins emerged, among them CLEAR FLGP04 which is composed of methacrylate oligomer (75-95%), methacrylate monomer (25-50%) and diphenyl oxide (2,4,6-trimethylbenzoyl) phosphine (less than 1%), with an indication for use in 3D printers [23].
CLEAR FLGP04 resin has a tensile strength of 38 MPa, a tensile modulus of elasticity of 1.6 GPa and a fracture strain of 12%. On the other hand, the modulus of elasticity to bending is 1.25 GPa and the deflection temperature at 264psi and 66psi it is 42.7 °C and 49.7 °C, respectively. These properties improve considerably after photocuring of the resin with 1.25 mW/cm2, 405 nm LED light, 60 min at 60 °C; as shown in Table 1 [24].
Mechanical properties | Value |
---|---|
Tensile strength (MPa) | 65 |
Tensile modulus of elasticity (MPa) | 2800 |
Flexural modulus of elasticity (MPa) | 2200 |
Thermal deflection temperature (° C) | 58.4 |
The adoption of digital technology in dentistry has been fast, computer-aided design and manufacturing are used with success in both fixed and removable prostheses, and three-dimensional (3D) printing has been an area of great growth in digital technology [25].
The term 3D printing is generally used to describe a manufacturing approach that builds objects additively, in which materials are added layer by layer to form a final multilayer object. This process is more correctly described as additive manufacturing and is also known as rapid prototyping [26].
The 3D prototyping process can be classified into three main steps: creation of a 3D model, generation of G-code in a slicing program for 3D printers, where the printing parameters are specified, and finally, the transfer of the G-code to be manufactured by a 3D printer [27]. These 3D printing technologies are not new; many of the modalities in use today were first developed and used in the late 1980s and 1990s [26–29]. However, the term “3D printing” is relatively new and is very appealing to the general public.
3D printing is one of the most advanced technologies in the manufacturing industry because it has advantages such as reduction of production time, reduction of required costs and allows the printing of articles with complex structures [29]. The most frequent uses of 3D printing in dentistry are the production of surgical guides for dental implants, the production of physical models for dental, orthodontics and surgical prostheses and implants, and the manufacture of impression copings and structures for dental prostheses [26,28,30–32]. Advances in this technology will have a great impact on the dentistry due to the affordable costs of desktop 3D printers, coupled with user-friendly software, offer opportunities for the use of polymer-based 3D printed materials in all aspects of dentistry [26].
A 3D printing method is stereolithography which uses light (laser or projector) to create thermoset polymers by activating free radicals in a liquid resin matrix, which contains chains of monomers that will be linked for it. This layered production method is characterized by being immersed in a resin bath as it is being built. Between each coat, a cleaning mechanism levels the resin to maintain consistent coat thicknesses [33].
This research was aimed to compare the biomechanical properties of commercial temporary abutments made of PEEK and abutments made of CLEAR FLGP04 light-curing resin obtained by 3D printing.
2. MATERIALS AND METHODS
The OnShape® program (Cambridge, USA) was used to model the temporary abutments; The devices were printed with CLEAR FLGP04 light-curing resin (Formlabs, Massachusetts, USA), using a Formlabs 2+ 3D printer (Formlabs, Massachusetts, USA) with the stereolithography technique, in the laboratory of the Universidad Autónoma de Occidentein Cali Colombia.
The printing parameters were: layer height: 0.1mm, filling density: 100%, printing temperature 190 °C and printing table temperature 70°C.
2.1. Mechanical Characterizations
These tests were carried out in the Materials Engineering laboratory of the Pontificia Universidad Javeriana (Sede Cali) using an Instron 3366 Universal Testing Machine (Instrom, Norwood, Massachusetts, USA) equipped with a 10kN load cell. Compression, bending and adhesion tests were performed on 18 abutments obtained by 3D printing and on 18 commercial provisional abutments made of PEEK (MIS, Galilea, Israel) using the same parameters and the same equipment.
2.2. Compression Test
In order to evaluate the mechanical compression properties of the abutments, they were adjusted with a titanium screw to a standard MIS® implant analog (MIS, Galilea, Israel.) With a torque of 15 Nm.; the analog and abutment were clamped with the lower grip of the Instron machine. Maintaining the axiality, the compressive load was applied through a disc until failure occurred. This process was repeated 6 times with the experimental abutments (photopolymerizable resin) and 6 times with the abutments of the control group (PEEK).
To develop this test, an adaptation of the ASTM D695 (ISO 604) standard was carried out. Due to the length-diameter ratio of the abutments, the probability of buckling in the compression test is high. However, it was sought to minimize buckling, conducting pilot tests where the speed was varied until the maximum test speed that did not produce significant or obvious buckling of the sample was identified (0.5 mm / min); this was done by visual verification. It is important to note that the abutment is an element that will go inside an implant, so in the application, it will have lateral supports that will prevent buckling. Also, the stresses generated in the abutment as a function of the cross-sectional area that supports the applied load was calculated (Equations 1 and 2) [34]. Fig. (1) shows a schematic representation of the abutment evaluated.
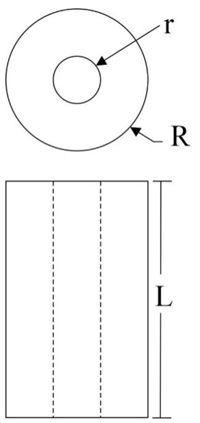


Where R is the outermost radius in the specimen and r is the innermost radius in the specimen.
2.3. Bending Test
Using a heavy body silicone impression material (Spedex, Coltene, Altstätten, Switzerland) of an upper premolar tooth, provisionals were made for 12 abutments (6 experimental abutments and 6 control abutments) in PMMA Veracryl (New stetic, Antioquia, Colombia) in a similar way to those that are manufactured in dental procedures. It was allowed to polymerize in situ for two weeks at room temperature.
To evaluate the bending mechanical properties, the sample was clamped at one end and a cantilever-type force was applied to the other until the abutment failed; the test was run at a speed of 1 mm/min. During the test, an adaptation of the ASTM D790 (ISO 178) standard was carried out; the standard considers bending test at three points. Therefore, according to the classical theory of solid mechanics, the stress generated in the specimens must be determined following Equation 3 [34].

Where M is the maximum moment generated in the specimen, c is the outermost point in the specimen from its center of mass and I is the inertia of the specimen.
As an adaptation of the standard and following the theory of solid mechanics, the applied stress was determined with Equation 4.
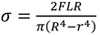
Where F is the load applied and L is the length of the specimen. R is the outermost radius in the specimen and r is the innermost radius in the specimen.
2.4. Interfacial Adhesion Test
The interfacial shear strength (IFSS) was determined as a measure of the quality of the adhesion between the abutments and PMMA provisionals; this with universal testing machine, as described: the abutments with their respective PMMA provisionals were located and held by the lower side from the analog in a vertical position; a traction force was applied over the provisional until failure. This process was repeated 6 times with the experimental abutments and 6 times with the abutments of the control group.
Regarding the interfacial adhesion test (Pull-out), until now there is not standard that standardizes the interfacial adhesion tests between two materials of the nature of the study materials. However, there are various methods that have been tested and published [35], which may be adaptable to evaluate the interfacial adhesion between the polymer-made abutment and the resin that will bond the provisional tooth. The classical theory of solid mechanics [34] is followed, where the necessary shear stress is estimated to overcome the union of the materials (Equation 5).

Where τ, is the shear stress, F, the applied loadand as is the joint area between the pieces or materials, As = 2πRL.
3. RESULTS
By means of the stress-strain curves, the mechanical properties in compression, flexion and pull-out of the temporary photopolymeric resin abutments and the control abutments in PEEK were determined and compared.
To contrast the results, it was proposed as a null hypothesis that by comparing mechanical properties in compression, bending and adhesion to PMMA of both types of abutments there are no significant statistical differences.
Statistical analysis was performed with STATA 14 software (version 14, Statacorp, USA). The data were analyzed by means of the Wilcoxon Mann-Whitney test, as these were two independent samples of reduced size.
Values lower than (p <0.05) were considered statistically significant in all tests and reject the null hypothesis of equality between the group medians.
3.1. Compression Test
Fig. (2) shows the stress vs. strain representative curves obtained from the compression tests performed on temporary abutments made with photopolymeric resin and commercial abutments in PEEK. As can be seen, the curve of the CLEAR FLGP04 abutments present, first an increase in the applied stress, followed by a step, in which the quasi-constant stress is maintained (appearance of a first failure due to compression); subsequently, there is an increase in stress until the strength of the abutment, stress in which the sample presents the failure. This behavior could be attributed to the high rigidity of CLEAR FLGP04 resin (modulus of elasticity of 2.8 GPa), which facilitates the formation of microcracks in the material. The PEEK abutments showed a constant increase in stress until the strength of the abutment, where failure occurs due to compression load, subsequently, the stress decreases slowly, an event caused by buckling after failure.
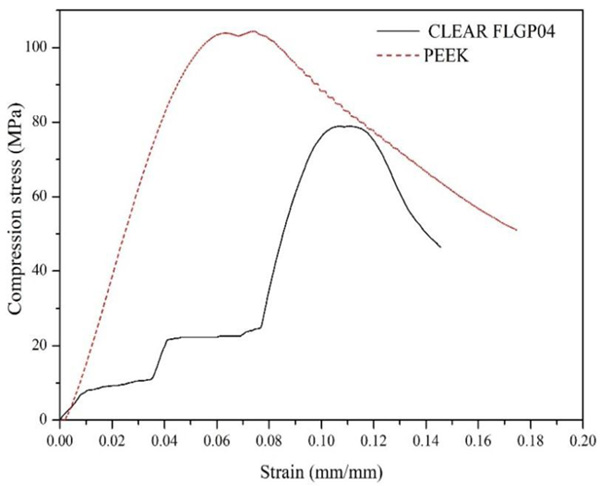
Fig. (3) shows the averages and standard deviation of the values obtained for compression strength. Six (6) samples were used for each type of abutment. Here it was possible to determinate that the abutments made with photopolymeric resin have an average compressive strength of 84.50 MPa, while the commercial abutments presented an average value of 114.41 MPa. The standard deviation was 8.93 and 8.54 MPa for resin and commercial abutments, respectively. From the above values, the null hypothesis (there are no significant statistical differences) is rejected with a p-value of 0.0039. Therefore, it is possible to say that there is a significant difference between the compressive strength values of CLEAR FLGP04 and PEEK abutments.
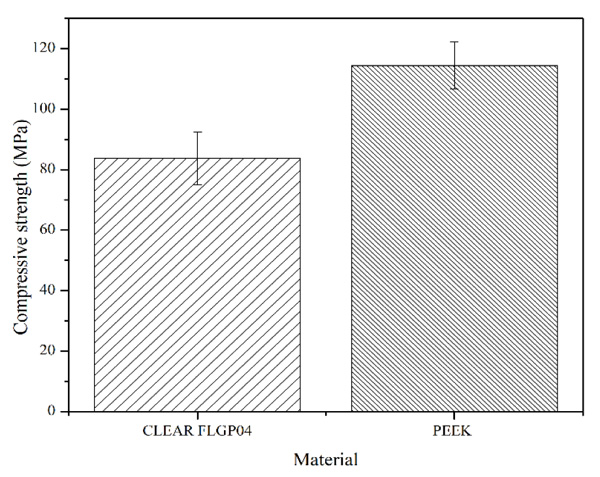
Fig. (4) shows the type of failure presented in the abutments (PEEK and CLEAR FLGP04) when they were subjected to compressive loads. Here it was observed that the failure mechanism of the PEEK abutments initially presented a compression failure at its base, followed by a buckling of the sample. The abutments made of CLEAR FLGP04 resin failed only due to compressive loads.
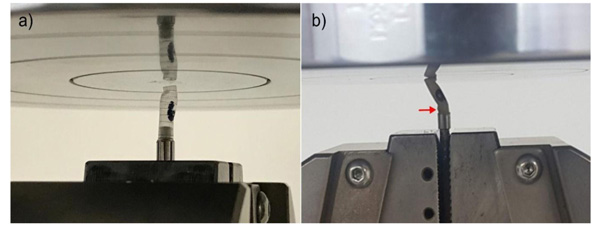
3.2. Bending Test
Fig. (5) shows the representative stress vs. strain curves of the bending tests of the evaluated abutments. Here it is possible to observe that the abutments made with PEEK presented, in addition to greater bending strength, an important plastic zone (characteristic of a ductile material), with a strain at fracture of 0.10 ± 0.022 mm/mm. In addition, it was possible to observe that the CLEAR FLGP04 abutments lack said pronounced plastic zone and their strain in the fracture was 0.068 ± 0.013 mm/mm, which confirms the rigid behavior of the CLEAR FLGP04 resin.
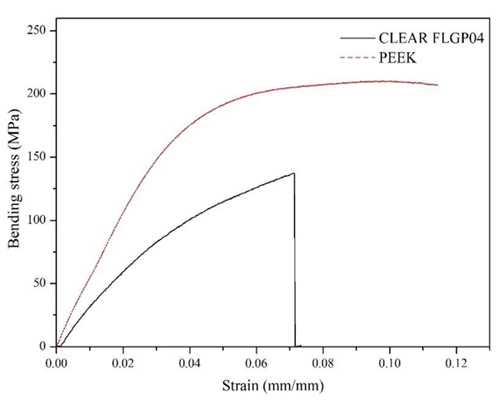
Fig. (6) shows the averages and standard deviation of the values obtained for bending strength. Six (6) samples were used for each type of abutment. Here it was possible to determinate that the abutments made with photopolymeric resin have an average bending strength of 120.92 MPa, with a standard deviation of 12.05 MPa; while the commercial abutments presented an average value of 205.2 MPa and the standard deviation was 6.59 MPa. From the previous values, the null hypothesis is rejected (there are no significant statistical differences) with a p-value of 0.0209. Therefore, it is possible to say that there is a significant difference between the bending strength values of CLEAR FLGP04 and PEEK abutments.
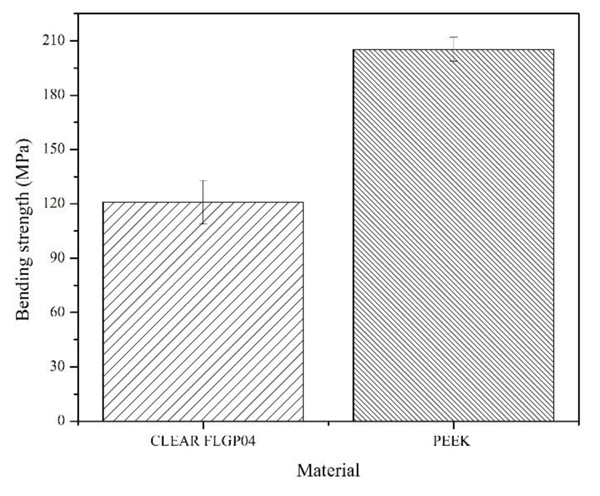
Fig. (7) shows the type of fracture presented by the abutments (PEEK and CLEAR FLGP04) when they were evaluated under cantilever-type bending loads. In both cases, the failure occurred at the base of the abutment (connection zone between the abutment and the provisional). This behavior is expected because the maximum moment occurs in said connection zone, which is generated by the application of the load at the opposite end. In addition, the high rigidity of the CLEAR FLGP04 abutment is evidenced, as it fails at low deflection; While in the PEEK abutment, a pronounced deflection could be observed at the instant of failure, this due to its plastic behavior, which is evidenced in its stress vs strain curve (Fig. 5).
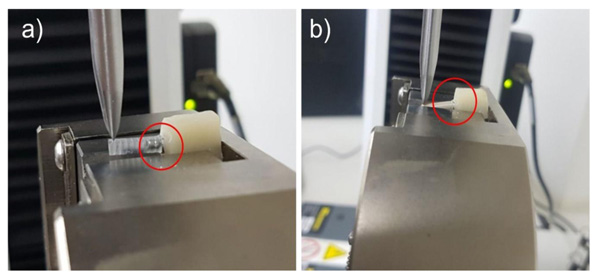
3.3. Interfacial Adhesion Test
Fig. (8) shows the shear stress vs extension curves obtained from the pull-out test performed between the abutments and provisionals made of PMMA. Here it is possible to observe that the interfacial debonding pattern of the samples with PEEK abutments present low values of interfacial shear strength (IFSS) compared to those obtained in samples with CLEAR FLGP04 abutments, in addition, an increase in shear stress is evidenced until maximum stress, in which the interface fails; subsequently, there is a gradual debonding of the abutment that manifests itself as a decreasing saw pattern in the stress vs extension curve. For its part, the pattern of the curve of the samples with CLEAR FLGP04 abutments shows a first increase in stress up to approximately 1.9 MPa, where there is a slight decrease in stress, then the stress increases with a stress/extension ratio less than in the first zone of the curve. Subsequently, the stress increases rapidly to a new zone of the curve where the stress remains quasi-constant until sample failure occurs. This behavior is attributed to the mechanical anchoring that is promoted by the “veins” that surround the outer diameter of the resin abutments (Fig. 7a); this type of anchoring is evidenced in the step pattern of the stress vs extension curve. In this sense, it is likely that an interfacial crack has occurred in the first decrease in stress. However, the sliding between the materials will have a greater restriction due to the presence of “veins”.
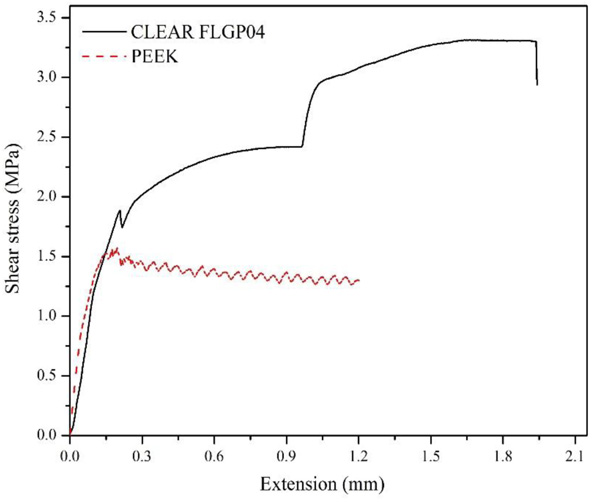
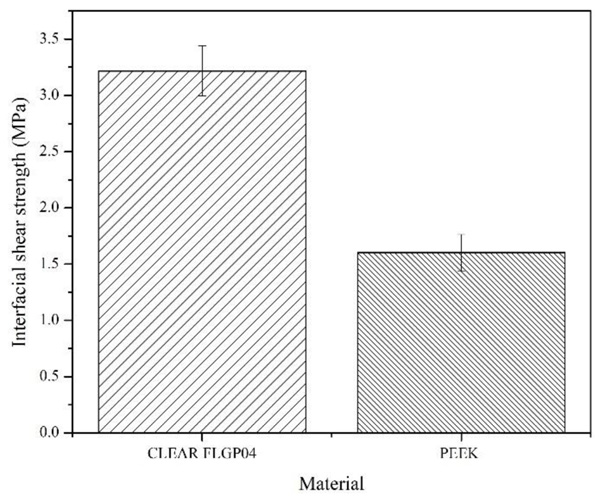
Fig. (9) shows the averages and standard deviation of the values obtained for IFSS. Six (6) samples were used for each type of abutment. Here it was possible to determine that the abutments made with photopolymeric resin have an average interfacial shear strength of 3.22 MPa, while the commercial abutments presented an average value of 1.6MPa. The standard deviation was 0.22 and 0.162 MPa for resin and commercial abutments, respectively. From the above values, the null hypothesis (there are no significant statistical differences) is rejected with a p-value of 0.039. Therefore, it is possible to say that there is a significant difference between the IFSS values of CLEAR FLGP04 and PEEK abutments.
Fig. (10a) shows the type of failure presented during the pull-out tests in the abutments made with PEEK; the sample shows a sliding-type debonding, since it leaves the zone near the connection between the provisional and the abutment uncovered, without apparent evidence of PMMA fragments attached to the abutment. Fig. (10b) shows the fracture zone of the abutment made with CLEAR FLGP04 resin, where the abutment does not fail due to shear stresses in the interfacial zone, but rather due to tensile stresses at the base of the abutment.
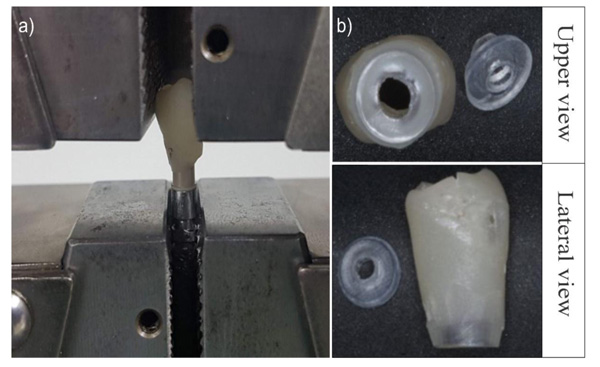
4. DISCUSSION
It is possible to design and manufacture abutments for temporalization in implant rehabilitation using different design platforms. In this work, the OnShape program was used with the Formlabs 2 printer to manufacture abutments in CLEAR FLGP04 by 3D printing with a stereolithography technique, achieving good results as revealed in reports the possibility of using this technology to manufacture devices with high definition [36].
Currently, light-curing resins are available for manufacturing [37–39] dental and medical devices such as Dental Model FLDMBE02 (Formlabs, Massachusetts, USA), Dental Sg FLDGOR01 (Formlabs, Massachusetts, USA) and Dental Lt Clear FLDLCL01 (Formlabs, Massachusetts, USA) have been used in the impression of study models, orthodontic retainers and surgical guides11; with properties similar to the resin used in this work and with adequate biocompatibility, which could be used in the manufacture of temporary abutments.
The CLEAR FLGP04 and PEEK abutments presented fractures when subjected to mechanical tests. PEEK abutments showed better performance, presenting buckling failure in the compression test, resisting more than 100 MPa, while the CLEAR FLGP04 abutment supported compressive stresses of around 84 MPa where the buckling failure occurred.
In this study, it was found that the temporary abutments made of PEEK bonded to PMMA and subjected to traction presented adhesive failures, which is consistent with the reported by Gama et al. (2020), where it was found that, although high-performance polymers such as PEEK have good mechanical properties, the same does not happen with their adhesive properties, which require a pre-treatment or conditioning of the surfaces to achieve adequate adherence to resinous materials [40].
In contrast, the temporary abutments made of CLEAR FLGP04 bonded to PMMA suffered cohesive failures when subjected to traction, thus demonstrating a stronger bond between both materials, which resulted in a fracture of the abutments in their cervical area.
The good adhesive properties shown by CLEAR FLGP04abutments open a new window of opportunity for the manufacture of abutments in a material other than PEEK. The PEEK abutment adhesive failures presented have been reported and attributed to the low possibility of direct adhesion to acrylic materials; Schmidlin et al, warned that not much attention has been paid to this material in restorative dentistry “... basically due to the difficulties to establish a strong and lasting adhesion to composite resin materials” and recommended the use of an adhesive of a hydrophobic nature [14]. In recent years, different investigations have been carried out aimed at improving the possibility of adhesion to acrylic materials, however the chemical structure of PEEK is very stable and has low surface energy, which makes it necessary to perform surface treatments to allow its bond to an adhesive allowing the union to the acrylic material [14,41]. Among the modifications proposed to improve the adhesive properties of PEEK, the following have been studied: abrasive air (aluminum oxide), 98% sulfuric acid, 9.6% hydrofluoric acid, argon plasma, UV rays, laser techniques, among others, followed by epoxy type adhesives [41].
Several of the surface treatments proposed to improve the adhesion of PEEK be effective in allowing the bonding of acrylic adhesives, the most recommended being 98% sulfuric acid, but some doubts remain about the mechanism. Some authors have proposed that this acid induces the formation of sulfonated groups (-SO3), which would allow bonding to methylmethacrylate adhesives by chains crosslink, while others do not recognize the formation of these groups and propose the formation of microporosity that allow the mechanical retention of methyl methacrylate [42].
Although sulfuric acid has been recognized as an effective method to improve the adhesive properties of PEEK, its use has been questioned due to that its high concentration can structurally weaken the material, and its use can be a safety risk in the dental office [14,42].
CONCLUSION
The use of new technologies such as computer design and 3D printing made possible the elaboration of provisional abutments for implant rehabilitation using a photopolymeric resin (CLEAR FLGP04) that showed mechanical performances in compression flexion and adhesion that allow its potential use as an alternative to prefabricated abutments made of PEEK.
AUTHOR CONTRIBUTIONS
Conceptualization, C.H.V.LL, M.L.R.P.; methodology, C.H.V.LL, M.L.R.P, M.A.B.P; I.M.V; C.A.V.R; and M.F.M.V.; investigation, M.A.B.P; I.M.V; C.V; and M.F.M.V; writing-original draft preparation, M.A.B; I.M.V; C.A.V.R; and M.F.M.V..; writing-review and editing, MC.H.V.LL, M.L.R.P, M.A.B.P and M.F.M.V.; funding acquisition, M.L.R.P, and C.H.V.LL.
ETHICS APPROVAL AND CONSENT TO PARTICIPATE
The ethical approval for this study was obtained by the Human Ethics Review committee of the Universidad del Valle, Cali Colombia by Act No. 010-018 of 05/22/2018.
HUMAN AND ANIMAL RIGHT
No Animals were used in this research. All human research procedures followed were in accordance with the ethical standards of the committee responsible for human experi- mentation (institutional and national), and with the Helsinki Declaration of 1975, as revised in 2013.
CONSENT FOR PUBLICATION
Not applicable.
AVAILABILITY OF DATA AND MATERIALS
Not applicable.
FUNDING
This research was funded by Vicerectoria de Investi- gaciones Universidad del Valle and Pontificia Universidad Javeriana. Cali. Colombia. Grant CI 1846 UNIVALLE
CONFLICT OF INTEREST
The authors declare no conflict of interest.
ACKNOWLEDGEMENTS
The authors are thankful to the Department of Atonation and Electronics, Universidad Autonoma de Occidente, Cali, Colombia for their support.