All published articles of this journal are available on ScienceDirect.
Effect of Static Compressive Force on Aldehyde Dehydrogenase Activity in Periodontal Ligament Fibroblasts
Abstract
Background:
The application of static compressive forces to periodontal ligament fibroblasts (PDLFs) in vivo or in vitro has been linked to the expression of biochemical agents and local tissue modifications that could be involved in maintaining homeostasis during orthodontic movement. An approach used for identifying mesenchymal cells, or a subpopulation of progenitor cells in both tumoral and normal tissues, involves determining the activity of aldehyde dehydrogenase (ALDH). However, the role of subpopulations of PDLF-derived undifferentiated cells in maintaining homeostasis during tooth movement remains unclear.
Objective:
This study aimed at analyzing the effect of applying a static compressive force to PDLFs on the activity of ALDH in these cells.
Methods:
PDLFs were distributed into two groups: control group (CG), where fibroblasts were not submitted to compression, and experimental group (EG), where fibroblasts were submitted to a static compressive force of 4 g/mm2 for 6 hours. The compressive force was applied directly to the cells using a custom-built device. ALDH activity in the PDLFs was evaluated by a flow cytometry assay.
Results:
ALDH activity was observed in both groups, but was significantly lower in EG than in CG after the application of a static compressive force in the former.
Conclusion:
Application of a static compressive force to PDLFs decreased ALDH activity.
1. INTRODUCTION
The remodeling of tooth-supporting tissues following orthodontic movement is closely linked to the metabolism of osteoblasts and periodontal ligament fibroblasts (PDLFs). When submitted to compressive or tensile forces, these cells undergo a mechanotransduction process, whereby they become capable of expressing intra- and extra-cellular biomechanical signals [1, 2]. The exact role played by compression has yet to be completely understood with respect to the role of cell subpopulations, particularly those of undifferentiated cells, because there is still no evidence for whether or not using compressive force could increase the number of cells in these subpopulations. It has been found that the use of lower levels of pressure on the periodontal ligament (PDL) could be more effective in maintaining the bone level, a finding that, if confirmed, would be particularly relevant for orthodontic treatment; however, possible correlations between this observation and the behavior of undifferentiated cells from the PDL still require investigation [3, 4].
Fibroblasts are mature mesenchymal cells that are particularly abundant in the connective tissue of every organ. Therefore, these cells are the most frequently involved cell phenotype “contaminating” several cell culture systems [5]. In any cell culture, it is not only difficult to apply techniques to successfully eliminate fibroblasts, but it is also particularly challenging to distinguish them from mesenchymal stromal cells (MSCs). Fibroblasts and MSCs have extremely similar morphological characteristics; they both proliferate well and have many identical cell-surface markers [6]. Furthermore, MSCs lack a specific surface antigen capable of distinguishing them from fibroblasts. Stro-1, and, more recently, CD146, have been found to be specific markers for MSCs [7-9]. However, these markers seem limited to MSCs derived from bone marrow (BM-MSCs) or from renal tissue, since adipose-derived MSCs (AD-MSCs), for instance, do not display these markers [10]. In spite of these differences, MSCs from different tissular origins possess very similar phenotypic profiles. They usually express high levels of mesenchymal markers, and generally do not express hematopoietic markers [10, 11]. To date, the most effective way of distinguishing MSCs from fibroblasts is to analyze their functional properties; MSCs self-renew and retain multipotent differentiation capacity, whereas fibroblasts seem more limited with respect to both of these functional capabilities [6].
In 1999, Storms et al. [12] characterized an aldehyde dehydrogenase–bright (ALDH[br]) population in the blood of the human umbilical cord, enriched for hematopoietic progenitors. By employing this technique, stem or progenitor cells were characterized in human bone marrow and umbilical cord blood.
Aldehyde dehydrogenase (ALDH) is a cytosolic enzyme, participating in the intracellular oxidation of aldehydes, and converts retinol into retinoic acid [13-15]. An approach used for identifying mesenchymal cells, or a subpopulation of progenitor cells, in tumoral and normal tissues, involves determining the activity of this enzyme. ALDH acts mainly in the final phase of cell differentiation [14], and, according to Moreb et al. [16], its activity can be understood as the percentage of viable and intact cells positively marked by flow cytometry.
The aim of this study was to analyze the effect of applying a static compressive force to PDLFs on the activity of ALDH in these cells.
2. MATERIALS AND METHODS
2.1. Study Design
This study was approved by the Research Ethics Committee of the Federal University of São Paulo (UNIFESP; approval no. 411.270), and was conducted in compliance with both the guidelines of Resolution no. 196/96, National Health Council, Ministry of Health (Brazil), and with the Helsinki Declaration of 1975, as revised in 2008, on the ethical standards for human experimentation. All of the participants signed a free and informed consent agreement before taking part in the study.
Ten patients aged 18 to 30, both male and female, non-smokers, without any history of systemic disease or use of medication, and with completely impacted third molars were selected. Two teeth were extracted from each patient (20 teeth total), and the fibroblasts from their PDL were harvested and expanded in culture.
2.2. Harvesting of PDL
The extracted teeth were placed in 15-mL sterile conical tubes (Corning, MA, USA) containing 5 mL of transport solution consisting of HBSS, and complete alpha-MEM supplemented with 5,000 IU of penicillin + 10,000 μg of streptomycin + 25 μg of amphotericin-B (Gibco, Grand Island, NY, USA).
The teeth were washed four times with HBSS (Sigma Aldrich, St. Louis, MO, USA) and antibiotics (5,000 IU of penicillin + 10,000 μg of streptomycin + 25 μg of amphotericin-B; Gibco,). PDL fragments were obtained by scraping the middle third of each tooth root.
The collected fragments were washed sequentially in four 15-mL conical tubes (Corning) containing 4 mL of HBSS solution supplemented with the same composition of antibiotics and antifungals described above, for 30 s each tube. The periodontal tissue was then transferred into a 15-mL conical tube containing 5 mL of alpha-MEM, 1 mg/mL of Type II collagenase (Gibco), and 1 mg/mL of dispase (Gibco), under heating (37°C), and then shaken using a TE-420 orbital shaker for 30 minutes (Tecnal Equipamentos Científicos, Piracicaba, SP, Brazil). Next, 5 mL of alpha-MEM (Lab Biotecnologia, São Paulo, SP, Brazil) supplemented with 10% bovine fetal serum (Gibco) was added to neutralize the action of collagenase and dispase enzymes. The supernatant was discarded, and the remaining solution was transferred to another 15-ml tube and centrifuged (Excelsa II; Fanem, Guarulhos, SP, Brazil) at 100 g for 6 minutes, at room temperature.
The supernatant was discarded and the cell pellet was resuspended in 5 mL of complete alpha-MEM culture medium. The number of cells was counted using an automatic cell counter (Countess; Invitrogen, Seoul, Korea). The solution containing the PDL cells was kept in a 25 cm3 culture flask (Corning) in a CO2 incubator (Elite II; Revco, Rio de Janeiro, RJ, Brazil) at 37°C, with 5% CO2 and 95% O2. The culture medium was changed every 48 hours for an average of 20 days, until a confluency of 80% was reached. The cells were then trypsinized using 0.25% trypsin with 0.02% EDTA (Sigma Chemical, Saint Louis, MO, USA) and subcultured. After the fifth passage, the cells were cryopreserved at –130°C, at a density of 1 x 106 cells/mL in alpha-MEM, supplemented with 50% inactivated FBS, 0.1% bovine serum albumin (Sigma Chemical, Saint Louis, MO, USA) and 10% dimethyl sulfoxide (Sigma Chemical).
2.3. Study Groups
At a density of 2 x 105 cells/mL, the fibroblasts were seeded onto the center of each well of a six-well plate and distributed into the following groups: control group (CG), where fibroblasts were not submitted to compression, and experimental group (EG), where fibroblasts were submitted to a static compressive force of 4.0 g/cm2. Both groups were analyzed after 6 hours, and all of the experiments were performed in triplicate.
2.4. Compressive Force Application
A model similar to that proposed by Nishijima et al. [17] and Nakajima et al. [18] was used to produce compression. Acrylic cubic containers measuring 2 x 2 x 2cm were placed over the cells in the center of each well of the culture plate, and stainless steel spheres were placed in the containers to produce a force corresponding to a mass of 16 g. A concentration of 2 x 105 cells/well diluted in 200 μL of culture medium was seeded onto the center of each well of a six-well plate, where it remained for 10 minutes for fixation, and the volume of the culture medium in each well was completed immediately afterwards, until it reached 3.0 mL. The cells were pre-incubated for 6 hours in a culture medium containing 10% fetal bovine serum, and only then was the compression applied to the cells for 6 hours in an incubator. After application of the compressive force, the cells were trypsinized and prepared for flow cytometry analysis.
2.5. ALDH Activity
The Aldefluor kit (Stemcell Technologies, Vancouver, BC, Canada) was used to stain the cells with ALDH. This is a reagent kit that is used to identify human cells that express high levels of this enzyme. The activated reagent, BODIPY-aminoacetaldehyde (BAAA), is a fluorescent non-toxic substrate for ALDH, which freely diffuses into intact and viable cells. BAAA is converted into BODIPY-aminoacetate (BAA) in the presence of ALDH, and BAA is then retained inside the cells. The amount of fluorescent reaction product is proportional to the ALDH activity in the cells, and is measured using a flow cytometer. A specific inhibitor of ALDH, diethylaminobenzaldehyde (DEAB), is used to control for background fluorescence. The cells were resuspended at a concentration of 1 x 106 cells/mL, using the buffer solution of the kit. Two 5-mL tubes for flow cytometry were separated and identified as test and control. In the control tube, 5 µL of DEAB was added to the tube, and the previously separated cells were placed at the previously described concentration. Subsequently, 5 µL of the homogenized ALDH solution was added, and 500 µL of this solution was quickly collected and dispensed into the control tube. The tubes were then incubated in the dark for 40 minutes at 37°C. After incubation, the tubes were centrifuged at 200 g for 5 minutes, the supernatant was discarded, and the cultures were resuspended in 300 µL of the buffer solution of the kit. The specimens were distributed onto a 96-well plate that is specific for the Guava flow cytometer, and then analyzed.
3. RESULTS
ALDH activity was observed in both groups, but was significantly lower in EG than in CG after the application of a static compressive force to the former. Fig. (1) shows the data obtained from one of the donors enrolled in the study.
All of the experiments were performed in triplicate, and the counts of fibroblasts marked with ALDH, as determined in the cytometry analysis, are represented in Table 1.
4. DISCUSSION
Orthodontic mechanotransduction is the mechanism by which periodontal cells convert mechanical stimuli into electrochemical activity during induced tooth movement [19]. This form of sensory transduction is responsible for a series of physiological senses and processes in the body, including proprioception, touch, balance and hearing [20], and induces the production and release of molecules capable of regulating and normalizing local bone homeostasis [21]. The specific cell responses to mechanical signals must be understood in order to interpret this mechanism appropriately, and thus enable reducing bone loss, promoting differentiation of osteogenic cells, and increasing bone formation [22].
The mechanotransduction mechanism basically involves the conversion of mechanical signals into electrical or chemical signals. In this process, ion channels mechanically blocked as a result of pressure or movement lead to a change in specialized sensory cell excitability and sensory neurons [22-25]. Stimulation of a mechanoreceptor allows mechanically sensitive ion channels to open and produce a transduction current that alters cell membrane potential [26, 27].
However, owing to the lack of instruments capable of investigating the response of periodontal ligament cells in vivo and in situ, this issue remains unsolved; therefore, different types of in vitro models have been proposed for this purpose. The static compressive force model used in this study to analyze cell behavior in vitro consisted of acrylic cubic containers measuring 2 x 2 x 2 cm and containing steel spheres to create a compressive load directly onto the cells. The same concept was used by Nakagima et al. [18], who demonstrated the possibility of studying the effect of compressive forces in vitro, by simulating physiological and pathological conditions.
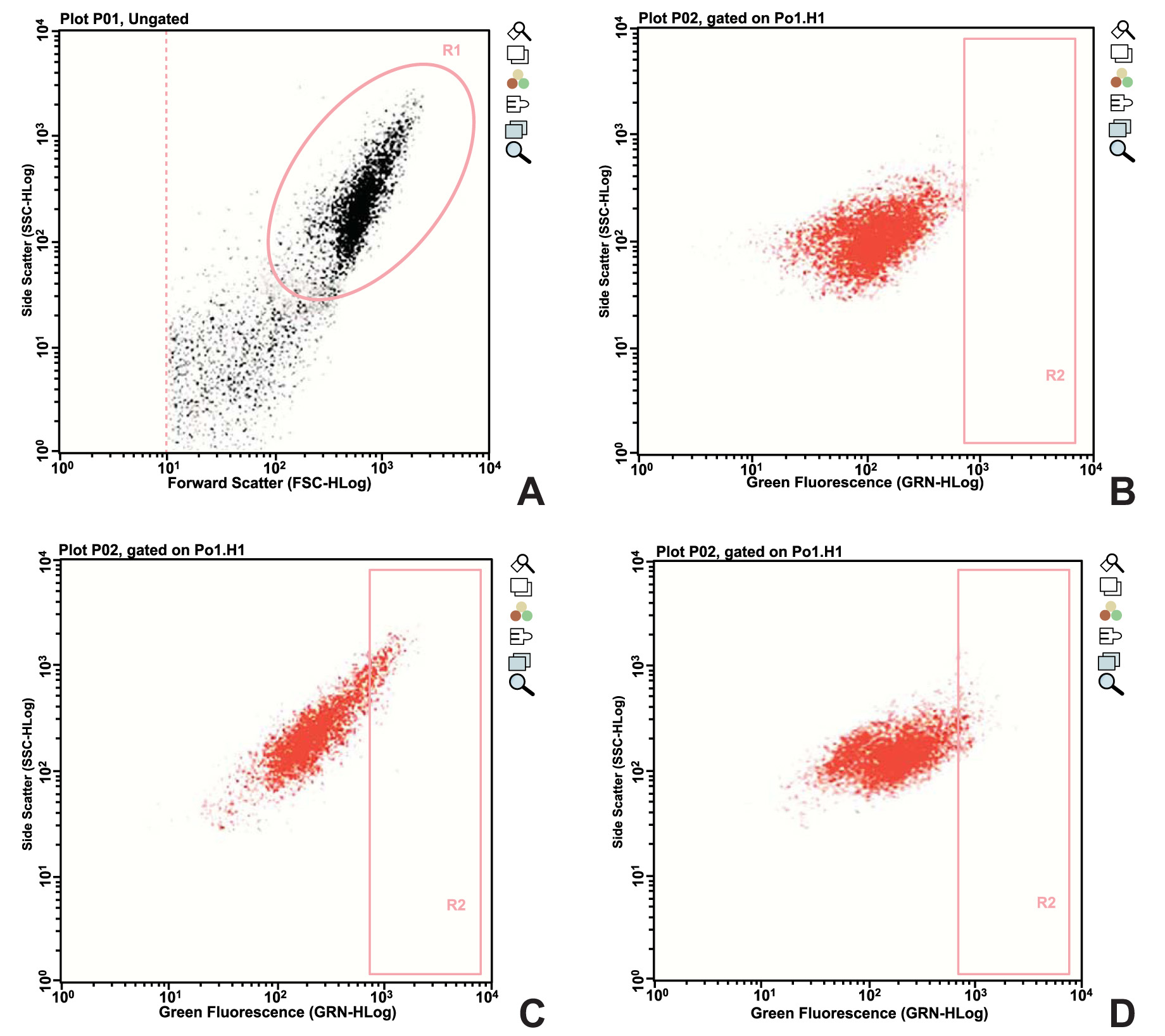
Donor | Control group | Experimental group |
---|---|---|
1 | 1.45 | 2.20 |
2 | 2.22 | 2.77 |
3 | 2.41 | 0.90 |
4 | 7.40 | 3.08 |
5 | 4.67 | 2.92 |
6 | 5.15 | 0.49 |
7 | 9.60 | 4.72 |
8 | 6.00 | 4.17 |
9 | 6.37 | 0.79 |
10 | 8.06 | 1.81 |
Mean | 5.33 | 2.38 |
Median | 5.58 | 2.49 |
Standard deviation | 2.69 | 1.42 |
p-value | 0.004 |
The compressive force of 4g/cm2 for 6 hours used in the present study was determined after performing a previous pilot study using the same custom-built force device with a wide range of force/time combinations. The criteria used in this pilot study were the highest cell viability and lowest rate of cell necrosis and apoptosis, both of which were analyzed by flow cytometry. Therefore, the 4g/cm2 / 6 hour combination was found to be the intensity and duration of force capable of stimulating cellular change without causing damage, and this combination was assumed to be equivalent to the in vivo conditions occurring during the application of light orthodontic force during tooth movement.
Evidence that undifferentiated mesenchymal cells are present in periodontal tissues was first provided by the histological study conducted in vivo by McCulloch et al. [28]. The complex series of events involved in periodontal regeneration include recruitment of locally derived progenitor cells, which can subsequently differentiate into periodontal ligament-forming cells, mineral-forming cementoblasts or bone-forming osteoblasts. Trubiani et al. [29] observed that the periodontal ligament can be an easily accessible source of autologous stem cells, which have a high expansion capacity, and which are able to differentiate into osteogenic cells capable of colonizing a recipient site and expanding when connected to a bio-compatible scaffold. Hence, the use of PDL-MSCs for generating graft biomaterials has been suggested as an option for bone tissue engineering in regenerative dentistry [30].
Choi et al. [31] used immunophenotyping and fluorescence-activated cell-sorting analysis to identify undifferentiated subpopulations, and found that certain MSC markers (CD44, CD73, CD90, CD146, and CD166) were heavily expressed in human adult dental pulp cells (hDPCs), periodontal ligament stem cells (hPDLSCs) and gingival fibroblasts (hGFs). On the other hand, ALDH, another marker of cell differentiation in tumoral cells, has been used to characterize undifferentiated healthy cells [32-35].
Recently, major new hypotheses have intensified the search for a more practical way of identifying stem cells. According to the concept of stem cell plasticity, somatic stem cells are thought to regenerate and repair different types of tissues. Another hypothesis is that cancer behaves like an organ, with its own sustaining cancer stem cells (CSC). Stemness markers or genes are widely sought after. For years now, ALDH has been known to be highly expressed in hematopoietic stem cells (HSC) [36, 37], and to provide protection against alkylating agents of the oxazaphosphorine family, such as cyclophosphamide and its derivatives [38-41]. Determining ALDH activity as the core of a flow cytometry-based method to sort hematopoietic progenitors has paved the way to study high ALDH activity as a marker for stem cells in different tissues [42].
As an ALDH inhibitor, DEAB was used as a negative control in the Aldefluor assay, and was originally viewed as a specific inhibitor of the ALDH1 family; hence, the ALDH enzyme activity measured with this assay was assumed to reflect the expression of ALDH1 isozymes [43]. However, recent data indicate that DEAB is not a specific inhibitor when assayed in vitro versus the ALDH1, ALDH2, and ALDH3 family members [44]. Several studies have correlated ALDH(br) cells, as assessed using the Aldefluor reagent, with cells staining positive with ALDH1-targeting antibodies in various tissues and cell populations, such as human breast epithelium [45] and MSCs [46]. However, many reports have failed to clarify the question of isozyme specificity due to the limited information about this specificity in both the Aldefluor assay, and in assays using ALDH-targeting antibodies.
ALDH activity was used in the present study as a functional marker for undifferentiated periodontal ligament fibroblasts, aiming to gain a better understanding of the role played by this subpopulation of cells in the homeostasis of tooth movement. Some ALDH activity was observed in both groups, but it was significantly higher in CG than in EG, where a compressive force was applied. This outcome shows that there is a decrease in ALDH activity even when the compressive force is within a range compatible with minimum damage to fibroblasts, i.e., it demonstrates that compressive forces cause functional damage, irrespective of whether or not the cell viability rate remains high.
Although this study successfully identified an ALDH+ subpopulation in PDL fibroblasts, the exact mechanism whereby the observed decrease occurred after applying force remains to be investigated. Furthermore, this study examined the expression of ALDH in an unselected population of PDL fibroblasts, as opposed to an exclusively ALDH+ subpopulation. Therefore, further investigation is warranted to address these issues, and further elucidate how functional markers such as ALDH and its isoforms can contribute to improving the knowledge of mechanotransduction.
CONCLUSION
The continuous static compressive force used in this study was found to decrease the counts of human periodontal ligament fibroblasts marked for ALDH.
AUTHOR'S CONTRIBUTIONS
Antonio C. Aloise conceived and designed the experiments. Fabio Schemann-Miguel and Silvana Gaiba performed the experiments, and analyzed the data. Lydia M. Ferreira contributed to conceiving and designing the experiments, and also provided reagents, materials, and analytical tools. All of the authors reviewed and approved the final version of the manuscript.
ETHICS APPROVAL AND CONSENT TO PARTICIPATE
This study was approved by the Research Ethics Committee of the Federal University of São Paulo, Brazil (UNIFESP; approval no. 411.270).
HUMAN AND ANIMAL RIGHTS
The study was conducted in compliance with both the guidelines of Resolution no. 196/96, National Health Council, Ministry of Health (Brazil), and with the Helsinki Declaration of 1975, as revised in 2008, on the ethical standards for human experimentation.
CONSENT FOR PUBLICATION
All of the participants signed a free and informed consent agreement before taking part in the study.
AVAILIBILITY OF DATA AND MATERIALS
Not applicable.
FUNDING
None.
CONFLICT OF INTEREST
The authors declare that they have no conflict of interest financial and otherwise.
ACKNOWLEDGEMENTS
Declared none.