All published articles of this journal are available on ScienceDirect.
Efficacy of MTA Modified by Nanosilver for the Prevention of Coronal Leakage
Abstract
Background:
Numerous materials have been introduced as coronal barriers, however, they have shown various degrees of microleakage. Therefore, attempts are undertaken to introduce more reliable materials with the potential to provide a long-term coronal seal.
Introduction:
This in vitro study aimed to assess the efficacy of gray ProRoot Mineral Trioxide Aggregate (MTA) modified by a suspension of silver nanoparticles (AgNPs) as an orifice plug using a bacterial leakage method.
Methods:
40 extracted human teeth were prepared and filled (except for the negative control group) using the lateral compaction technique. The coronal 2 mm of the root filling was removed in the experimental groups. The teeth were randomly divided into two experimental (n = 15) and two control (n = 5) groups. In the experimental group 1 (MTA group) and the experimental group 2 (Ag-MTA group), MTA modified by a suspension of AgNPs was used as an orifice plug. In the negative control group, the entire root surfaces were covered with two layers of nail varnish. In the positive control group, the root canals were filled with a single gutta-percha cone without a sealer and no orifice plug. Bacterial leakage was assessed using a two-chamber system. The teeth were incubated at 37°C and 100% humidity for 120 days, and human saliva was added to the samples every 3 days. Bacterial microleakage was assessed by daily monitoring and observating the turbidity of the Brain Heart Infusion (BHI) broth. The data were analyzed using the log-rank test.
Results:
All positive controls showed contamination after 5 days. None of the negative controls were contaminated during the experiment. The Ag-MTA group demonstrated a significantly better coronal seal than the MTA group (p = 0.031).
Conclusion:
Gray ProRoot MTA modified by AgNPs has the potential for being used as an orifice plug in endodontically treated teeth.
1. INTRODUCTION
Coronal leakage of bacteria and their products is the main cause of the occurrence and progression of periradicular diseases [1]. Three-dimensional filling of the root canal system is an important factor for the success of endodontic treatment since it can prevent the leakage of microorganisms and their toxins from the oral cavity to the periradicular tissues [2, 3].
Coronal microleakage is probably the most important factor responsible for the failure of endodontically treated teeth [4]. It is assumed that the loss of coronal seal is responsible for the reinfection of the root canal system after root canal therapy and can lead to treatment failure [5]. Thus, the long-term prognosis of endodontically treated teeth highly depends on the quality of the final restoration [6].
Several factors may cause reinfection of the root canal system, such as delay in permanent restoration of the tooth, fracture of the tooth or coronal restoration, insufficient thickness of the temporary restoration, inadequate seal by the temporary restoration, recurrent caries, and restorations with improper margins [3, 4, 7]. Furthermore, the duration of exposure to the oral cavity and the type of restorative material used can affect coronal leakage [8].
Previous studies have shown that the quality of coronal restoration has a greater effect on the periradicular tissue status than the quality of endodontic treatment. It has been reported that bacterial products can be seen at the apex of endodontically treated teeth without a coronal restoration 3 months after their endodontic treatment [9].
Yamauchi et al. reported that the use of coronal plug following endodontic treatment decreased the risk of occurrence of periapical periodontitis [10]. Moreover, the placement of an orifice plug has been suggested [5, 8] immediately after endodontic treatment for the coronal seal of the root canal system. Several materials have been proposed for use as an extra layer to protect the obturated root canal against microleakage and provide an optimal coronal seal following endodontic treatment and prior to temporary restoration of the teeth [8].
Materials used for orifice plug must have easy and fast handling, provide a high-quality seal, and yield high bond strength [6]. Several filling materials have been recommended for use as orifice plugs, such as Cavit, composite resin, ProRoot MTA, and Super EBA, with controversial results. Due to the high resistance against leakage, high adaptation, and high biocompatibility, ProRoot MTA has attracted more attention than other materials for this purpose [4, 8, 11].
The results of studies on the antibacterial and antifungal properties of ProRoot MTA have been contradictory. However, it is believed that this material has limited antimicrobial activity against some microorganisms [11]. Evidence shows that ProRoot MTA does not have antibacterial activity against Candida albicans, Staphylococcus aureus, Streptococcus mutans, Enterococcus faecalis (E. faecalis), Escherichia coli, or Streptococcus sanguinis. Moreover, it has no antibacterial activity against anaerobes either. However, it has antibacterial properties against some facultative bacteria [11, 12].
Silver nanoparticles (AgNPs) are increasingly used in medicine for the production of disinfecting agents. Increased surface area (for more efficient contact) and surface charge density of nanoparticles increase their interference with the bacterial cell membrane and inhibit their antibacterial activity [13-17]. In dentistry, AgNPs are added to some dental materials to enhance their antibacterial properties, which have shown promising results [18].
This study aimed to assess the effect of the addition of AgNPs to MTA (for use as an orifice plug) on coronal microleakage using the bacterial leakage model. The null hypothesis was that no significant difference exists between Ag-MTA and MTA for the prevention of microbial microleakage when used as an orifice plug.
2. METHODS AND MATERIALS
The study protocol was approved by the Ethics Research Committee of Tehran University of Medical Sciences (approval no: IR.TUMS.REC.1394.1075). 40 sound single-canal, single-rooted human teeth freshly extracted for orthodontic reasons or periodontal disease with straight roots, mature apices, and no cracks or resorption were chosen for this study. All teeth were cleaned from soft tissue residues and calculus using a curette (Hu-Friedy, Chicago, USA). Radiographs were taken to ensure that the teeth had a single canal, and the roots were inspected under a loupe (Riester, Jungingen, Germany) with × 2 magnification to ensure the absence of cracks. The roots were cut vertical to the longitudinal axis of the tooth using a bur (Dentsply International, York, PA) underwater and air spray. The root length of all teeth was adjusted to be 16 mm.
A #15 K-file (Dentsply Maillefer, Ballaigues, Switzerland) was introduced into the root canal until its tip was visible at the apex. The working length was determined 1 millimeter short of this length. The coronal third was prepared using #1, 2, and 3 Gates Glidden drills (Dentsply International Inc, Pennsylvania, USA). Root canals were then prepared according to the working length using ProTaper rotary system (S1, S2, F1, F2, F3, F4, and F5, in an orderly manner) (Dentsply Maillefer, Ballaigues, Switzerland). RC Prep (Premier, North America, USA) was used as a lubricant, and 1 mL of 2.5% sodium hypochlorite (NaOCl) was used as irrigating solution between files. After completion of the instrumentation, the smear layer was removed via irrigation with 3 mL of 17% ethylenediaminetetraacetic acid and 5.25% NaOCl each for 5 minutes. A final rinse with 5 mL of saline was then performed. The roots were then cleaned, and the entire root surface except for the orifice and apical 2 mm of the roots was covered with two layers of nail varnish to prevent microbial contamination from the outer surface. In the negative control group, the entire root surfaces were sealed with two layers of nail varnish. All samples were sterilized through autoclave-, and the rest of the experiment was performed under a hood (Nuaire, Plymouth, MN, USA) in sterile conditions. The teeth were then randomly divided into 2 experimental (n = 15) and 1 positive and 1 negative control (n = 5) groups. All teeth except for the controls were filled with gutta-percha (Meta Biomed Co., Ltd., Cheongwon, Korea) and AH Plus sealer (Dentsply DeTrey, Konstanz, Germany) using lateral compaction technique. Then, the coronal 2 mm of the root filling in all experimental groups was removed by a heat carrier (Dentsply Maillefer, Ballaigues, Switzerland). The remaining root canal filling was horizontally condensed by a plugger (Dentsply Maillefer, Ballaigues, Switzerland). Moreover, a cotton pellet dipped in alcohol was used to remove sealer from the orifice walls.
The study groups were prepared as follows:
Group 1 (MTA): Gray ProRoot MTA (Dentsply Tulsa Dental, Johnson City, TN) was used as an orifice plug (n = 15). MTA was mixed and applied according to the manufacturer’s instructions. One gr of Gray MTA (Dentsply Tulsa Dental, Johnson City, TN) was mixed with 0.35 gr of distilled water placed in the orifice using an MTA carrier (Medesy, Manigo, Italy) and condensed by a plugger (Dentsply Maillefer, Ballaigues, Switzerland). Also, a wet cotton pellet was placed on top of it. All materials were weighted by a digital weighing machine (AND GR-200 Analytical Balance, Lab Recyclers Inc., Gaithersburg MD, USA).
Group 2 (Ag-MTA): The teeth in this group were prepared as in group 1; the only difference was that MTA liquid was mixed with a suspension of AgNPs (200 ppm, mean particle size of 20nm) (NanoSany Corporation, Mashhad, Iran) with a volume ratio of 1:1 on a vortex, and the resultant solution was then mixed with gray ProRoot MTA powder (n = 15).
Group 3: The entire root surfaces were covered with two layers of nail varnish. This group served as the negative control group (n = 5).
Group 4: Root canals were filled with a single gutta-percha cone without a sealer and no orifice plug. This group served as the positive control group (n = 5).
The teeth were then placed in boxes, coded, and incubated at 37°C and 100% humidity for 24 hours to allow setting of sealer and MTA.
2.1. Assessment of Bacterial Leakage
Microleakage was assessed by a two-chamber system according to Fathi et al. [6]. Briefly, the prepared root (cut at the cementoenamel junction) was placed in cut-end microtubes such that the coronal 2 mm of the tooth was inside the microtube and its root was out of it. The microtube was used to provide a space above the root canal orifice for saliva contamination. A hole was created in a penicillin bottle rubber stopper using an acrylic bur (Dentsply International, York, PA, USA) and handpiece. The microtubes were then inserted through the created holes, and the gaps between the tooth surface, microtube, and the rubber stopper were sealed with glue (UHU, Bühl, Germany) to prevent bacterial leakage from the outside environment to the testing environment (Fig. 1). The assemblies (microtube, tooth, and penicillin bottle rubber stopper) were sterilized through the exposure to ethylene oxide gas for 12 hours. After sterilization, sterile Brain Heart Infusion (BHI) broth (QUELAB, Montréal, Canada) was added to sterile vials in sterile conditions under a hood, and then the assemblies were placed in the vials such that the apical 2 mm of the root was placed in the BHI broth (Fig. 2). Moreover, all interfaces were sealed with glue. All samples were stored in an incubator at 37°C and 100% humidity for 5 days prior to the experiment to ensure sterilization, and it was observed that no contamination occurred. After ensuring sterilization, the human saliva was added to the samples every 3 days. All groups were monitored daily until turbidity was observed in the BHI broth and the turbidity of the BHI broth indicated microbial leakage in the liquid culture medium. All samples were monitored for 120 days as performed by Fathi et al. [6].
2.2. Assessment of Bacterial Culture
The contaminated BHI broth was then cultured to determine the bacterial species causing turbidity and identify the bacteria with the highest capability to pass through the orifice plug. By using a graded pipette, one drop (equal to 0.05 mL) of the contaminated BHI broth was inoculated on the blood agar and Eosin Methylene Blue agar culture media. For determining the type of bacteria, the formed colonies were removed by a sterile needle and cultured on differential media, including triple-sugar iron, sulfide indole motility, citrate, and urea culture media.
2.3. Statistical Analysis
The log-rank test was used to analyze the data, and
p < 0.05 was considered statistically significant.
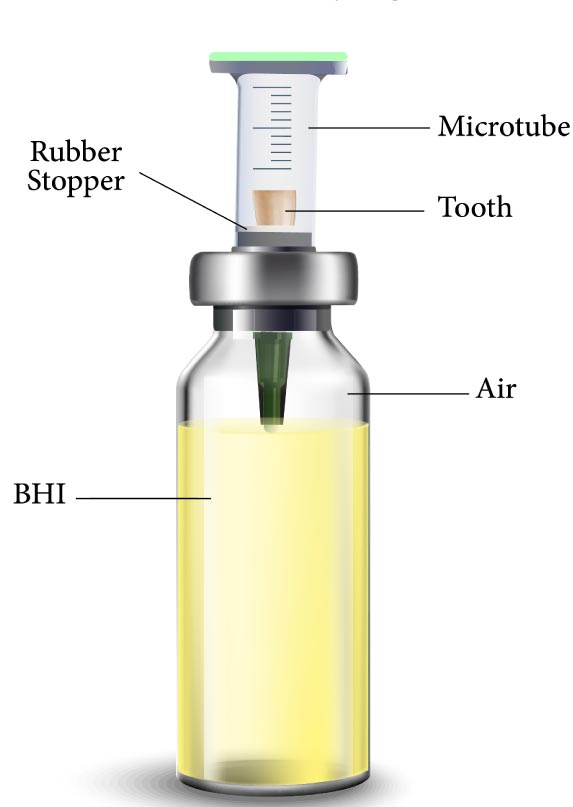
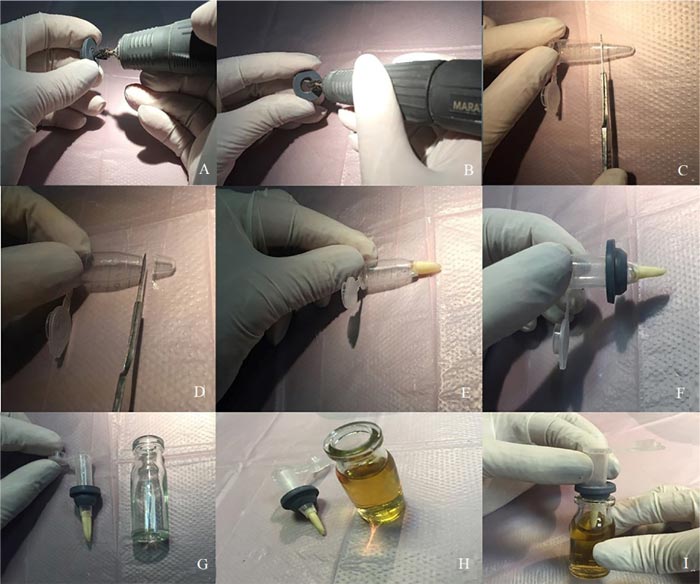
3. RESULTS
No contamination was noted throughout the experimental period in the negative control group, which indicated the effectiveness and reliability of the model employed in this study. However, contamination occurred within 5 days in the positive control group.
The mean and standard deviation of microleakage were 32.66 ± 5.74 days in the MTA group and 54.73 ± 7.58 days in the Ag-MTA group. The difference between the two experimental groups was statistically significant (p = 0.031) using the log-rank test. One-third (n = 5) of the samples in the Ag-MTA group showed no contamination by the end of the experiment, whereas, in the MTA group, all samples showed contamination by day 77.
3.1. Microbial Assessment Results
After monitoring samples for 24 hours, the culture media were evaluated by differential tables. Evaluation of the isolated bacteria revealed that bacteria from the Enterobacteriaceae family had the highest capability to pass through the orifice plug. In the MTA group, Escherichia coli and Klebsiella were the most commonly found bacteria, which passed through the orifice plug, whereas, in the Ag-MTA group, Klebsiella was the most commonly detected bacterium.
4. DISCUSSION
Bacterial leakage occurs in the absence of an ideal coronal seal [10, 19]. The elimination of bacteria from the root canal system during root canal instrumentation and the prevention of their reentry play important roles in the successful treatment of apical periodontitis [20].
Several in vitro models have been suggested to assess the root filling materials and their efficacy in providing an ideal coronal seal, such as the dye penetration method, scanning capacitance microscopy, fluid filtration technique, radioisotope electrochemical method, and bacterial microleakage model [21]. Some of the suggested models cannot ideally simulate the clinical setting. For instance, since the size of dye molecules and electrochemical radioisotopes is smaller than the actual size of bacteria, they would have faster diffusion than the bacteria, which compromises the accuracy of the obtained results. Moreover, in the dye penetration method, air bubbles may prevent dye penetration, which may also change the results [22, 23]. Oliver and Abbot reported that the dye penetration method was a poor indicator for the assessment of clinical leakage of root canal filling materials or the assessment of the efficacy of different root canal obturation techniques [23]. The assessment of coronal leakage by the bacterial leakage model is widely performed because this method can better simulate the in vivo environment and its results are more generalizable to the clinical setting [24]. Maltezos et al. assessed the bacterial leakage of root-end filling materials using Streptococcus salivarius [21]. Moreover, E. faecalis [25] have also been used in the previous studies. It has been found that saliva penetrates into the filled root canals in the clinical setting, which has a poly-microbial nature. Thus, the bacterial leakage model with the use of natural saliva better simulates the oral clinical setting and yields more reliable results [4]. Moreover, the use of only one bacterial strain cannot be a true reflection of in vivo clinical environment. A previous study showed that the assessment of microleakage in endodontically treated teeth using saliva provided more reliable results and better simulated the oral clinical setting [4]. Thus, in the current study, natural human saliva was used similar to the previous studies [26, 27].
The significance of coronal seals in the preservation of periapical health via the prevention of microleakage of bacteria and their byproducts has been well emphasized [4, 5]. Previous studies have supported the placement of a barrier over the orifice [5, 8, 10]. However, no material has been introduced to completely inhibit bacterial microleakage into the root canal system for indefinite periods of time. The use of MTA as an orifice plug has been extensively studied. Some previous studies found no difference between the efficacy of MTA, Cavit, and Tetric [5, 8], resin-modified glass ionomer and MTA [4], or Cavit TMG and EvoFlow [8] for orifice plug while some others reported that MTA orifice plug had the lowest microleakage [1]. Despite the favorable properties of MTA, such as prevention of coronal leakage, high adaptation to the canal walls, minimal cytotoxicity, and high biocompatibility, its antibacterial activity is still questionable [11].
However, studies comparing gray MTA and white MTA [4, 28, 29] and those comparing Portland cement and MTA using bacterial leakage model found no significant difference in the antibacterial seal of these materials. All these findings show that change in the amount of Al2O3, SiO2, CaO, and bismuth does not improve the antibacterial activity of MTA. Thus, we decided to enhance the antibacterial properties of MTA by the addition of AgNPs. The antimicrobial properties of silver compounds have long been recognized [30], and AgNPs show superior antibacterial properties compared with other silver salts due to their much higher surface area, which provides an effective contact with the microorganisms. The AgNPs attach to bacterial cell membranes and penetrate into the bacterial cells. The bacterial membrane contains sulfur-containing proteins, and AgNPs react not only with these proteins but also with phosphorous-containing compounds, such as DNA, and also exert antibacterial effects [31, 32]. Due to these properties, AgNPs are extensively used in contemporary dentistry [18].
She and Zhang also confirmed the antibacterial effects of AgNPs on six oral pathogens, including Streptococcus mutans, lactobacillus, Actinomyces viscosus, Candida albicans, Staphylococcus aureus, and Escherichia coli, and recommended the addition of AgNPs to dental materials wherever a strong antibacterial activity is required [33]. Casemiro et al. added AgNPs and zinc zeolite to acrylic resins and reported a significant increase in their antibacterial activity [34]. Moreover, Kawahara et al. suggested that AgNPs could serve as a carrier with strong antibacterial activity for dental materials even under anaerobic conditions, as in deep periodontal pockets [35]. Hiraishi et al. found that Ag(NH3)2F nanoparticles had antimicrobial activity against E. faecalis biofilm and claimed that silver deposition within the dentinal tubules enabled sustained release of silver ions over time [36].
The current study was the first to use AgNPs in combination with MTA for the orifice plug. The results showed that the addition of AgNPs to MTA caused a significant difference in bacterial leakage, and one-third of the samples had no bacterial leakage at 120 days. Therefore, the null hypothesis of the study was rejected. This finding confirmed the results of previous studies that reported the increased antibacterial activity of dental materials following the addition of AgNPs.
CONCLUSION
This study highlighted the potential of using AgNPs in combination with MTA for use as an orifice plug in endodontically treated teeth to prevent bacterial leakage. However, further studies are required to assess the effect of the addition of AgNPs on other properties of MTA.
ETHICS APPROVAL AND CONSENT TO PARTICIPATE
The study protocol was approved by the Ethics Research Committee of Tehran University of Medical Sciences,Iran (approval no: IR.TUMS.REC.1394.1075).
HUMAN AND ANIMAL RIGHTS
No animals were used in this research. All human research procedures followed were in accordance with the ethical standards of the committee responsible for human experimentation (institutional and national), and with the Helsinki Declaration of 1975, as revised in 2013.
CONSENT FOR PUBLICATION
Informed consent was obtained from all participants prior to the study.
AVAILABILITY OF DATA AND MATERIALS
The data sets analyzed during the current study are available from the corresponding author [F.A], on request.
FUNDING
None.
CONFLICT OF INTEREST
The authors declare no conflict of interest financial or otherwise.
ACKNOWLEDGEMENTS
The support by Tehran University of Medical Sciences, International Campus is acknowledged.