All published articles of this journal are available on ScienceDirect.
Improving Oral Surgery: A Workflow Proposal to Create Custom 3D Templates for Surgical Procedures
Abstract
Background:
Computer-guided technologies are adopted in various fields of surgery to limit invasiveness and obtain patient benefits in terms of surgery duration and post-operative course. Surgical templates realized through CAD/CAM technologies are widely diffused in implant dentistry. The aim of this work is to propose, beyond implantology, the feasibility of application of 3D printed surgical templates in oral surgery procedures requiring osteotomies (like maxillary cyst enucleation and tooth disimpaction) in order to obtain accurate surgeries, avoid anatomical damage of surrounding structures and decrease patient’s morbidity, using a simple, low-cost protocol of fabrication.
Objective:
To provide a reliable CAD-CAM workflow for the realization of surgical templates in oral surgery.
Methods:
Three clinical scenarios are described: A maxillary canine disimpaction, a mandibular cyst removal, and an orthodontic miniscrew placement. Each one was managed using custom surgical templates realized using the proposed workflow. A Stereolithography (STL) file of maxillary structures was obtained by the use of a 3D medical image processing software (Materialise Mimics 20.0) a segmentation toolbox acquiring RX volumes by Cone-Beam Computed Tomography (CBCT). Digital models of the teeth, acquired as STL files directly, are imported in the same 3D medical image processing freeware (Materialise Mimics 20.0) to merge STL files of maxillary structures and teeth. Data are transported into Blue Sky Plan 4.0 (Blue Sky Bio, LLC), a software for 3D implant guides fabrication, together with the DICOM images package of maxillary volumes to carry out the pre-surgical treatment planning. Anatomical structures at risk are identified; a contour of ideal incision shape and bone osteotomy extent is drawn. Finally, the resulting three-dimensional guide is digitally generated and the surgical guide printed. The resulting 3D template shows the following major features: teeth support, flap management and bone osteotomy design.
Results:
The proposed work-flow aided the surgeon in both pre-operative and intra-operative work phases through accurate virtual planning and the fabrication of precise surgical guides to be used in oral surgery practice. In each clinical scenario, the use of custom 3D templates allowed better control of the osteotomy planes and flap management. No adverse events occurred during both surgical and healing phases.
Conclusion:
The proposed digital workflow represents a reliable and straightforward way to produce a surgical guide for oral surgery procedures. These templates represent a versatile tool in maxillary cyst enucleations, tooth disimpaction, and other surgical procedures, increasing accuracy, minimizing surgical complications, and decreasing patient’s morbidity.
1. INTRODUCTION
Computer-Aided technologies (CAx) [1] are widely adopted in various fields of medicine, providing different tools in the design, analysis, and manufacture of several products intended for both surgical and non-surgical procedures, thanks to their accuracy [2-5].
In dentistry, 3-D printed templates, developed through Computer-Aided Design/Computer-Aided Manufacturing (CAD/CAM) workflows, have been successfully used in orthodontics, endodontics, prosthodontics, orthognathic surgery, oral surgery, and most of all, implant surgery [6-11].
Surgical templates represent a link between preliminary virtual planning and the actual surgical treatment, simplifying the approach to the procedure and enabling surgeons to carry it out in a more predictable, more conservative way, thus leading to better outcomes [12-14].
Therefore, the typical surgical paradigm, whereby the view of the surgical area achieved through tissue exposure is strictly needed, becomes progressively less mandatory [15].
In recent years, prosthetically-driven implant surgery has been emphasized for its different upsides, such as long-term stability of peri-implant hard and soft tissues, favorable esthetic outcomes, and better prosthetic design, avoiding non-retrievable cemented restorations in favor of screw-retained solutions [16-19]. Prosthetically-driven implant positioning has to account for different aspects, such as the desired inter-implant distance, tooth-to-implant distance and implant depth, [15, 20, 21] and, consequently, requires an accurate preliminary virtual planning, further highlighting the pivotal role of computer-guided surgical protocols.
According to the literature, two protocols exist for guided implant surgery: Computer-guided surgery, or static protocol, and computer-navigated surgery, or dynamic protocol [22].
According to the static protocol, the implant position is transferred from virtual planning based on computerized tomographic data straight to a surgical guide, which does not allow intra-operative modifications of the fixture position [15, 22-24].
Dynamic surgery utilizes surgical navigation systems to reproduce the virtual implant position, additionally allowing for intra-operative modifications of the fixture position. [15, 23] Computer-navigated surgery is beyond the scope of this paper and will not, therefore be further described.
Static systems tend to be more accurate than their dynamic counterpart [24], although an exact comparison is not possible since most studies on computer-assisted surgical protocols are preclinical, thus allowing more precise measurements and excluding intraoperative variables, such as patient’s movements, the presence of blood/saliva, and others [23]. In consideration of their supporting structures, three types of In consideration of their supporting structures, three types of surgical guides can be distinguished: tooth-supported templates, in which the surgical guide is placed on the remaining teeth; mucosa-supported templates, placed on top of the mucosa; bone-supported templates, positioned on the bone after mucoperiosteal flap elevation.
According to the literature, tooth-supported surgical guides are the most reliable, [25-27] while bone-supported ones show the highest inaccuracy [15, 24]. Different methods have been introduced to produce surgical guide from virtual planning data, such as the conversion of radiographic templates to surgical templates through mechanical positioning devices or drilling machines and a computer transformation algorithm, [28, 29] or CAD-CAM workflows to generate stereolithographic templates (rapid prototyping) [30].
Digital model acquisition represents the first step toward CAD/CAM manufacturing [31].
An alternative to conventional plaster casts, digital models show several benefits, such as improvements in efficiency and precision of treatment planning, [31] facilitated data storage, reproducibility, [32-34] better treatment documentation, and better communication with either patient, laboratory, or other professionals [32, 35].
They can be produced directly, via intraoral scanning, or indirectly, using extraoral scanners.
This work aims to propose, beyond implantology, the application of 3D printed templates in oral surgery procedures requiring osteotomies and/ or minimally invasive flaps. Three clinical scenarios are presented to describe the different steps of the workflow used to realize customized surgical templates for oral surgery procedures.
2. METHODS
The proposed workflow for surgical templates production is composed of different steps, as described below:
1 DICOM CBCT scan file importation and 3-dimensional rendering in a 3D-imaging software;
2 CBCT 3-D rendering alignment to the digital model STL;
3 Render/STL files merging;
4 Merged file exportation in a virtual planning software and alignment to CBCT’s DICOM data;
5 Surgical template realization;
6 Surgical procedure.
The patient undergoes a maxillary CBCT scan with a Field of View (FOV) chosen in accord with anatomic and surgical needs. Additionally, a direct or indirect digital impression of dental arches is acquired. In this study, a plaster model from an alginate impression was digitalized via extraoral scanning.
After collection, radiographic data were imported in a dedicated software. For our surgical planning protocol, the software of choice was Mimics (Materialise NV, Leuven, Belgium), a commonly used commercial package designed for medical image processing [36]. The MIMICS software converts DICOM files into a proprietary format, presenting the data in axial, coronal, sagittal, and 3-dimensional recons tructions [37].
Using its “segmentation mode”, a 3-dimensional render of the jaws (either one or both) is then produced. To further improve the render quality, it is possible to use the “Smooth” and “Reduce” tools.
The STL model file is then imported and superimposed to the render using the “Alignment” tool. Once paired and correctly aligned, the CBCT render is selected, and the portion including the teeth’s surfaces is cut and deleted. This way it is possible to discard low-quality tooth rendition of dental surfaces generated from the CBCT DICOM file in favor of a more detailed and better defined one, acquired from the digital model STL and to overcome the impression anatomical limits.
The two files are then merged, creating a single 3-dimensional reconstruction containing info from both the radiographical exam (bone structures) and the digital model (teeth surfaces) (Fig. 1).
Following this step, the merged file is exported in a virtual planning software. In the proposed workflow, the Authors used the freeware Blue Sky Plan® (Blue Sky Bio LLC, Grayslake, IL), a medical device that allows the practitioner to view and reformat images acquired from computerized tomography and digital scanning. To verify the correctness of the DICOM render/STL alignment, the merged file is paired with the DICOM file obtained from the CBCT scan and aligned. With correct execution, the files should overlap without significant discrepancies (Fig. 2).
Surgical guide production is then performed in the Blue Sky Plan, according to the software’s routine (Fig. 3).
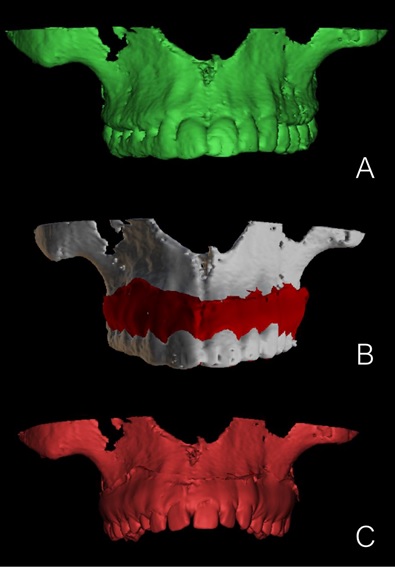
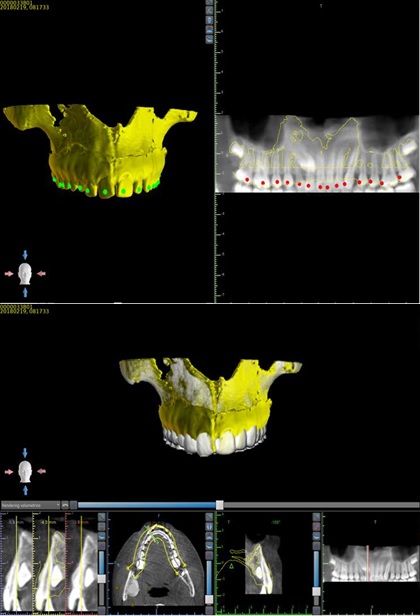
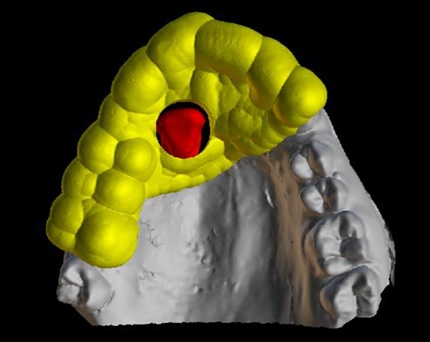
Guide contours corresponding to osteotomy lines are drawn using software dedicated tools, with custom fixtures as reference pins, whenever a bone trap is requested.
Finally, the resulting STL file is sent to the laboratory for guide realization. In the case series described below, the surgical templates were printed using a Form 2 SLA Desktop 3D printer (FormLabs Inc., Somerville, MA, USA) and resin drying was accelerated using BB Cure Dental UV chamber (Meccatronicore, Trento, Italy).
In order to provide better comprehension of this digital workflow, three case reports are presented below. All the three patients were treated by the Oral Surgery Unit of the Department of Biomedical and Dental Sciences and Morphofunctional Imaging of the University of Messina. A single oral surgeon performed all surgeries.
3. CASE REPORTS
3.1. Clinical Scenario A
A 13-year-old male, in good health, was referred by the Orthodontics Unit complaining about the presence of the upper right primary canine, that was not correctly exfoliated and was still retained and was firm. However, the patient did not like the discrepancies between the upper left canine and the right one, that appeared smaller and greyish. A final complaint regarded the upper incisors misalignment, which he perceived as highly unaesthetic. No significant medical history was found, nor any extraoral abnormality detected. There was no history of trauma or surgical procedure related to the head or face. The Orthopantomogram (OPT) confirmed the upper right canine impaction, thus a Cone-Beam Computed Tomography (CBCT) was prescribed to assess the tridimensional characteristics of the canine impaction. The CBCT highlighted the mesioangular endosteal impaction of the canine, positioned with its crown adjacent to the lateral incisor root. In consideration of this clinical scenario, an orthodontic-surgical approach was chosen to comply with the patient’s functional and aesthetic needs. No antibiotic prophylaxis was administered prior to the surgical procedure. The surgical template was therefore produced with a round-shaped window to identify the profile of the impacted crown. The tooth-supported template extended from the first right molar to the upper left canine, to provide sufficient stability and structural integrity. Following local anesthesia with mepivacaine hydrochloride 2% with adrenaline 1:100,000, soft tissue removal was performed by the use of a diode laser (Sirolaser Blue (Sirona Dental Systems GmbH Bensheim, Germany)). A piezosurgical device (Surgysonic II, Esacrom, Imola, Italy) was used to erode the thin palatal bone shield and expose the crown of the impacted tooth Fig. (4). The primary upper right canine was then removed. The access to the impacted teeth was maintained during post-surgical healing to promote passive tooth eruption using a periodontal dressing. Dietary and oral hygiene instructions were given, and soft tissue healing was monitored over the following 3 weeks. No adverse events occurred during this time.
The delayed placement of an auxiliary attachment was performed after ten days Fig. (5).
3.2. Clinical Scenario B
A 32-year-old female, non-smoker and affected by ulcerative colitis, came to observation referring paresthesia of the lower incisors and chin area. Orthopantomography revealed the presence of a radiolucent area in the interforaminal region, diagnosed as an odontogenic cyst. A dental CT exam was then prescribed to plan cyst removal. The lesion extended from the apex of the right lower lateral incisor to the left lower lateral incisor, with no sign of dehiscence of both the buccal and lingual cortical plates.
According to the workflow steps, a plaster model was poured, digitalized, and then combined with CT 3-dimensional render to ultimately realize a surgical guide with a rectangular-shaped window Fig. (6).
Once infiltration alveolar nerve block was performed, a full-thickness paramarginal mucoperiosteal flap was reflected, and the surgical guide positioned. The osteotomy was then performed using Esacrom Surgysonic II and its ES007T tip (thickness: 0.6mm, operative length: 12mm, length by the angle: 14mm) and the bone trap removed to gain access to the lesion Fig. (7). The cyst was enucleated using the ES003AT (ø: 4.5mm, operative length: 7.4mm, length by the angle: 11mm) and ES003BT (ø: 4.5mm, operative length: 7.4mm, length by the angle: 13mm) for the initial elevation of the cystic membrane, that was completed by the use of Prichard periosteal elevator. After enucleation, two small holes were drilled in both the removed bone trap and the vestibular cortical plate. The trap was then put back in place and kept in position using resorbable sutures. Wound closure was obtained through simple interrupted stitches with a 5.0 size nylon suture (Ethilon1, Ethicon Inc., Somerville, NJ) removed seven days later.
Clinical and radiological follow-up at one year showed no sign of relapse.
3.3. Clinical Scenario C
An 18-year-old male, in good health, was referred by the Orthodontics Unit for a palatal expansion treatment. The intraoral and extraoral examination highlighted a Class III malocclusion, with a concave profile and a bilateral buccal crossbite. Remarkable discrepancy between the retruded maxilla and the protruded mandible was associated with constriction of the maxilla.
Treatment of choice was the Miniscrew-assisted Rapid Palatal Expansion (MARPE), which consists of the placement of two orthodontic screws in the palatal processes of maxillary bone in order to obtain skeletal anchorage of an expansion device. The planned position of the screw is crucial for correct appliance of forces able to open the median palatal suture.
Therefore, a dental CT was required to correctly choose the insertion site. After data processing and surgical guide realization Fig. (8), two orthodontics screws (OrthoEasy Pal, 8mm x 1.7mm, Forestadent) were placed in the rugae region Fig. (9). and the expander then placed and cemented Fig. (10). The miniscrews showed no noticeable change in position throughout the expansion phase. Expansion was terminated at 6 weeks, with a 9.3 mm increase in intermolar width. The expander was kept in position for an additional 3 months to allow bone formation in the separated palatal suture, thus stabilizing the result. No sign of soft tissue inflammation around the miniscrews was detected during the treatment.
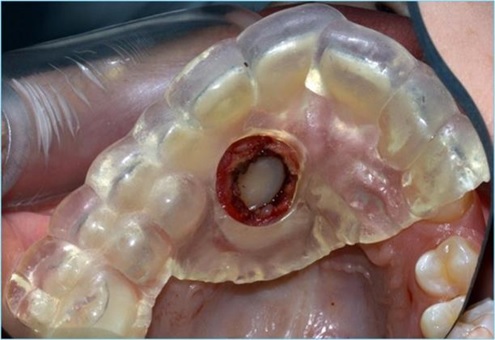
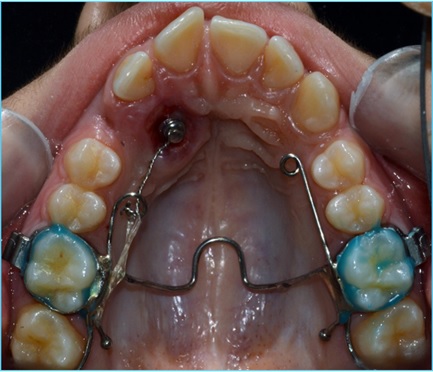
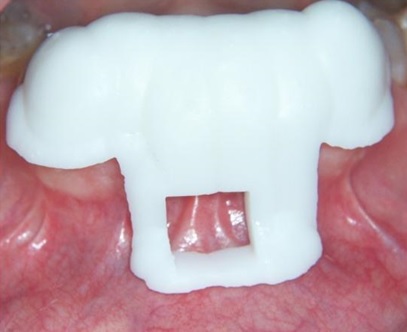
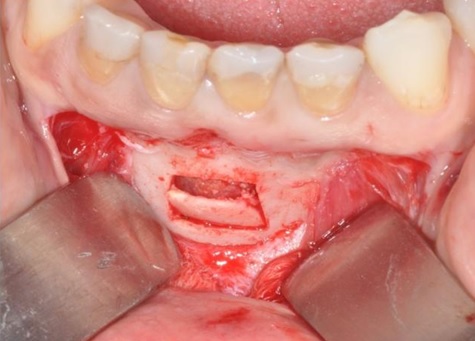
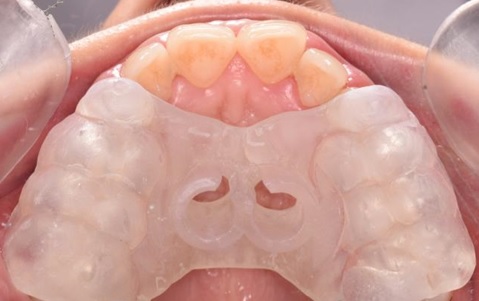
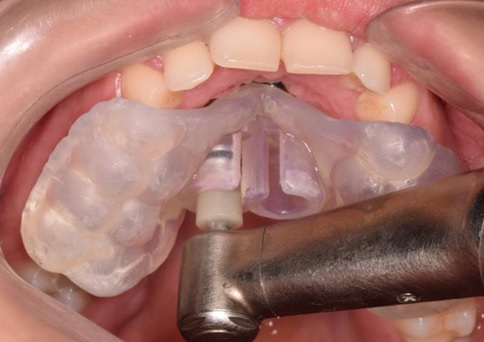
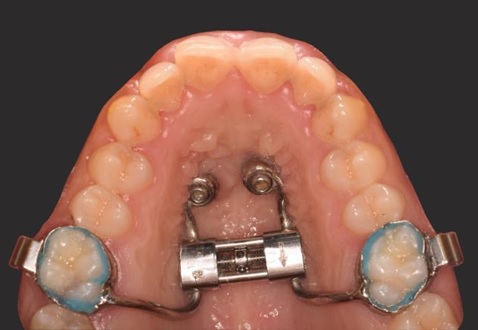
CONCLUSION
The objective of the study was to present a specific workflow to be applied by Oral Surgery Units when planning surgical oral treatment. In oral surgery, the preparation of the osteotomy window is crucial, size, design, and position may affect the intra- and post-surgical complication rates. The use of 3D system techniques surgery can provide useful intra-operative guidance and may help to further increase the accuracy of the surgery and patient safety, reducing the risk of surgical complication related to incision size and extent of the osteotomy. This paper shows the successful application of a simple, low-cost digital workflow to different clinical scenarios, like tooth disimpaction, cyst removal, and miniscrew insertion. Clinical trials are needed to better evaluate the efficacy of the proposed protocol.
ETHICS APPROVAL AND CONSENT TO PARTICIPATE
Not applicable.
HUMAN AND ANIMAL RIGHTS
No animals/humans were used in the study that are the basis of this research.
CONSENT FOR PUBLICATION
Not applicable.
STANDARD FOR REPORTING
Care guidelines and methodology were followed to conduct the study.
FUNDING
None.
CONFLICT OF INTEREST STATEMENT
The authors declare no conflict of interest, financial or otherwise.
ACKNOWLEDGEMENTS
Declared none.