All published articles of this journal are available on ScienceDirect.
Effect of Angle and Type of Customized Abutment (Castable & Cast-to) on Torque Loss and Fracture Resistance After Cyclic Loading
Abstract
Background:
Implant placement with more than 25° angle and use of customized abutments are still challenging in implant dentistry. Also, casting is still the most commonly used method for fabrication of customized abutments.
Objective:
This study evaluated the effect of angulation and type of abutment (castable and cast-to) on torque loss and fracture resistance after cyclic loading.
Methods:
Two implants were mounted with 0 and 30° angle on a gypsum model. Castable and Cast-to abutments were casted by cobalt-chromium alloy on each implant (10 samples in 4 groups). Rotational freedom was measured by a video-measuring microscope. The reverse torque values before and after cyclic loading (500,000 cycles) were measured by a digital torque-meter. Abutments were subjected to fracture resistance test in a universal testing machine. Data were analyzed using the Kruskal Wallis, two-way ANOVA and repeated measures tests.
Results:
Difference between castable and cast-to abutments regarding rotational freedom was not significant. Torque loss in castable abutments was significantly greater than cast-to abutments before and after cyclic loading (P < 0.05). The effect of abutment angle on torque loss before and after cyclic loading was not significant.
Conclusion:
Irrespective of the abutment angle, torque loss was significantly higher in castable groups. Considering the high fracture resistance, abutment fractures were not clinically an issue.
1. INTRODUCTION
Ideally, implant body must be placed perpendicular to the curves of Wilson and Spee that compressive loads apply along the longitudinal axis of implant. Axial parallelism minimizes the stress applied to the entire implant system and
decreases the related biological and mechanical complications (screw loosening and abutment fracture). Nonetheless, some anatomical variations such as bony concavities at the facial surface of maxilla or lingual surface of mandible may force the surgeon to place the implant in an angulated fashion. Implant angulation must be corrected by prosthesis via the placement of angulated abutments in order to maintain the integrity of dental arch and the efficiency of mastication [1].
Angulation more than 25° in implant placement and use of customized abutments are still challenging in implant dentistry. Despite the advances in computer-aided design/computer aided manufacturing systems, casting is still a common method of fabrication of these abutments. Cast abutments can be used in cases with limited inter-occlusal distance and improper implant angle to 30°. Moreover, these abutments are cost-effective [2]. Cast abutments are available in two forms of cast-to and castable. The main advantage of cast-to abutments is the precise fit of the connection. However, in castable type the connection area is also cast and therefore, has lower precision [3].
Several studies have assessed the effect of rotational freedom of abutments on implants, indicating that movement of the abutment on implant can cause screw loosening [4]. Binon et al., [5], indicated that increasing the rotational freedom from 2 to 3° caused 26% reduction of the cycle in cyclic loading prior to screw loosening. Rotational freedom of abutment on implant should not be more than 5° and rotational freedom less than 2° would result in most stable screw connection [6]. Moreover, it has been shown that complete elimination of rotational freedom (0°) increases the resistance to screw loosening in five million cyclic loads, and rotational freedom greater than 5° would decrease the number of cycles before screw loosening by 63% [5].
Screw loosening primarily occurs due to misfit of implant components, insufficient tightening, application of excessive loads to the complex and improper screw design [7, 8]. Application of high bending forces to the screw, settling effect and decreased preload are among other causes of screw loosening [9, 10].
Fatigue fracture is among the main factors responsible for structural fracture of implants. Thus, cyclic loading can better simulate the clinical setting in vitro [11, 12].
By increasing the use of base metal alloys, there is an obvious need to assess the rotational freedom, screw loosening and fracture resistance of these abutments especially the angulated types. Therefore, this study aimed to assess the effect of angle and type of customized abutments (castable and cast-to) on torque loss and fracture resistance following cyclic loading.
2. MATERIALS AND METHODS
2.1. Sample Size Calculation
Using Adatia et al., study [13] and PASS 11 software, by one-way ANOVA power analysis option and considering α = 0.05, β = 0.2, SD = 245, effect size = 0.5 the sample size calculated 10 in each group (4 groups: angled castable, angled cast-to, straight castable, straight cast-to).
2.2. Mounting of Analogs on the Cast
In this experimental in vitro study, a mandibular gypsum cast was used for mounting two fixture analogs (DAN38, Implantium, Dentium, South Korea) in tooth #20 and #29.
Using a surveyor, analogs were placed with 30° lingual angle (the worst case) relative to the longitudinal axis of the adjacent tooth in one side of the cast and parallel to the longitudinal axis of the adjacent tooth in the other side such that 1 mm of the analog margin was out of the gypsum. Next, metal casting abutments (Implantium, Dentium, South Korea) were used as cast-to and plastic temporary abutments (Implantium, Dentium, South Korea) were used as castable abutments for the fabrication of customized abutments. Ten cast-to and 10 castable abutments were fabricated for each implant (a total of 40 customized abutments).
2.3. Abutment Preparation
Abutments had 4.5 mm diameter, 4 mm height and 1 mm gingival height. Hex abutments were used. No taper was considered for the abutments.
A jig was used for the fabrication of angulated abutments to control the buccal and lingual contour. This jig was placed on the occlusal surface of the adjacent teeth for further stability.
Abutments were waxed and mounted in phosphate bonded carbon-free investment gypsum (Polivest, Polident, Brazil). Casting with cobalt-chromium (Co-Cr) alloy (Wirobond C, Bego, Germany) was performed according to the manufacturer’s instructions. After casting, no change was made to the implant-abutment connection site.
2.4. Mounting of Implants in Acrylic Resin
Implants with 12 mm in height and 4 mm in diameter (Implantium, Dentium, South Korea) were thoroughly wrapped in thin aluminum foil (that did not interfere with load transfer) in order to prevent penetration of acrylic resin into the implant threads. Using a surveyor, angulated implants were mounted with 30° angle and straight implants were mounted vertically relative to the vertical line such that 1 mm of the fixture margin was out of the acrylic resin (Acropars™, Marlic Co., Tehran, Iran).
2.5. Assessment of Rotational Freedom
A Video Measuring Microscope (VMM) (MV300, Starrett, England) was used for measurement of rotational freedom. The software of the VMM determined several points on the periphery of the implant platform and implant center. Next, the samples were placed on implant without screwing and their complete seating on implant connection was ensured. Each abutment was then rotated in clockwise fashion until stopped. This process was scanned by VMM. Next, the abutment was rotated in anti-clockwise fashion and scanned again. The angle between the two images indicated the rotational freedom of each sample and reported in degree (Fig. 1).
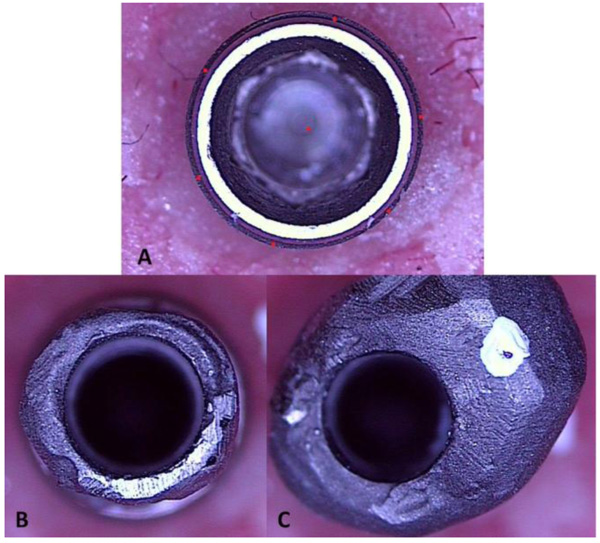
2.6. Measurement of Reverse Torque Value (RTV)
Mounted implants were stabilized by a mounting jig to stand without rotation during torquing and detorquing. The abutment screws were then torqued to 30 Ncm suggested by the manufacturer on respective implants. Electronic torque meter (Lutron Electronic; Lutron Electronic Enterprise CO, Taiwan) used for this purpose was calibrated before the test. After 10 minutes, the samples were retorqued and then detorqued after 10 minutes. The detorque value of each sample was recorded. Torque reduction for each sample was reported by the percentage of torque loss relative to the tightening torque.
2.7. Cyclic Loading
Abutments were tightened on their respective implants by applying 30 Ncm torque. The samples were then retorqued with the same value after 10 minutes. Then, they were subjected to cyclic loading by 500,000 cycles with 1 Hz frequency under 75 N load. This value corresponded to two years of clinical service [14]. The final detorque value after loading of each sample was measured by a digital torque meter and recorded. Torque reduction for each sample was reported by the percentage of torque loss relative to the tightening torque.
2.8. Fracture Resistance Test
A straight stainless steel jig was designed and fabricated for vertical mounting of straight (castable and cast-to) abutments. An angulated stainless steel jig was designed and fabricated for mounting of angulated (castable and cast-to) abutments with 30° angle. Implants were completely fixed on the jigs and 1 mm of their margin was placed outside of the jig margin. When implants were placed on the jig, restorations were tightened on implants with 30 Ncm torque and were then retorqued after 10 minutes. Load was applied using a universal testing machine (Zwick Roell, Ulm, Germany) at a crosshead speed of 1 mm/min, and the applied load increased until deformation or fracture occurred. Simultaneous with load application, the monitor of the machine draws the fracture resistance graph.
Data were analyzed using SPSS 21 and the Kruskal Wallis, two-way ANOVA and repeated measures tests (P-value = 0.05).
3. RESULTS
Table 1 shows the maximum, minimum, mean and standard deviation of rotational freedom obtained by VMM in degree separately for each group. According to the results of the Kruskal Wallis test, due to high dispersion in castable groups, no significant difference was noted between castable and cast-to groups in terms of rotational freedom. Of 20 samples in castable groups, 10 had zero-degree rotational freedom while zero-degree rotational freedom was not seen in cast-to group.
Groups | Mean | Std. Deviation | Min. | Max. |
---|---|---|---|---|
Straight Castable | 66.1 | 73.48 | 0 | 181 |
Straight Cast-to | 150.5 | 38.32 | 78 | 213 |
Angled Castable | 84 | 92.86 | 0 | 222 |
Angled Cast-to | 155.9 | 31.58 | 101 | 220 |
Table 2 shows the maximum, minimum, mean and standard deviation of percentage of torque loss before (primary) and after (secondary) cyclic loading compared to the tightening torque applied (30 Ncm) separately for each group (groups: Angled castable, angled cast-to, straight castable, straight cast-to).
Abutment Angle | Abutment Casting Method | Percent of Torque Loss | Descriptive | |||
---|---|---|---|---|---|---|
Mean | SD | Min | Max | |||
Angled | Castable | Primary | 36.67% | 9.03% | 26.67% | 50.00% |
Secondary | 90.67% | 3.44% | 83.33% | 93.33% | ||
Cast-to | Primary | 20.67% | 4.39% | 13.33% | 26.67% | |
Secondary | 81.67% | 5.27% | 73.33% | 90.00% | ||
Straight | Castable | Primary | 30.67% | 11.74% | 13.33% | 46.67% |
Secondary | 88.00% | 4.22% | 80.00% | 93.33% | ||
Cast-to | Primary | 20.33% | 7.77% | 6.67% | 33.33% | |
Secondary | 82.00% | 6.52% | 70.00% | 90.00% |
Two-way ANOVA was applied to assess the effect of angle of abutments and type of casting on the percentage of torque loss and indicated that type of casting significantly affected the percentage of torque loss before cyclic loading. The cast-to groups (straight and angled) lost significantly lower percentage of torque before and after cyclic loading (P < 0.05). It also showed that angle of abutments had no significant effect on the percentage of torque loss before and after cyclic loading (Table 3).
Repeated measures ANOVA demonstrated that cyclic loading significantly increased the percentage of torque loss and this occurred irrespective of the type of casting (P = 0.08) and angle (P = 0.53).
The results of fracture resistance test showed that of the four studied groups, 5 samples in the angled castable group experienced fracture in response to static load application and all these fractures occurred at the site of abutment screw. Fractures occurred in response to the application of 1500 to 4500 N load. The remaining samples only experienced deformation and did not break even in response to the application of maximum load (6000 N).
After Cyclic Loading | Before Cyclic Loading | Variable |
---|---|---|
.465 | .254 | Angle |
<0.001* | <0.001* | Casting |
.349 | .307 | Angle*Casting |
4. DISCUSSION
Many manufacturers suggest using customized cast abutments as an alternative to prefabricated abutments for prosthetic rehabilitation of unconventional angulation of implants. Use of Cr-containing alloys such as Co-Cr and Ni-Cr has greatly increased due to their cost-effectiveness [15]. Evidence shows that defects in base metal restorations are mainly due to inaccurate casting rather than the suboptimal properties of the alloys [16]. The current study aimed to assess and compare the torque loss and fracture resistance of customized cast abutments after cyclic loading in two groups of straight and angulated abutments. Metal casting abutments with machined connection and plastic temporary abutments with castable connection were used in the current study. Regarding cyclic loading, it has been claimed that crown fabrication on the abutments for the conduction of this test cannot show the actual effect of aging on the abutment because the materials used for crown fabrication are mainly subjected to loads and serve as a protective shield [14, 17, 18]. In present study crown was not fabricated.
The results showed that in terms of rotational freedom between abutment and implant, all the values recorded by the VMM in castable and cast-to groups were within the acceptable threshold (5°) [8]. An interesting finding was that in 20 samples in castable groups, a high dispersion in rotational freedom was noted compared to cast-to groups and 10 samples had zero degree of freedom, which may be due to their casting process causing greater irregularities and dentations on the connection surface of these abutments compared to the machined connection, causing friction and resistance against the rotation of abutments before loading. Byrne et al., [19] reported less screw engagement in cast abutments compared to non-cast abutments. They added that misfit and rotational freedom at the abutment-implant connection can cause abutment screw loosening after loading.
By measuring the RTV, the degree of reduction in preload of different abutments can be compared [20]. To prevent preload reduction in the clinical setting, it has been suggested to retorque the abutment in the same magnitude of the tightening torque after 10 minutes [21]. The results of this study showed that the detorque value was less than the tightening torque value, which was in line with the results of the previous studies [22, 23]. Reduction in the RTV compared to the tightening torque is a result of embedment relaxation [24]. When abutment screw is subjected to torque application, micromovements between the screw and the internal surface of implant occur and the irregularities on the screw surface are flattened [25]. Such a wear between the surfaces in contact with each other approximates the metal surfaces. This phenomenon, known as settling, decreases the preload by 2-10% [24, 26]. In the current study, 20-36% reduction in the preload occurred (before cyclic loading). Also, the percentage of primary torque loss in cast-to groups was less than that in castable groups. It means that this type of abutment maintains higher tightening torque before loading. When the tightening torque is applied, some of this energy is used to smoothen the micro-porosities of casting process; instead of causing the clamping preload. Consequently, the screw loses part of its torque [27].
Height and type of connection are important factors in the percentage of torque loss. Evidence shows that longer implant-abutment connection can decrease the load applied to the internal wall of connection and screw and distribute forces more uniformly [28]. The connection height (1.2 mm) was the same in all abutments used in our study and therefore, requires no discussion. A previous study showed that compared to external connections, preload causes greater connection stability in internal connections due to the wedging effect as the result of abutment sinking [20]. Moreover, internal conical connection type was used in all groups in the present study; thus, comparison of connection type is not an issue either.
The results of this study also indicated that after cyclic loading, irrespective of the abutment angle, the percentage of torque loss in cast-to groups was less than that in castable groups. The reported difference may be due to greater misfit of castable abutment. It may be concluded that the same surface irregularities of the cast connections that caused high dispersion in the results of rotational freedom of this type of connection before cyclic loading are smoothened by cyclic loading and create greater misfit between connection components.
The abutment angle had no significant effect on the percentage of torque loss (P < 0.001); this finding was in contrast to the findings of some previous studies [29, 30]. This difference is probably attributed to the difference in the type of alloy used in the present study since no previous study has used Co-Cr alloy. It seems that casting with Co-Cr alloy causes some misfit and before the angle takes effect, loosening occurs at the connection site because of flattening of irregularities.
Delben et al., [31] reported that the RTV in Gold UCLA prefabricated abutments decreased by 68% of the initial insertion torque after mechanical cycling and this was 66% in cast UCLA abutments. Current study was comparable to that of Delben et al., [31]. However, the percentage of torque reduction after cyclic loading in current study (up to 93% in the castable and 90% in cast-to groups) was much greater than the values reported in Delben’s study [31]. This difference could be due to different implant systems, the fabrication and casting process, type of alloy and the magnitude and condition of load applied.
A systematic review regarding the success and complications of dental implant systems reported that abutment and screw fracture occurs in 1.5% of abutments at the five-year follow-up and 2.5% at the 10-year follow-up [32]. Fracture strength of different abutments was evaluated in a previous study; the results showed that the mean fracture strength was 508 ± 334 N for the prefabricated titanium and 698 ± 492 N for the zirconia abutments [17]. Moreover, previous studies showed that angulation of implant and cyclic loading with over 106 cycles can significantly decrease the fracture strength of this type of abutment [17, 30]. In the current study, angled groups (compared to the straight groups) and castable groups (compared to cast-to groups) were expected to have lower fracture resistance and higher frequency of fracture while only five abutments in angled castable group underwent fracture, which was in accord with the previous hypothesis. However, no significant difference was noted among the four groups because these fractures occurred in loads (1500-4500 N) much higher than the values reported in previous studies and the maximum masticatory forces reported in humans. Moreover, fracture in all five samples occurred at the site of screw. No fracture occurred in the remaining samples in response to loads as high as 6000 N and only deformation occurred in them.
According to the fracture resistance values obtained in present study, it may be concluded that castable and cast-to angulated and straight abutments are suitable for rehabilitation of posterior regions of the mouth and the main concern regarding their selection is the percentage of torque loss following cyclic loading especially in the castable groups.
CONCLUSION
Within the limitations of this study, the following conclusions were obtained:
- Rotational freedom of abutments on the implants in cast-to groups had less dispersion than that in castable groups and was less than 5° in all groups.
- Torque loss before and after cyclic loading, irrespective of the angle, was significantly higher in castable groups compared to that in cast-to groups.
- Only the abutments in angled castable group underwent fracture; however, considering their high fracture resistance, these fractures were not clinically significant.
ETHICS APPROVAL AND CONSENT TO PARTICIPATE
Not applicable.
HUMAN AND ANIMAL RIGHTS
No animals/humans were used for studies that are the basis of this research.
CONSENT FOR PUBLICATION
Not applicable
CONFLICT OF INTEREST
The authors declare no conflict of interest, financial or otherwise.
ACKNOWLEDGEMENTS
This study was supported by Dental Research Center Dentistry Research Institute of Tehran University of Medical Sciences (Grant No: 33239-70-02-95).