All published articles of this journal are available on ScienceDirect.
The Influence of Cold Atmospheric Plasma Irradiation on the Adhesive Bond Strength in Non-Demineralized and Demineralized Human Dentin: An In Vitro Study
Abstract
Purpose:
While aiming at the use of Cold Atmospheric Plasmas (CAPs) in restorative dentistry, the present study intended to assess if plasma irradiation increases the Tensile Bond Strength (TBS) in non-demineralized and demineralized dentin.
Materials and Methods:
Forty-eight human dentin samples were assigned to three different treatment modalities: I: Plasma jet irradiation (CAP I); II: Dielectric barrier discharge plasma treatment (CAP II); and III: No plasma (control). In each group, half of the specimens had previously been demineralized. A fourth generation of adhesive and dental composite was applied to all of the samples. The testing of the TBS was performed after artificial aging.
Results:
In the non-demineralized dentin, the mean TBS values were significantly higher after using CAP II (16.95 MPa) than in the control samples (4.2 MPa; p = 0.001). Significantly higher TBS values were also obtained after irradiating the demineralized dentin with CAP I and CAP II (11.68 and 4.6 MPa) when compared to the control samples (0 MPa; p = 0.003 and 0.038). The differences between both of the plasma sources were only slightly significant (p = 0.05).
Conclusion:
CAPs can potentially enhance the adhesive/dentin interfacial bonding strength, whereby the underlying effects seem to depend on the type of plasma source and the degree of dentinal (de-) mineralization. In the non-demineralized dentin, after a complete caries excavation, dielectric barrier discharge devices might be favorable over the plasma jets, in order to improve the adhesive/dentin interfacial bonding. In contrast, the plasma jets could be more effective in the demineralized dentin after an incomplete caries excavation.
1. INTRODUCTION
The past several decades have revealed vast technological advances in adhesive dentistry. At the resin-enamel interface, microleakage and a loss of retention no longer occur because of acid etching. But there are some problems at the resin-dentin interface in dental adhesion that still have to be solved. The bonding in dentin is sensitive to techniques and materials, because other than enamel, dentin is very heterogeneous in itself [1] and the high water content interferes with the bonding. Furthermore, a smear layer adheres to the dentin after the cavity’s preparation [2]. In addition, the wettability of the substrate surface determines the penetration of the adhesives [3]. A bonding agent has to perform hybridization, and therefore, it has to contain both the hydrophilic parts in order to bond with the enamel/dentin and the hydrophobic parts in order to bond with the resin [4]. The hydrophilic parts often times interfere with the hydrophobic photoinitiators resulting in lack of polymerization [5].
After the treatment of deep carious lesions, patients often suffer from dentinal hypersensitivities that can result in irreversible pulpitis or necrosis making a root canal treatment necessary. Micro-leakage or nano-leakage, as a result of insufficient adhesive bonding, leads to secondary caries, again resulting in endodontic interventions. In order to avoid a root canal treatment, it has been proposed to leave the carious tissue alone in deep lesions. In these particular areas of demineralized dentin, the adhesive bonding strength is lower when compared with the non-demineralized dentin.
Cold Atmospheric Plasma (CAP), as the fourth state of matter, is known for its antibacterial effects [6-13], but also for its surface-modification effects [14-16].
On dental substrates, such as enamel, dentin, and composites, hydrophilic surfaces can be achieved without any morphological changes to the surfaces [17-19]. Some studies have also shown that the Micro Tensile Bond Strength (µTBS) of various adhesives to dentin could be improved and increased [20]. It has been shown that an adhesive penetration into demineralized dentin collagen is also improved [21]. CAP is generated by either a Dielectric Barrier Discharge (DBD) or by plasma jets with the help of an inert gas.
The aim of the present study has been to investigate the influences of two different CAP sources on the strength of adhesive/dentin interfacial bonding. It was hypothesized that plasma irradiations of non-demineralized and demineralized human dentin surfaces would result in significantly higher TBS values when compared to the untreated controls, regardless of the applied plasma devices.
2. MATERIALS AND METHODS
2.1. Sample Preparations
The institutional review board of the Charité – Universitätsmedizin Berlin, approved the collection of extracted human teeth (EA4/102/14). The samples were prepared from 48 caries-free extracted human molars that had been stored in 0.5% Chloramine-T Solution (Carl Roth, Karlsruhe, Germany). From the crown of each tooth, a horizontal slice was cut off with a 0.2 mm band saw (EXAKT 300 CL, EXAKT, Advanced Technologies, Norderstedt, Germany). Each of the obtained specimens was trimmed to a thickness of 2.0 mm by using a grinding machine (LaboPol 25, Struers, Willich, Germany), together with SiC P1000 sandpaper (Buehler, Düsseldorf, Germany). Subsequently, the dentin slices were polished by utilizing SiC P2500 and P4000 sand papers (Buehler, Düsseldorf, Germany). For the demineralization, 24 randomly selected samples were transferred into Buskes solution (2.205 g CaCl2 x 2H2O; 2.041 g KH2PO4; 10.0 ml 100% methylene diphosphonic acid; and 14.3 ml 100% CH3COOH; these were all added to 4.5 L distilled water and then supplemented with 10 M KOH (Merck, Darmstadt, Germany) until the pH was 5.0). This solution was stored for 72 hours in an incubator/shaker at 37°C and at 50 rpm (ES-20 Orbital Shaker-Incubator, Biosan, Riga, Latvia). All of the specimens were then stored in distilled water before being further processed, as follows:
Initially, the slices were air-dried for 3 sec and then etched for 15 sec by using 37% phosphoric acid (Total Etch, Ivoclar Vivadent, Schaan, Liechtenstein). The samples were then rinsed with an air-water-mixture for 15 sec. Subsequently, the non-demineralized and the demineralized specimens were randomly assigned to one of the three treatment modalities in equal parts, so eight samples per group were further processed as follows:
I (CAP I): The plasma jet (kINPen® MED, neoplas tools GmbH, Greifswald, Germany) irradiation was statically administered to the samples’ center for 60 seconds, whereby the plasma source was kept in a perpendicular position and within a distance of 8.0 mm to the dentin surface. The jet was constantly fed with 4.3 sL*min-1 argon.
II (CAP II): A DBD plasma source (PlasmaDerm®, Cinogy, Duderstadt, Germany) was utilized in order to ignite the CAP in the ambient air between the devices’ electrodes and the specimens’ surfaces for 60 seconds, whereby a distance of 1.0 mm was kept between both. III (Control): No additional treatment was performed.
Following the respective treatments, the samples were rewetted with 0.05 ml of 2% Chlorhexidine (Chlorhexamed® Forte, GlaxoSmithKline, Bühl, Germany) for 15 sec by using a microbrush (Appli Tip, Medirel, Agno, Switzerland). According to the manufacturer's instructions, the use of a fourth generation adhesive (OptiBond™ FL Primer and Adhesive, Kerr, Rastatt, Germany) was applied and light-cured for 40 seconds (Bluephase®, Ivoclar Vivadent, Ellwangen, Germany). Consecutively, hollow acrylic glass cylinders (Plexiglas® Tube, Steiner-Technology, Rendsburg, Germany) with an internal diameter of 3.2 mm were filled with a light curing composite (Tetric® Ceram A 3.5, Ivoclar Vivadent, Schaan, Liechtenstein), and attached to the center of the pre-treated dentin surfaces. Any excess composite was carefully removed. Again, light curing was performed from the two sides for 80 sec in total. All of the specimens were transferred into distilled water and thermocycling was conducted for 5000 cycles. The temperature range was 0°C - 55°C and the dwell times and the transfer times were 20 sec and 10 sec, respectively. Before any further processing, the samples were again stored in distilled water at a temperature of 23°C.
2.2. Tensile Bond Strength Testing
After a total wet storage time of 168 hours, the TBS testing was performed by using a computerized universal testing machine (Z010, Zwick Roell, Ulm, Germany) and by applying the parameters as previously described by [22]. A special fixation device was utilized in order to ensure an axial moment-free force application, as well as a self-centering procedure during the pull-off testing. The TBS values were determined by pulling with a constant speed of 2.0 mm/min until a detachment occurred.
2.3. Electron Microscopy Scanning
After the TBS testing, the fracture surfaces of all of the samples were comparatively examined by using a Scanning Electron Microscope (SEM) (CamScan Maxim 2040S, CamScan Electron Optics, Cambridgeshire, United Kingdom) at varying magnifications of up to 1500 x. The SEM images were recorded at an acceleration voltage of 15 kV.
3. RESULTS
3.1. Tensile Bond Strength
In the non-demineralized dentin, the measured means of TBS were 7.14 (CAP I), 16.95 (CAP II) and 4.2 MPa (control), respectively. When compared to the control samples, the TBS values after the CAP I treatment did not differ significantly (p > 0.05; Mann-Whitney U test), whereas the TBS values of the CAP II treatment were significantly higher (p = 0.001). Regarding the TBS values of CAP I and CAP II, no significant differences were detected (p = 0.065).
In the demineralized dentin, the measured means of TBS were 0 (control), 11.68 (CAP I) and 4.6 MPa (CAP II), respectively. When compared to the control samples, the TBS values of both CAP I and CAP II were significantly higher (p = 0.003 and 0.038; Mann-Whitney U test). Additionally, the TBS values after the CAP I treatment were significantly higher than after the CAP II applications (p = 0.05).
When comparing the TBS values of the non-demineralized dentin and the demineralized dentin, significant differences were only detected in the CAP II group (p = 0.009; Mann-Whitney U test), whereas the significance thresholds were not reached within the CAP I group and the control group (p > 0.05) (Fig. 1).
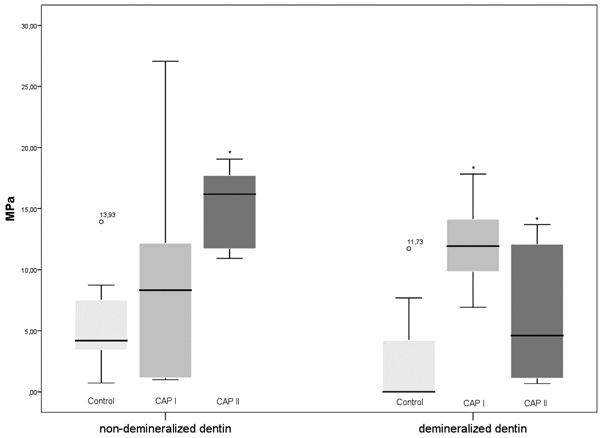
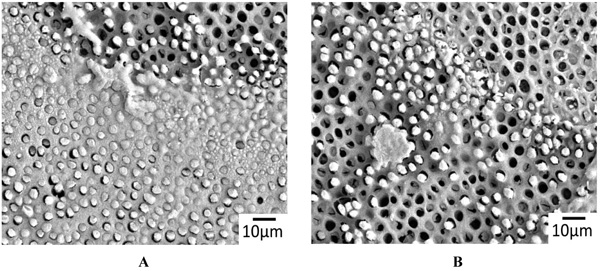
3.2. Electron Microscopy Scanning
The representative SEM images of the non-demineralized and the demineralized dentin control samples showed a variable mixture of fractured resin tags that were located in dentinal tubules, as well as empty tubules, whereby the adhesion failures were located in different depths of the hybrid layer (Fig. 2A). Only isolated areas of separations within the adhesive/composites were evident (Fig. 2B). The corresponding detached composite surfaces showed fractured tags as well as intact tags (Figs. 3A & B). In contrast, extended areas of fractures within the adhesive/composites dominated in the non-demineralized and the demineralized specimens that had been previously treated with CAP I or CAP II Figs. (4A, B & 5A, B).
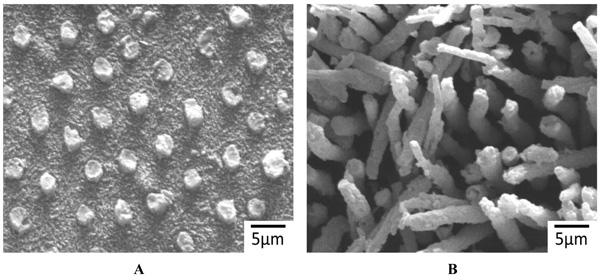
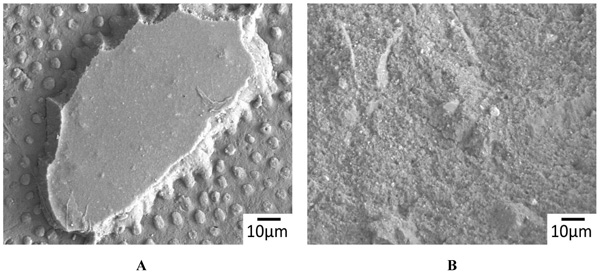
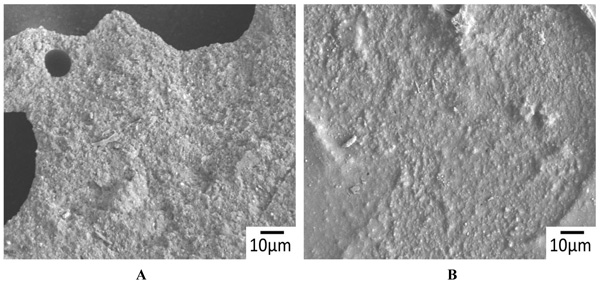
4. DISCUSSION
Cold atmospheric plasmas have previously been described as a way to improve dentin adhesive bonding. These effects have been attributed to an increased wettability of the dentin surface [20, 23, 24]. However, these underlying studies have not investigated if the resulting enhancements of the bonding strengths might differ in size between the different plasma sources, or if they have depended on the degree of (de-) mineralization of the treated dentin. In order to address these questions, the aim of the present study has been to evaluate the influences of CAP irradiation on the two most commonly used types of plasma sources for dentin/adhesive interfacial bonding strength. It was hypothesized that a CAP application would result in significantly higher TBS values when compared to the non-irradiated specimens, regardless of the applied plasma source. Differently from this assumption, only the treatment that used the DBD source resulted in significantly higher TBS values when compared to the control samples in the non-demineralized dentin. However, in the demineralized dentin, the hypothesis was confirmed, whereby the application of the plasma jet was superior to the DBD device when regarding the TBS values.
Overall, the CAP irradiation that was emitted by the DBD source enhanced the TBS values significantly in both the non-demineralized dentin and the demineralized dentin, when compared to the samples that were unexposed to the plasma. Based upon these findings, these devices seem to be promising when regarding a broad use of them in adhesive dentistry. However, the TBS values that were obtained from the non-demineralized dentin were significantly higher than those that were recorded from the demineralized dentin. The demineralization that caused a relative reduction of the polar mineral components might have decreased the wettability to an extent. It is improbable that this situation can be fully compensated, even by a CAP treatment. Thus, it has to be expected that the effect size that is gained from the DBD plasma treatment will at least be diminished in the demineralized areas. This might be seriously considered, when regarding the applied clinical approaches, specifically, the caries excavation strategies. The CAP that was emitted by the plasma jet was found to improve the TBS values, but only in the demineralized dentin when compared to the other groups. It may, therefore, be considered of being applicable, in those teeth that are treated with an incomplete caries removal. In the healthy dentin, the wettability was either not sufficiently improved, or the enhanced wettability was neutralized by the adverse effects that diminished the adhesive/dentin bonding. Even though all of the answers cannot be satisfactorily retrieved from within the present approach, it has to be pointed out that the plasma jets were operated by using a gas stream, which was inevitably directed towards the treated surfaces, causing severe exsiccation. Despite the fact that rewetting was performed before any further processing, the occurred collapse of the exposed collagen structures might have been partially irreversible.
As a consequence, further studies may focus on these assumptions by keeping the dentins wet via applying irrigants during the CAP irradiation. Furthermore, the influences of varying irradiation parameters, as well as extended wetting storage times, might be investigated. In a good accordance with other studies, the TBS values varied within their respective groups. These variable values have previously been described as being due to structural inhomogeneities within the samples, e.g., dentinal sclerosis and inter-individual dentin consistencies [25, 26]. Overall, the obtained results are within a comparable range when related to other research. The TBS values that were measured after seven days were between three and 30 days of wetting storage time, as has been previously reported [27, 28].
CONCLUSION
Cold Atmospheric Plasma Irradiation treatments are a promising approach in order to improve the adhesive/dentin bonding strength in restorative dentistry. However, the use of different plasma sources in the non-demineralized dentin and the demineralized dentin resulted in different TBS values. Within the limitations of the present study, it can be concluded that DBD plasma sources might be favorable over plasma jets. This is especially so, if complete caries excavations are performed before restoration. In demineralized dentin, plasma jets might be superior in terms of improving the strength of adhesive/dentin interfacial bonding.
CLINICAL RELEVANCE
CAPs can potentially enhance the adhesive/dentin interfacial bonding strength, whereby the effect seems to differ between different types of plasma sources and the degree of dentinal (de-)mineralization.
ETHICS APPROVAL AND CONSENT TO PARTICIPATE
The institutional review board of the Charité-Universitätsmedizin Berlin, approved the collection of extracted human teeth (EA4/102/14).
HUMAN AND ANIMAL RIGHTS
No animals/humans were used for studies that are the basis of this research.
CONSENT FOR PUBLICATION
Not applicable.
CONFLICT OF INTEREST
The authors declare no conflict of interest, financial or otherwise.
ACKNOWLEDGEMENTS
The authors would like to thank Dr. Herbert Renz (Department of Craniofacial Developmental Biology, Charité - Universitätsmedizin Berlin) for his most valuable contribution in scanning electron microscopy.