All published articles of this journal are available on ScienceDirect.
Evaluating Resin-Dentin Bond by Microtensile Bond Strength Test: Effects of Various Resin Composites and Placement Techniques
Abstract
Objectives: This in vitro study evaluated the microtensile bond strength (MTBS) of a methacrylate- based compared to a silorane-based resin composite in Class I cavity using different placement techniques.Materials and Methods: Class I cavities with dimension of (4 mm long, 4 mm wide, 3 mm deep) were prepared in extracted sound human molars. The teeth were randomly divided into six groups. The first three groups were filled with Filtek P90 using three methods of insertion; bulk, incremental and snow-plow, and the remaining three groups were filled with Clearfil AP-X using the same three placement techniques. After 24 hours of storage in water at 37°C, the specimens were thermocycled to 1000 cycles. Specimens were prepared for MTBS testing by creating bonded beams obtained from the pulpal floor. Statistical analysis used: Statistical analyses of data were performed by two-way ANOVA/Tukey (α=.05). Results: The experiment showed significant differences between the two resin composites with regard to filling techniques (P<0.05). The MTBS was significantly higher in each of Filtek P90 subgroup compared to Clearfil AP-X ones (P<0.05). With respect to filling technique in both resin composites, bulk insertion showed the significantly lowest MTBS (P<0.05), while no significant difference was found between the outcome of incremental and snow-plow techniques (P>0.05). Conclusion: Silorane-based resin composite as opposed to methacrylate based resin composite and layering placements in contrast to bulk filling method had higher microtensile bond strength.
INTRODUCTION
Polymerization shrinkage of resin composite materials is a major impediment to their clinical durability over time. Many contemporary composite materials are based on methacrylate chemistry. During the process of polymerization, volumetric shrinkage occurs, ranging from 1.5 to 5% [1]; and these internal stresses are transferred to the tooth/restoration interface as tensile forces [2]. Polymerization contraction stress produces powerful forces that can debond the material from dentin. Some factors can influence stress formation such as volumetric polymerization shrinkage, adherence of the resin composite to the cavity walls, the elastic modulus, the flow ability of the resin composite, and the cavity configuration factor (C-factor). Regarding the C-factor, the ratio of the bounded surface to the unbounded area grows, when the polymerization shrinkage increases [3, 4]. To minimize volumetric shrinkage efforts have been directed toward slowing down the composite polymerization rate [5], as well as developing a none-shrinking high performance polymer for use as a matrix material for dental composites [6].Siloran-containing resin monomers have been developed from the reaction of cycloaliphatic oxirane rings and siloxane backbone. They possess two key advantages: volumetric shrinkage less than 1% due to ring opening during polymerization [7], and increased hydrophobicity owing to the presence of silorane species [8]. Silorane-based composites have proved to exhibit good mechanical properties comparable to those of the methacrylate-based composites [1, 7, 9]. For methacrylate-based composites, the incremental placement technique has been considered to minimize the effects of the polymerization shrinkage [10]. On the other hand, the silorane resin composite had lower incremental bonding properties compared with metacrylate composite [11], and therefore, bulk placement can be considered as a suitable approach. Many resin composites used as posterior restorations are not able to flow easily; hence, the use of a liner to act as a flexible intermediate layer between restoration and tooth structure has been suggested to relieve the shrinkage stress [12]. Flowable resin composites have been recommended as elastic liners due to their low viscosity and high elasticity [13]. However, there is scarce published information about using un-cured mixture of flowable and regular resin composites as snow-plow method in Class I cavities that commonly consist of both enamel and dentin walls with high C-factor. The aims of the current study were to compare the effects of different resin monomer systems and various insertion techniques on the microtensile bond strength of Class I resin composite restorations.
MATERIALS AND METHODS
Thirty-six extracted erupted non-carious, non-restored, human third molars were gathered following informed consent approved by the Commission for Medical Ethics of the University of Medical Sciences (N#900672). Teeth were selected, disinfected and stored in normal saline solution until use. Pumice stone was used to clean the molars of soft tissue. The occlusal enamel was trimmed at the level of the main grooves using a slow-speed disc (KG Sorensen, Barueri, SP-Brazil) under copious running water, exposing an occlusal flat enamel surface. Uniform box-shaped Class I cavities were prepared, measuring approximately 4 mm (mesial-distal) × 4 mm (buccal-lingual) × 3 mm deep at the occlusal crown center, using diamond burs (Brasseler, Savannah, GA, USA) in a high-speed handpiece with copious air-water spray. The burs were replaced after every five preparations. The cavosurface margins were prepared at 90 degree. All external prepared Class I cavities consisted of enamel and dentinal walls, and the pulpal floor lay on dentin. The uniformity of cavity preparation was a critical factor for the study, because having cavities with similar dimensions is essential to inserting and photo activating a standardized volume of composite in each sample. These dimensions yielded a box-shaped cavity with a C-factor of 4 (bound surface/unbound surface area = 64 mm2/16mm2= 4). The prepared teeth were then randomly divided into two main groups according to the two types of resin restorative materials; silorane-based resin composite (Filtek P90, 3M ESPE) or methacrylate-based resin composite (Clearfil AP-X, Kuraray, Japan). Either Filtek Silorane System Adhesive with Filtek P90 resin composite or Clearfil SE Bond with Clearfil AP-X resin composite (shade A2) was applied to the cavity according to the manufacturer's instructions (Table 1) and was then light polymerized using a quartz-tungsten halogen Optilux 501 unit (Demetron- Kerr, Orange, CA, USA) set at 600 mW/cm2. The light intensity was checked with the digital radiometer. Each of the two main groups (n=18) was subdivided into three subgroups (n=6) based on three different resin composite placement techniques as below:
-Bulk: resin composite was placed in one bulk and cured for 40 seconds.
-Incremental: resin composite build-ups were constructed in two 1.5 mm-thick horizontal layers, which were individually light polymerized for 20 seconds.
-Snow-plow: Firstly, it was inserted 0.5 mm-thickness of un-cured Filtek Z350 flowable composite. Then 1 mm-thick of resin composite was condensed into the un-cured flowable resin composite and the excess of flowable resin composite was removed with an explorer, which was followed by light curing for 20 seconds. The rest of the cavity was filled by regular resin composite. The samples of each group were cured additionally for 40 seconds from occlusal direction.
MICROTENSILE BOND STRENGTH (MTBS) TESTING
All the restored specimens were stored for 24 hours at 37°C in distilled water and subjected to a thermocycling process (custom procedure made by Nemo Institute for Dental Materials, Mashhad, Iran) for 1000 cycles between 50°C and 55°C with a dwell time of 30 seconds and transfer time of 6 seconds. Under copious amount of water, a sectioning machine (CNC, Nemo, Mashhad, Iran) was used to serially section the specimens perpendicular to the bonded pulpal floor surfaces in both x and y directions to obtain bar-shaped specimens with a 0.81 mm2 cross section. Next, each beam was visually analyzed to select suitable bars with the flattest interfaces for the microtensile bond strength test. The cross-sectional area of each specimen was measured with a digital calliper (Absolute Digimatic, Mitutoyo, Tokyo, TYO, JPN) and recorded for measuring the bond strength. The beams were then fixed to a holding device with cyanoacrylate glue (Model Repair II Blue, Dentsply-Sankin, Tokyo, Japan) and tested in tension in a universal testing machine (Santam, Tehran, Iran) at a cross-head speed of 1 mm/min equipped with a load cell of 100 N until failure (Fig. 1). The MTBS values were calculated in megapascals (MPa) by the following equation: MPa= N/mm2. The failure mode of each beam was evaluated with a stereomicroscope (Dino-Lite Pro, AnMo Electronics Corp, Taiwan) at a magnification of up to 50×. Failures were categorized as cohesive (occurring within dentin or composite), adhesive (occurring between the two materials), or Mixed (a combination of cohesive and adhesive). Statistical comparison of the microtensile bond strength values were evaluated by two-way ANOVA and Tukey’s Multiple comparisons test at a significance level of α=0.05.
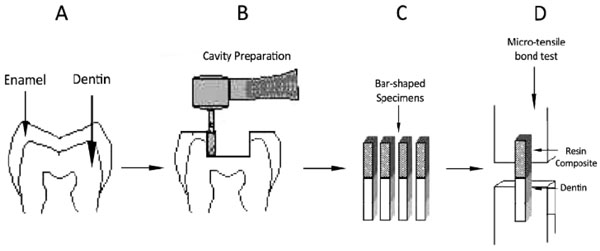
Specimens preparation for microtensile bond strength test: A) Molar tooth sample B) Class I cavity preparation for resin restoration; C) resin-dentin sticks with a rectangular cross-sectional area approximately .9 mm × .9 mm; D) microtensile bond strength test at a crosshead speed of 1 mm/minute.
Materials, chemical compositions and procedure used in the study.
Procedure | Chemical composition | Materials (Manufacturer) | |
---|---|---|---|
The primer applied to the entire cavity wall and massaged over the entire area for 15 sec. A gentle stream of air used. The primer cured for 10 sec. | phosphorylated methacrylates, Vitrebond copolymer, HEMA, Bis-GMA, water, ethanol, silane-treated silica filler, initiators, stabilizers | Primer | Silorane System Adhesive (SSA) (3M ESPE) |
The bond applied to the entire cavity wall. A gentle stream of air used. The bond cured for 10 sec. | hydrophobic dimethacrylate, phosphorylated methacrylates, TEGDMA, silane-treated silica filler, initiators, stabilizers | Adhesive | |
The primer applied to the entire cavity wall and leaved it in place for 20 sec. The volatile ingredients evaporated with a mild air stream. | HEMA , 10-MDP, hydrophilic dimethacrylate, water, accelerators, dyes, camphorquinone | Primer | Clearfil SE Bond (Kuraray,Tokyo, Japan) |
The bond applied to the entire cavity wall. A gentle stream of air used. The bond cured for 10 sec. | Bis-GMA , HEMA, 10-MDP, hydrophilic dimethacrylate, colloidal silica, initiators, accelerators, dyes, camphorquinone | Bond | |
Silane treated quartz, 3,4-epoxycyclohexylcyclopolymethylsiloxane, Bis-3,4-epoxycyclohexylethyl-phenylmethylsilane, yttrium tri fluoride | Filtek P90 (3M ESPE) | Resin Composite | |
Bis-GMA, TEGDMA, silanated barium glass filler, silanated silica filler, silanated colloidal silica, dl-camphor Quinone, catalysts, accelerators, pigments | Clearfil AP-X (Kuraray, Tokyo, Japan) | ||
Silane treated ceramic, BIS-GMA,TEGDMA,BIS-EMA, silanetreted silica and zirconium oxide, functionalized dimethacrylate polymer | Filtek Z350 flowable restorative (3M ESPE) |
Abbreviations: HEMA, 2-hydroxyethyl methacrylate; Bis-GMA, bisphenol A diglycidylmethacrylate; TEGDMA, triethylene glycol dimethacrylate; 10-MDP, 10-Methacryloyloxydecyl dihydrogen phosphate; BIS-EMA, ethoxylated bisphenol A glycol dimethacrylate.
Mean values and respective standard deviations of microtensile bond strength (MPa) in experimental groups.
Resin composite | Placement technique | Mean (Mpa) | Std. Deviation | N | P-value |
---|---|---|---|---|---|
Filtek P90 | Bulk | 15.6800 a | 5.54238 | 12 | <0.000 |
Incremental | 47.7000 b | 6.23822 | 13 | ||
Snow-plow | 41.2367 b | 8.32955 | 12 | ||
Clearfil AP-X | Bulk | 5.0992 c | 1.13913 | 11 | <0.000 |
Incremental | 20.2400 d | 3.63996 | 12 | ||
Snow-plow | 27.3542 d | 2.58572 | 12 | ||
P-value | P<0.05 |
The fracture pattern mode % (N) of each experimental group.
Resin composite | Placement technique | Adhesive | Cohesive in resin | Cohesive in dentin | Mixed |
---|---|---|---|---|---|
Filtek P90 | Bulk | 16.7(2) | 58.3(7) | 8.3(1) | 16.7(2) |
Incremental | 23.1(3) | 61.5(8) | 7.7(1) | 7.7(1) | |
Snow-plow | 16.7(2) | 75.0(9) | 0.0(0) | 8.4(1) | |
Clearfil AP-X | Bulk | 18.2(2) | 9.1 (1) | 0.0(0) | 72.7(8) |
Incremental | 8.3 (1) | 25.0 (3) | 16.7(2) | 50.0(6) | |
Snow-plow | 16.7(2) | 33.3(4) | 0.0(0) | 50.0(6) |
RESULTS
The Mean and standard deviations of each group are displayed in Table 2. The cross-sectional area of the MTBS specimens ranged from 0.72 to 0.9 mm2, with a mean value of 0.81±0.1 mm2. Two-way ANOVA detected a cross-product interaction (P=0.024). The means and standard deviations (MPa) of this test are shown in Table 2. The MTBS was significantly higher in each of the Filtek P90 subgroups compared to Clearfil AP-X ones (P<0.05). With respect to filling technique in both resin composites, bulk filling significantly showed the lowest MTBS (P<0.05), while no significant difference was found between incremental and snow-plow techniques (P>0.05). There was no significant difference among the groups concerning mode of failure (P>0.05). Table 3 demonstrates that the highest number of cohesive failure in resin occurred in case of Filtek P90, whereas the higher mixed failure was observed with Clearfil AP-X resin composite.
DISCUSSION
The main objective of the bond strength tests is to evaluate bonding efficacy of adhesive systems to the dental hard tissues. In the present study, the MTBS test was used as a method to evaluate the adhesion index of adhesive systems to dentin. With regard to the filling technique, Silorane-based composite presented significantly higher MTBS than methacrylate-based resin composite. Giacobbi and Vandewalle evaluated microtensile bond strength of Silorane System Adhesive (SSA) and Clearfil SE Bond adhesive systems and found no significant difference between the two adhesive agents. However, after 12 months of storage in water, Clearfil SE Bond lost 53% of its original bond strength, while SSA lost 41% [14].Silorane System Adhesive is a two-step self-etch adhesive, comprising a separate primer (SSA self-etch primer) and adhesive resin (SSA Bond) that require separate light curing. SSA-Bond contains hydrophobic bifunctional monomers to match the hydrophobic silorane resin. It was revealed that SSA effectively bridged the hydrophilic tooth structure with the hydrophobic silorane composite [15]. Besides providing a compatible link to the silorane matrix, this hydrophobic SSA-Bond can be expected to have sealed off dentin surface by blocking osmotic water-sorption from dentin, which can be beneficial with regard to bond stability [16-19]. These properties, amongst others like possibly a more favorable polymerization conversion of SSA, may altogether have contributed to the higher MTBS obtained for Filtek P90 [14, 20]. Filtek P90 has a lower filler content of 79% by weight compared to Clearfil AP-X (86% by weight). It was reported that bond strength increased with the rise in the filler content of composites [21]. However, one study recently investigated the effect of filler content on MTBS in Class I cavities. The authors showed that the filler content did not influence the MTBS of resin composites [22]. The result of the present study also confirmed this finding. With respect to filling technique, bulk filling resulted in the significantly lowest MTBS in both resin composites. The previous studies also reported advantages of incremental
filling technique [23-28]. Incremental filling of the cavities can reduce the C-factor and thus the effect of polymerization stress, which is especially important for conventional composites. Other factors are more likely to improve the bond strength of silorane composite. Adaptation of a relatively stiffer resin composite might have been more appropriate. Furthermore, excess intense light curing at the cavity bottom and interface level might have occurred due to lower material thickness. It is mentioned in a previous study that a lower depth of cure and degree of conversion is achieved with silorane-based composites compared to metacrylate-based composites [29].Guiraldo et al. also reported that even though there was no difference in light transmission between silorane- and metacrylate-based composites, silorane-based composites were not as well polymerized below the surface as metacrylate-based composites [30]. So, it seems that less polymerization and gradual build-up of the silorane-based resin composite modulus in comparison to the metacrylate–based resin composite, allow the material to absorb a considerable amount of shrinkage stress, thus minimizing the effect of contraction forces at the tooth-restoration interfaces as well as causing higher bond strength compared to metacrylate–based resin composite. Snow-plow technique is a purposed method for Class II cavities that extend to gingival floor, which enriches the dentin more than the enamel [31]. In present study, the use of flowable composite in snow-plow method showed no significant effect on MTBS of resin composites compared to incremental filling. Previous studies showed varying results with regard to the use of lining materials [27, 32]. The low stiffness of flowable composites might compensate for the polymerization shrinkage of the resin composites [33]. In the present study, MTBS of Clearfil AP-X resin composite in snow-plow group was higher than that of the incremental group; however this increase was not significant. It seems that using snow-plow placement method may decrease voids in the first layer volume of resin composite restorations. With respect to failure mode, mixed failures were predominant for all tested groups of Clearfil AP-X resin composite, which is in agreement with previous studies [32, 34]. The most predominant fracture pattern for silorane composite was cohesive failure. This finding is not in correspondence with the failure pattern observed in previous study [24]. It was probably that lower depth of cure and degree of conversion and reduced polymerization below the surface for silorane-based composites compared to metacrylate-based composites may be the responsible for high incidence of cohesive fauiler in resin composite, regarding Filtek P90 in this study. Various material properties like polymerization shrinkage and non- material related factors such as cavity configuration and filling technique seem to play a role in modifying the bond strength. These influencing factors may be rather similar or rather varied in different studies and may account for divergent results reported in these different evaluations. Furthermore, this pilot in-vitro study requires further long term in-vitro and in-vivo implementation with higher sample size, using various adhesive systems, resin composites, and depth of dentin or cavity type, since factors like dentinal fluid and resistance to hydrolytic degradation of resin materials may affect dentin bonding.
CONCLUSION
In view the limitations of this study, it could be concluded that silorane-based resin composites opposed to methacrylate based resin composite had higher microtensile bond strength with regard to the filling technique. The incrementally and snow-plow placement techniques remain recommended, even for low-shrinking resin composites.
CONFLICT OF INTEREST
The authors confirm that this article content has no conflict of interest.
ACKNOWLEDGEMENTS
The authors would like to acknowledge the research chancellor of Mashhad University of Medical Sciences for providing this research with financial support (grant number 900672). The results described in this paper have been taken from a DDS, MS undergraduate student thesis (# 2590).