All published articles of this journal are available on ScienceDirect.
Effect of Short-Time Povidone-Iodine Application on Osteoblast Proliferation and Differentiation
Abstract
Background and Objective:
Povidone-iodine [polyvinylpyrrolidone-iodine complex (PVP-I)] is a broad-spectrum antimicrobial agent, frequently used in dentistry. In this study we investigated the short- and longterm effects on osteoblast number, viability, and function after short exposure to PVP-I with and without additional bone-morphogenetic protein-2 (BMP-2).
Material and Methods:
Confluent osteoblast-like cell line (MC3T3-E1, subclone 24) cultures were exposed to pure PVP-I solution (7.7 mg/ml) and dilutions of 1:10, 1:100 and 1:1000 for 10 seconds and washed with phosphate buffer solution. Cell proliferation and viability was determined by MTT and differentiation status by alkaline phosphatase (ALP) activity 6 days after initial plating. In a separate experiment, long-term cell proliferation, viability and function were assessed 4 weeks after PVP-I treatment by MTT and deposited calcium using an Alizarin-red staining test.
Results:
PVP-I decreased ALP activity substantially. Stimulation by BMP-2 recovered ALP activity to near control levels at 1:100 and 1:1000 dilutions of PVP-I. The MTT assay showed reduced proliferation of the preosteoblastic cells for all treatments, irrespective whether BMP-2 was used or not. Only at PVP-I dilutions of 1:1000 proliferation rate was back to normal levels (95.6±2.4 %). No adverse long-term effect of PVP-I on mineralization of the extracellular matrix (Alizarinred) for dilutions higher than 1:100 was observed. Interestingly, undiluted and 1:10 diluted PVP-I even showed a significant increase in mineral deposition, especially in the presence of BMP-2.
Conclusion:
Short-time application of PVP-I in concentrations of 1:10 and higher lead to decreased viability and impaired differentiation. However, surviving cells showed good recovery and mineralization potential.
INTRODUCTION
Periodontitis is a bacterial infection modified by systemic and environmental factors [1]. The main treatment is the effective reduction of supra- and subgingival pathogenic flora by mechanical means. A variety of antibiotics and antiseptics have been introduced as adjunct to mechanical therapy [2]. Whereas the use of antibiotics is warranted in certain cases, especially in patients with insufficient response to non-surgical therapy, it is associated with certain risks, notably the development of antibiotic resistance in various bacterial species [3]. Administration of antimicrobial agents as a quick and inexpensive means of supporting mechanical periodontal debridement is worth consideration [4]. Antiseptic agents prevent or arrest the growth or action of microorganisms. They have a considerably broader spectrum of activity than antibiotics and, in contrast to antibiotics, often aim at multiple intracellular targets, which reduce the likelihood of resistance development [4]. However, since antiseptics, unlike antibiotics, are potentially toxic to both infectious and host cells, their application in humans is limited to infected wounds, skin and mucosa. Povidone–iodine [polyvinylpyrrolidone–iodine complex (PVP-I)] is an iodofor with a broad spectrum and high antimicrobial activity, low potency for developing resistance and adverse reactions, and low financial cost [5]. In the oral cavity it is used at dilution levels of 1 to 10% in several conditions and its utility has also been reported in non-surgical periodontal therapy [4-7]. Like other antiseptics, PVP-I has a non-selective mechanism of action, and the high concentrations used in the oral cavity may eventually adversely affect the host cells. In vitro studies have reported deleterious effects on fibroblasts and osteoblasts [8, 9]. A recent in vitro study by Cabral & Fernandes [9] using osteoblastic human alveolar bone cells has shown that 5 and 10% PVP-I caused cell death within minutes; contact with 1% PVP-I resulted in a loss of the characteristic elongated cell shape.
The aim of the present study was to investigate short- and long-term effects on preosteoblastic cells and maintenance of their osteoblastic lineage by BMP-2 application following a 10 second exposure to PVP-I which mimics the clinical situation during periodontal interventions.
MATERIAL AND METHODOLOGY
We have chosen murine MC3T3-E1 cells, since osteoblastic cells are exposed to PVP-I during periodontal interventions. MC3T3-E1 cells represent a well characterized cell line of the osteoblastic lineage which undergoes all stages of differentiation from preosteoblasts to mature osteoblasts in vitro. MC3T3 secrete bone morphogenetic proteins (BMP) and differentiate by the action of BMP to mature osteoblasts. An accelerated differentiation towards mature osteoblasts can be induced by exposure to additional BMP-2 and can be monitored by an increase in alkaline phosphatase (ALP) activity. ALP activity is an early marker for osteoblast maturation. The cellular activity of mature osteoblasts is characterized by the mineralization of their extracellular matrix. Therefore, mineralization is a late marker for osteoblast maturation. Quantification of matrix mineralization can be performed by the dye Alizarin red which stains deposited calcium.
Activity of mitochondrial dehydrogenases (MTT-test) substituting for the radioactivity based tritiated thymidine incorporation assay was used to determine cellular activity and proliferation. Live/dead staining was used to identify living cells based on their ability to convert a calcein derivative into its fluorescent equivalent which is mediated by intracellular esterase enzyme activity. Dead cells are detected by ethidium bromide, since only in leaky cells the DNA is exposed allowing ethidium bromide binding.
Cell Line, Culture Technique and Alkaline Phosphatase Determination
MC3T3-E1 cells of passage below 20 were supplied by ECACC (Porton Down, Salisbury; Great Britain) and grown in an alpha-modified Minimum Essential Medium (Invitrogen/Gibco) containing 10 % fetal calf serum, 50µg/ml penicillin/streptavidin, and 50 µg/ml ascorbic acid. For BMP treatment, the culture medium was supplemented with 1 µg/ml rhBMP-2. To examine the biological effect of antiseptics, 1x105 cells were plated in each well of 6-well plates and grown to confluency for 2 days. On day 3 the cell culture medium was aspirated and 1 ml antiseptic (PVP-iodine, B. Braun Medical AG, Switzerland) added, either undiluted (7.7, mg/ml) or diluted volume/volume (1:10, 1:100 and 1:1000) in phosphate buffered saline (PBS). Chlorhexidine (CHX) at a concentration of 0.2% (Kantonsapotheke, Zürich, Switzerland) was used as a control agent. After an exposure time of 10 seconds, the respective antiseptic solution was aspirated and the cells washed twice with 4 ml of PBS to remove all residual antiseptics, before 4 ml of culture medium were added. Medium exchange was performed on day 4 and alkaline phosphatase (ALP) determined on day 6. The cells were washed 3 times with phosphate buffered saline, and the cells from a single well were combined in 0.5 ml of lysis buffer (0.56 M 2-amino-2-methyl-propane-1-ol pH 10), and homogenized using an omni-mixer. 200 µl of the cell lysate were mixed with 200 µl of lysis buffer supplemented with 20 mM p-nitrophenylphosphate, and 4 mM MgCl2 at 4°C, allowing the determination of ALP activity. ALP activity was normalized to total protein and expressed as nmol nitrophenylate generated per min per mg protein.
Cell Proliferation
MC3T3 cell proliferation was assessed using the thiazolyl blue tetrazolium (MTT; SigmaAldrich) dye reduction assay. Briefly, MC3T3 cells were grown in 6 well plates as described for ALP activity measurements. At selected time points, MTT (500µl; 5mg/ml in phosphate buffered saline) was added to each well and incubated for 4 hours at 37°C in the dark. Following incubation, MTT was aspirated from each well and MTT-formazan crystals were solubilised by the addition of isopropanol (200µ l; 1N HCl). Absorbance of each well was then measured at 570 nm.
Calcium Deposition by Preosteoblastic Cells
MC3T3-E1 cells were plated at a density of 30,000 cells/cm2 in 6 well plates. Exposure to antiseptics was performed as described earlier. From day 3 on the culture medium was supplemented with 50 µg/ ml ascorbic acid and 10 mM β-glycerophosphate. Medium was changed once a week and calcium deposition determined 4 weeks after the exposure of the cells to the antiseptics. For Alizarin red staining of calcium, culture cells were washed once with phosphate buffered saline and fixed for 1 hour in 70% ethyl alcohol. After rinsing the cells with water, 1 ml of an Alizarin red solution (40 mM, pH 4.2) was added, and the cells were stained for 10 minutes. Non-specific staining was removed by 5 washes with water and 15 minutes incubation in PBS, before the stained wells were photographed. For quantization, cells stained with Alizarin red were destained with 10% cetylpyridinium chloride in 10 mM sodium phosphate, pH 7.0. Calcium complexed by Alizarin red was determined in the distaining solution at OD562 and the amount of calcium calculated according to a standard calcium curve respectively.
Cell Live/Dead Staining: (Calcein AM/Ethidium Bromide (EtBr)
The live/dead staining is based on Calcein AM, a substrate which is converted intracellularely into fluorescent calcein by intracellular esterases, only retained in cells with an intact plasma membrane. Ethidium bromide is a fluorescent nucleic acid stain that is only able to pass through disrupted nuclear membranes of dead cells. Briefly, for the live/dead discrimination, the cell medium was supplemented with calcein (5 µM). Following an incubation of 30 minutes, the supernatant was aspirated and the cells washed once with Tris-buffered saline (TBS; 10 mM Tris; 150 mM NaCl, pH 7.4). Next, the cells were exposed to EtBr (10 µg/ml in TBS) for 2 minutes at RT followed by two washes with TBS whereupon an inspection under the fluorescence microscope was performed. Calcium fluorescence was determined at λex=496 nm and λem=516 nm, and assessed using a FITC filter. EtBr was analysed at λex=518 nm and λem=605 nm, using a rhodamine filter.
Statistical Analysis
Student's t-test was implemented by a commercially available software package (SSPE, Chicago, Il). All values are represented as means ± standard deviations of at least 2 independent experiments, each performed in triplicates. P<0.01, indicated by ** was set for statistical significant differences.
RESULTS
The short-term effect of PVP-I determined by changes in ALP activity showed that a 10 second exposure to PVP-I decreased ALP activity substantially (Fig. 1A). Even 1:1000 dilutions of PVP-I lowered ALP activity to 63.5±10.2 % (P<0.01, N=6) of untreated cells indicating a dedifferentiation effect of PVP-I on preosteoblastic cells. Exposure of preosteoblasts treated with 1:100 dilutions of PVP-I to the differentiation factor BMP-2 recovered ALP-activity to near control levels (98.6±5.0 %). At 1:1000 dilutions of PVP-I BMP-2 exposure increased ALP activity above control levels (111.6±12.2 %) although this increase was not statistically significant. These results suggest that PVP-I exposure retards the differentiation of osteoblasts as seen by the reduction in ALP activity without blocking the susceptibility of the preosteoblasts to the differentiation factor BMP-2.
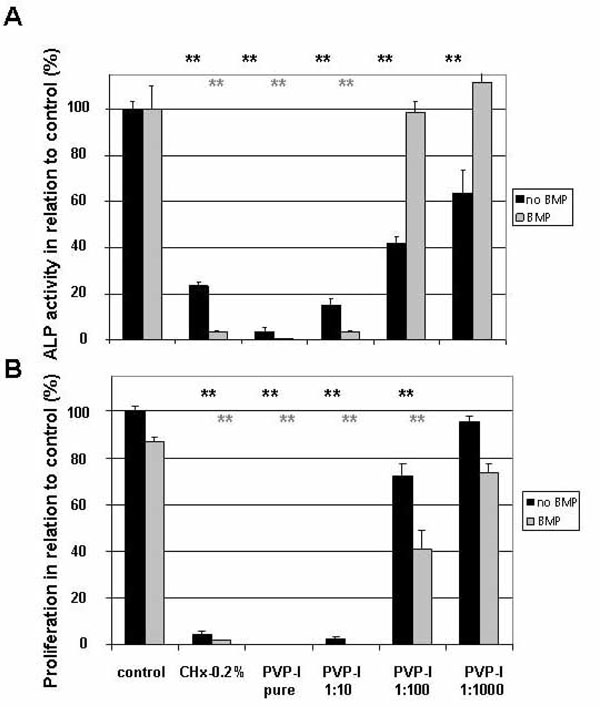
Short term effect of PVP-I treatment on ALP activity and proliferation. Preosteoblastic cells were exposed to different concentrations of PVP-I on day 2 after seeding and ALP activity was determined on day 6. (A) ALP activity without exposing MC3T3-E1 cells to 1 µg/ml BMP-2 was significantly reduced compared to the control even beyond PVP-I dilutions of 1:1000 (**). In case that MC3T3-cells were stimulated by the osteogenic differentiation factor BMP-2, a significant (**) decrease in ALP activity below the control level was only observed in cells exposed to PVP-I dilutions of 1:10 or below. (B) MTT test revealed that only dilutions of PVPI up to 1:100 reduced proliferation rates significantly below the control level, irrespective of exposure to BMP-2 (**) or not (**). ** indicates P values of a simple t-test below 0.01 when compared to the control.
The MTT assay was used to determine proliferation rate of the preosteoblasts. As shown in Fig. (1B), all treatments with the exception of the PVP-I dilution 1:1000, including exposure to BMP-2, reduced the proliferation of the preosteoblastic cells. At PVP-I dilutions of 1:1000, proliferation rate was back to normal levels (95.6±2.4 %) although ALP activity was still much lower than in the control cells (Fig. 1A). Thus, preosteoblastic cells exposed to 1:1000 dilution of PVP-I show a decrease in their differentiation status in the short-term despite the fact that proliferation rate had recovered to normal levels. When the differentiation factor BMP-2 is added, however, ALP activity recovered to normal levels already at 10 fold higher PVP-I concentrations. Therefore, preosteoblastic cells exposed to PVP-I, even if diluted 1:1000 have partially lost their osteogenic differentiation level but maintain their susceptibility to osteogenic signals like BMP-2.
The long-term effect of PVP-I on mineralization of the extracellular matrix was assessed by alizarin-red binding to calcium depositions. Mineralization determined 4 weeks after the exposure to PVP-I was normal for dilutions 1:100 and higher (Fig. 2A). Therefore, exposure of preosteoblasts to PVP-I dilutions 1:100 and higher seems to have no negative effect on the maturation of preosteoblasts to osteoblasts in the long-term, both for untreated and BMP-2 treated cells. Interestingly, undiluted and 1:10 diluted PVP-I showed a significant increase in mineral deposition (Fig. 2A) suggesting that a certain level of cellular activity survives even this harsh treatment. The CHX-0.2% treated cells with BMP could not be evaluated because the cells and their extracellular matrix always detached over the period of 4 weeks.
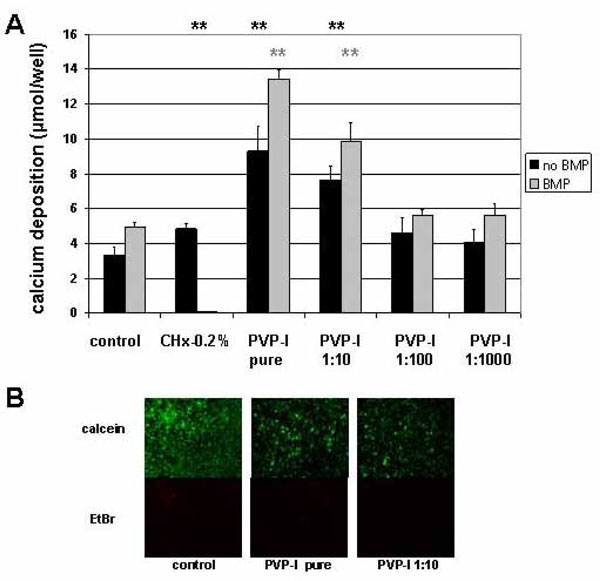
Long-term effect of PVP-I treatment on preosteoblastic cells. Preosteoblastic cells were exposed to different concentrations of PVP-I on day 2 after seeding and evaluated 4 weeks later. (A) Alizarin-red staining method was used to determine mineral deposition. Dilutions of PVP-I of 1:100 and higher had no effect on the calcium deposition into the extracellular matrix. Exposure to undiluted or 1:10 diluted solutions of PVP-I, however, increased mineral deposition compared to the control untreated or respective BMP treated sample significantly (**) (**). (B) Live/dead staining revealed the presence of a majority of calcein stained living cells and a minority of EtBr stained dead cells in control samples. Although to a much lower extend living cells were also detected in samples treated with pure PVP-I or 1:10 dilutions. This minor fraction of surviving cells could account for the extended mineral deposition detected in the respective samples.
To further assess this finding live/dead staining was performed in preosteoblastic cells exposed to PVP-I pure and 1:10 PVP-I dilutions and compared to untreated cells. 4 weeks after exposure of preosteoblastic cells to PVP-I, fluorescent calcein signal indicative for living cells is present in all samples although to a much lower extent than in the untreated control sample (Fig. 2B). Therefore, a minor fraction of the cells survive a short 10 second long exposure to 1:10 diluted PVP-I and pure PVP-I since they maintain an intact cell membrane enclosing active esterases necessary for a positive readout for fluorescent calcein detection. This minor fraction of cells could potentially be responsible for the more intense mineralization of the matrix, suggesting that these cells have a higher mineralization potential than untreated cells.
DISCUSSION
This study assessed the short- and long-term effects on osteoblast proliferation, viability, and function after short exposure to PVP-I. The existing literature concerning this topic is scarce, especially when focusing on dental tissues. Two studies have demonstrated that PVP-iodine is cytotoxic to dental tissue, i.e. fibroblasts and osteoblasts [8, 9]. In accordance to these data, we found a significant decline in cell proliferation and ALP activity after short-time application of higher PVP-iodine concentrations (up to 1/10) but we also found effects on the differentiation status of preosteoblasts, an aspect, which had been overlooked so far.
The results of the present study suggest that PVP-I in concentrations of 1:10 and higher lead to decreased viability and loss of differentiation towards the osteogenic lineage although these cells were already determined to become mature osteoblasts. The application of BMP-2 can overcome this loss of determination and redirect these cells towards the osteoblastic lineage (Fig. 1A). Since preosteoblasts deposit autologous BMP in the extracellular matrix one could speculate that PVP-I treatment reduces the osteogenic cues already deposited into the extracellular matrix by inactivating autologous BMP. Therefore, a therapeutic application of BMPs or other bone formation stimulating agents following PVP-I treatment may be an appropriate approach, which merits further investigation.
In the long-term, however, preosteoblasts exposed to PVP-I will recover and mature to osteoblasts even without addition of BMP since the cells will continue to deposit osteogenic cues into the newly formed extracellular matrix (Fig. 2A). Prerequisite would be that osteogenic cells will repopulate disinfected areas. If not, osteogenic cues like BMP-2 should be added to the extracellular matrix of the disinfected and washed area.
This transient effect on the differentiation let this antiseptic to appear as a considerable alternative for other effective disinfection normally applied during periodontal surgery. Further clinical studies are, however, needed to clear these aspects. In this context it is important to mention that the clinical situation greatly differs from the laboratory model. Data concerning the penetration of antiseptic agents into these vital tissues is scarce and the real concentrations during application under clinical conditions can only be estimated. Studies used different concentrations ranging from 0.1 % to 10 % [10-12], but no mandatory recommendations are given in literature so far. Laboratory conditions cannot be directly transferred to the situation of the bacterial biofilm in periodontal pockets in vivo. In the clinical situation, dilution of the antimicrobial agent must be anticipated due to bleeding caused by mechanical instrumentation and due to concomitant rinsing. Several inactivating reactive substances of the blood and serum may additionally reduce the substantivity of iodine. This might explain that albeit cytotoxic effects in vitro, wound healing in vivo is less affected [13]. In addition, reparative and regenerative periodontal processes are guided by the surrounding tissues, namely epithelium, connective tissue, periodontal ligament and bone which act as a source of growth factors and cells. Both will substitute for loss of cells in the treated area but also redirect surviving cells into the appropriate lineage.