All published articles of this journal are available on ScienceDirect.
Antibacterial Activity of Curcumin-loaded Mesoporous Silica Nanoparticles against Streptococcus mutans
Abstract
Background
Streptococcus mutans is an important contributor to tooth decay. Curcumin exhibits minimal side effects and has broad applications in various antimicrobial research. Although curcumin derivatives have a wide range of medical effects, their inherent physicochemical parameters, such as poor water solubility, chemical instability, low bioavailability, short half-life, and rapid metabolism, make their medicinal use challenging. Nanoparticle drug delivery systems can enhance the efficacy of curcumin in treating several diseases, especially infectious diseases.
Aims
This present study aimed to investigate the antibacterial effect of curcumin-loaded mesoporous silica nanoparticles (Cur-MSNs) on S. mutans and their biofilm form and adhesion.
Objectives
Recently, novel approaches have been utilized to overcome pharmacological issues and increase the therapeutic effectiveness of curcumin. Among them, nanoformulation has been reported as an innovative and developing technology to overcome these limitations.
Methods
In the present study, the antibacterial, biofilm inhibitory, and anti-adherence activities of free curcumin, mesoporous silica nanoparticles (MSNs), and Cur-MSNs were assessed against S. mutans.
Results
The results showed that Cur-MSNs demonstrated more antibacterial effects against S. mutans than free curcumin and MSNs (p<0.05). The MICs spectrum of Cur-MSNs, free curcumin, and MSNs was 128 µg/mL, 512 µg/mL, and 1024 µg/mL, respectively. The biofilm inhibitory effect of Cur-MSNs was 32 times more than free curcumin (p<0.05). The bacteria adhesion after 12 hours in Cur-MSNs was significantly reduced compared with free curcumin and MSNs.
Conclusion
The antibacterial activity of curcumin increased by its nanoformulation. These results indicated that Cur-MSNs possess potential anti-cariogenic and anti-plaque properties, warranting further investigation through in vivo experiments and clinical studies.
1. INTRODUCTION
Streptococcus mutans inhabits the human oral cavity and is known as one of the main causes of caries [1, 2]. S. mutans attaches to surfaces and then colonizes the oral cavity, and creates biofilms [3]. Dental bacterial biofilm is a mass of biofilm-forming bacteria, and the bacterial pheno-type in the biofilm form is different from its planktonic form [4]. The dental biofilm bacteria are much less sensitive to adverse growth conditions, such as biocide agents and hydrodynamic shear forces [5, 6]. Curcumin is a natural, yellow-orange, low molecular weight polyphenol compound found in the root of the plant Curcuma longa, which is used in the management of diabetes, wound healing, and treatment of cardiovascular diseases [7-9].
Different investigations reported that curcumin is a potential herbal antibacterial agent against drug-resistant bacteria [10]. Furthermore, curcumin is effective against oral bacteria which is related to dental and oral diseases [11]. One study demonstrated that curcumin could prevent the adherence of S. mutans [10], while another reported that curcumin could inhibit the activity of sortase A in S. mutans, thereby exerting anti-adhesive effects that may contribute to the prevention of dental caries [12]. Moreover, blue light-activated curcumin can photoin-activate the planktonic form of S. mutans, but its effects on the biofilm form of S. mutans are weak [13]. For these reasons, this research aims to determine the potential application of curcumin as an herbal antimicrobial agent and highlights the importance of investigating how curcumin performs on dental and oral bacteria.
Curcumin is very safe for use in animals and humans and has low toxicity even at high doses [14]. However, its low bioavailability, poor stability, and low solubility, along with a short half-life, have limited the clinical usage of curcumin [15, 16]. To overcome these limitations, nano-formulation has been introduced as an advanced technology [17-20].
Curcumin nanomaterials have shown greater thera-peutic results than free curcumin in various in vitro and in vivo investigations. Drug delivery through nanoparticles can enhance the efficacy of curcumin in several diseases, especially infections [21-24].
Various recent research works have focused on the application of silica nanoparticles as carriers [25]. Proper-ties of inorganic silica, such as topology, surface area, and size, can be modified to generate distinct interactions with different types of biological systems [26]. Furthermore, mesoporous silica has advantageous properties, such as high pore volume, large surface area, and tunable pore morphological structures, which make it suitable for use as a nanocarrier [27]. In the case of encapsulating pro-drugs in organic nanoparticles, the rapid decomposition of the organic material leads to the fast release of the drugs. Mesoporous silica nanoparticles (MSNs) allow for the prolonged release of drugs compared to organic nano-particles because the drugs are trapped inside the nanopores [28, 29]. MSNs can load natural agents, such as curcumin, to exert significant antimicrobial actions [30, 31].
Despite progress in dental care, caries remain an important health concern. Sometimes, traditional agents often fail to eradicate bacteria, leading to persistent infections and resistance. Loading curcumin in MSNs can increase its delivery and efficacy against caries-causing bacteria. This study addresses the research gap by eva-luating the antibacterial efficacy of curcumin-containing MSNs against S. mutans, thereby providing a novel and effective strategy to improve dental health and combat caries. The present study aims to investigate the antibacterial effect and biofilm inhibition of curcumin-loaded mesoporous silica nanoparticles (Cur-MSNs) against S. mutans. In addition, it investigates the impact of Cur-MSNs on the adhesion of S. mutans to surfaces.
2. MATERIALS AND METHODS
2.1. The Antibacterial Activity
In our previous study, Cur-MSNs were prepared via a chemical precipitation technique and then were physico-chemically characterized [32]. For the preparation of Cur-MSNs, 1000 mg of MSNs powder (Temadkala, Iran) and curcumin (Sigma Aldrich, USA) (100 mg) were suspended in 50 mL of dimethyl sulfoxide (DMSO) via ultrasonication. Then, the prepared suspension was stirred for 24 h and centrifuged at 17,000 rpm for 30 min. The resulting Cur-MSNs precipitates were washed with ethanol and dried in an oven [32].
The average particle size of Cur-MSNs was 92 nm. The pattern of XRD showed that Cur-MSN had a mesoporous structure related to the MCM-41 family. TEM image demonstrated rod-shaped mesoporous nanoparticles. The curcumin loading efficiency in MSNs was 82% [32].
The antimicrobial effects of compounds and controls were studied on S. mutans ATCC 35668. All steps were repeated three times. The antibacterial effect of free curcumin and Cur-MSNs was assessed using the broth microdilution method to evaluate the minimum inhibitory concentration (MIC) according to the Clinical and Laboratory Standards Institute (CLSI) protocol [33]. Serial two-fold dilutions of free curcumin, MSNs, and Cur-MSNs were prepared in varying concentrations and added to a bacterial suspension (0.5 McFarland) in BHI broth, followed by incubation at 37°C for 24 hours. The control contained only broth medium inoculated with bacteria and was incubated for 24 hours at 37°C. The MIC value was considered the lowest concentration of Cur-MSNs, MSNs, and free curcumin, which completely inhibited S. mutans growth as detected by the naked eye.
2.2. Inhibitory Activity on Biofilm
The minimum biofilm inhibitory concentration (MBIC) was evaluated for MSNs, free curcumin, and Cur-MSNs. In the first step, 0.1 mL of bacteria suspension (0.5 McFarland) and 0.1 mL of TSB were added to the microplate wells. To stimulate biofilm production, TSB containing 0.25% glucose was added to the microplate and maintained in an incubator at 35 °C for 24 hours. Then, serial concentrations of MSNs, Cur-MSNs, and free curcumin were added and incubated for 24 hours at 35 °C. In the next step, the content of the wells was removed and washed with sterile water. The OD of the wells was read at a wavelength of 650 nm before and after incubation at 35°C for 6 h. The biofilm inhibitory concentration is defined as the lowest concentration of an antibacterial agent, which results in an OD650 nm difference of ≤10% from the mean OD of the two positive control wells [34].
2.3. Bacterial Attachment
The 0.5 MIC as sub-inhibitory concentrations were used to evaluate the inhibitory action of Cur-MSNs, MSNs, and free curcumin on biofilm formation. Fresh TSB (1:20) with and without the mentioned substances was used to dilute the overnight bacterial culture. Then, the bacterial sus-pension was added to the wells and incubated at 35 °C. After 24 hours, the content of the wells was removed, and the generated biofilms were rinsed with sterile water and methanol used for fixing them. The wells were then allowed to dry and stained with crystal violet solution. The 33% acetic acid was used for biofilm dissolution. Lastly, the OD of the wells was read at 492 nm. Wells containing TSB without bacteria were used as negative controls.
2.4. Statistical Analysis
One-way ANOVA and t-test were carried out to calculate the antibacterial and antibiofilm effects. The significance level of p<0.05 was considered. To assess the normality of data, we conducted normality tests on the antimicrobial effect data for each compound and control group (MSNs, curcumin, and Cur-MSNs). Specifically, we carried out the Shapiro-Wilk test due to its robustness for small sample sizes.
3. RESULTS
Cur-MSNs exhibited an antibacterial effect on S. mutans, which was more pronounced than free curcumin and MSNs (p<0.05) (Fig. 1). The MICs spectrum of MSNs, free curcumin, and Cur-MSNs was 1024 µg/mL, 512 µg/mL and 128 µg/mL, respectively.
The biofilm inhibitory effect of Cur-MSNs was 32 times greater than that of curcumin (p<0.05) (Fig. 2).
The adhesion of bacteria to surfaces was assessed to evaluate the effect of Cur-MSNs at sub-MIC levels (0.5 MIC). The adhesion of bacteria after 12 hours in Cur-MSNs was significantly reduced compared with free curcumin, MSNs, and control (grown of bacteria without any treatment) (Fig. 3).
In the present study, it was found that Cur-MSNs decreased attachment of S. mutans at sub-MIC levels (p<0.05).
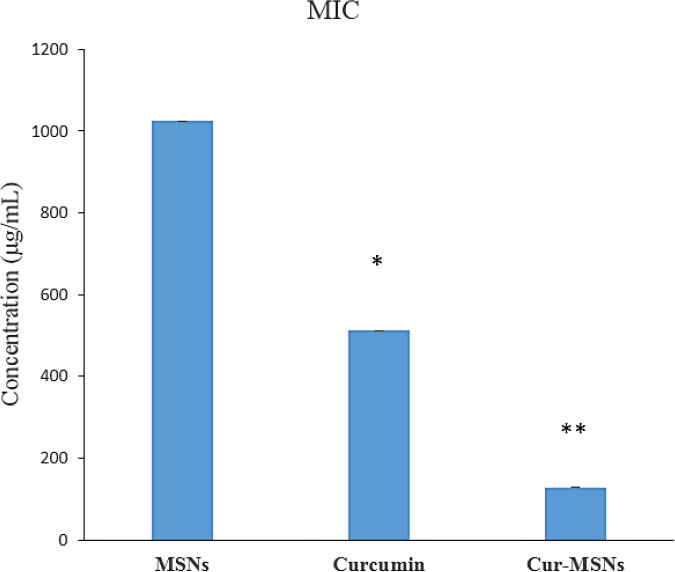
The inhibitory effects of MSNs, curcumin, and Cur-MSNs on S. mutans. (*) and (**) indicate p ≤0.05 compared with MSNs and curcumin, respectively.
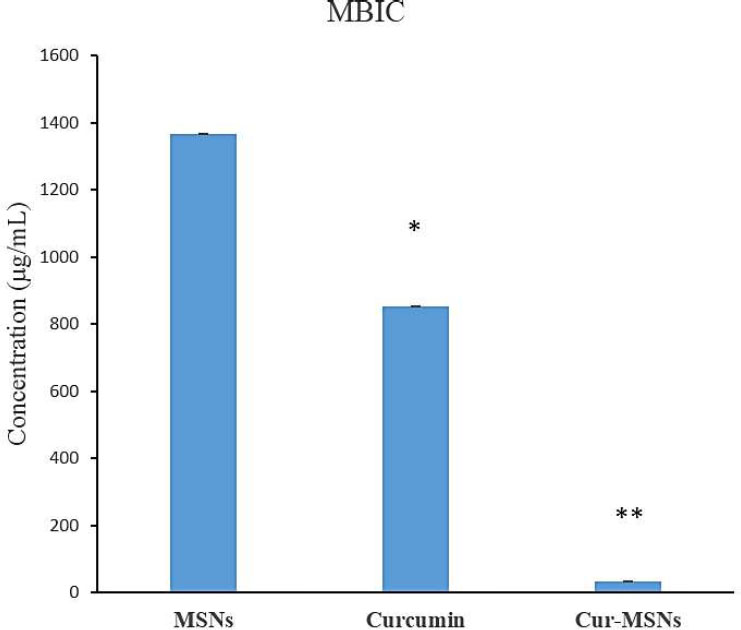
The bacterial biofilm inhibition of MSNs, free curcumin, and Cur-MSNs. (*) and (**) indicate p≤0.05 compared with MSNs and curcumin, respectively.
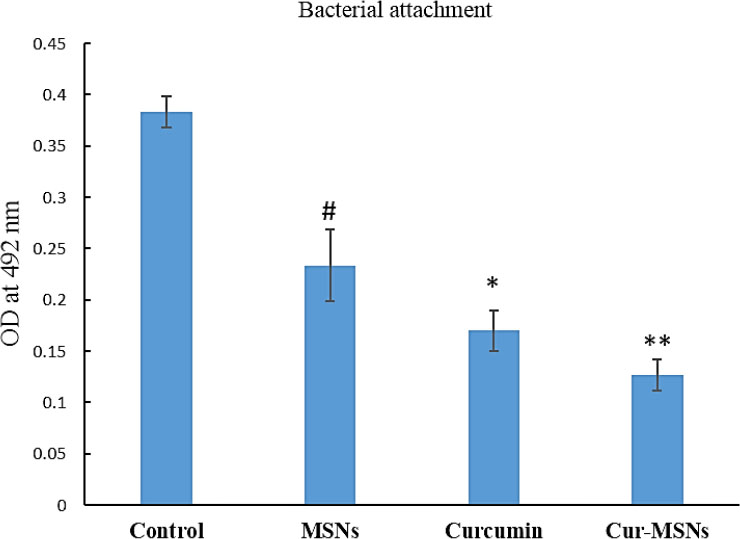
Effect of free curcumin, MSNs, and Cur-MSNs on the bacterial attachment to the plastic surface. (#), (*) and (**) and indicate p≤0.05 compared with control, MSNs and curcumin, respectively.
4. DISCUSSION
Nanoparticles containing drugs may interact with bacteria by two probable mechanisms: one, nanoparticles may integrate with bacterial cells' surface to raise the permeability to antibacterial agents, and the other, nanoparticles may be absorbed by the cell wall of bacteria and act as a reservoir for the antibacterial agent release [35].
Cur-MSNs exhibited an antibacterial effect on S. mutans, which was more significant than free curcumin and MSNs (p<0.05). The antibacterial effects of curcumin on gram-positive and gram-negative bacteria were also confirmed. Cheng et al. prepared core-shell magnetic MSNs bonded to intermittent mesoporous periodic organic silica with different loading properties. Curcumin and gentamicin were separately loaded into the hydrophobic and hydrophilic spaces, respectively, to create a dual-function antibacterial and anticancer nanoformulation [36].
A trio-hybrid nanocomposite of MSNs containing curcu-min and copper and decorated with nanoparticles of Ag showed the photokilling effect against E. coli [37]. In a study, a nanocomposite of curcumin-loaded MSNs and chito-san was produced and showed antibacterial activity against S. aureus and E. coli in the disc diffusion test [38].
Li and coworkers prepared a hybrid hemostatic organic-inorganic material via the electrospinning method by incorporating the MSNs containing curcumin into PVP nanofibers. They showed increased antibacterial effects by hybrid nanofibers against methicillin-resistant S. aureus (MRSA) in vitro [39].
Curcumin nanoformulations improved the antimicrobial effect of bulk curcumin against different microbes [40, 41]. Negahdari and coworkers prepared nanocrystals of curcumin and investigated their effects against Entero-coccus faecalis, S. aureus, and E. coli inside the implant fixture. Curcumin nanocrystals decreased bacterial colony-forming unit (CFU) significantly (p<0.01) over the incu-bation period [24]. Maleki Dizaj and coworkers evaluated the effect of curcumin nanocrystals against Porphyromonas gingivalis, which showed MBC of 12.5 μg/mL and MIC of 6.25 μg/mL [42].
Antibacterial drugs can affect the bacteria in planktonic form but may not be effective against the biofilm form of the same bacteria [43]. Sometimes, eradication of biofilm is difficult as a result of its antibacterial resistance. Removing the infected tissues or devices is critical for eradicating biofilm-associated infections that may be inconvenient or impossible in some cases. The usage of a suitable delivery system for antimicrobial agents can help eradicate biofilms [44]. Hence, the use of nanoformulations can increase the sensitivity of biofilms to antimicrobial agents [45]. In this study, curcumin and Cur-MSNs inhibited the biofilm formation of S. mutans (p<0.05). The biofilm inhibitory effect of Cur-MSNs was 32 times more pronounced than that of curcumin (p<0.05).
The attachment of bacteria is an important factor for their colonization on surfaces. In the present study, it was found that Cur-MSNs decreased attachment of S. mutans at sub-MIC levels (p<0.05).
Pamukçu et al. prepared curcumin-loaded hyper-branched polyethylenimine-grafted mesoporous silica nano-particles (F-MSN-PEI/Cur) and investigated their antibiofilm activity on Staphylococcus aureus biofilm. It was found that F-MSN-PEI/Cur inhibited biofilm formation and induced biofilm eradication [46].
Barros et al. synthesized curcumin-conjugated silica nano-particles, which were able to hinder the biofilm formation of model P. putida by up to 50% and disrupt mature biofilms by up to 54% [47].
CONCLUSION
Silica nanoparticles containing herbal agents with extraordinary advantages, such as cost-effective prepara-tion and exceptional biocompatibility, can be utilized as promising nanoformulations for the treatment of infec-tions. However, more evidence is required to prove the safety and therapeutic efficiency of MSNs, highlighting the need for extensive research before drug-loaded MSN systems can be translated to clinical applications. Critical evaluation of clinical data on side effects has reported that herbal drugs are usually better tolerated than synthetic drugs. Nevertheless, potentially serious complications, including plant interactions, have been described. This requires vigilance when using herbal medicines, especially in certain situations, such as the pediatric population and during pregnancy.
This study demonstrated the antibacterial effect of curcumin nanocrystals against S. mutants in vitro, which could have anti-caries and anti-plaque effects. However, the clinical use of Cur-MSNs may be challenged by issues, such as toxicity, stability in the oral environment, and scalability of the production process. To ensure the biocompatibility of these nanoparticles and their safe use in humans, it is necessary to conduct further additional studies.
AUTHORS' CONTRIBUTIONS
The authors confirm their contribution to the paper: S.S. and S.M.D.: Study conception and design; M.Y.M.: Data collection; M.Y.M., S.S., and S.M.D.: Analysis and interpretation of results; S.M. and M.Y.M.: Draft manuscript. All authors reviewed the results and approved the final version of the manuscript.
ETHICAL APPROVAL AND CONSENT TO PARTICIPATE
This study was approved (Ethical code: IR.TBZMED.DENTISTRY.REC.1403.044) by the Ethics Committee of the Tabriz University of Medical Sciences, Tabriz, Iran.
AVAILABILITY OF DATA AND MATERIALS
The datasets used and/or analyzed during the current study are available from the corresponding author upon reasonable request.
ACKNOWLEDGEMENTS
The authors would like to acknowledge the financial support provided by the Tabriz University of Medical Sciences (No. 75293).