All published articles of this journal are available on ScienceDirect.
The Use of 3D Model Printing for Acute Planning in Oral and Maxillofacial Traumatology
Abstract
Introduction
Due to the complex anatomy of craniomaxillofacial structures, facial reconstruction following high-impact traumas is extremely challenging. Therefore, this study aimed to evaluate the use of biomodels in the surgical planning of complex fractures and the sequelae in the oromaxillofacial region.
Materials and Methods
This is a cross-sectional, exploratory, descriptive, and quantitative documentary study. It was conducted at the “Dom Luiz Gonzaga Fernandes” Emergency and Trauma Hospital, in the hinterland of the state of Paraíba, in Brazil, based on cases recorded between November 2021 and November 2022. The research instrument utilized was a self-developed questionnaire.
Results
No statistically significant correlation was observed between the biomodel acquisition time and the length of hospitalization, with a Spearman's correlation coefficient of r = -0.079. Although the need for adjustment of osteosynthesis material was 50% in cases where acrylonitrile butadiene styrene (ABS) models were used, with p (1) = 0.464, in order to be significantly more faithful, it would require a study with a higher sample size. The average length of surgery, in minutes, was 91.25 ± 28.00. The average cost of the materials used to manufacture the biomodels, in Brazilian reais (R$), was R$122.00 ± R$89.09.
Conclusion
The use of biomodels in the surgical planning of complex fractures and oromaxillofacial sequelae did not increase the length of hospitalization. The material used for the prototype manufacture suggests a potential influence on its faithfulness. The length of surgery was shorter in interventions that utilized biomodels in the planning phase. It was also noted that the average cost of manufacture varies according to the type of material chosen and the quantity required.
1. INTRODUCTION
The complexity of craniofacial anatomy and the uniqueness of each defect require precise preoperative planning followed by highly individualized surgical treatment [1, 2]. Advanced imaging techniques have become essential components in reconstructive surgical planning. However, conventional forms, including three-dimensional (3D) re-constructions, are limited by their representation on two-dimensional (2D) computer monitors, which hinders physical interaction with the viewed reconstruction [2].
Thus, with the advent of additive manufacturing, it has become possible to rapidly generate physical models of patient anatomy for customized preoperative planning, using imaging techniques such as 3D computed tomo- graphy, magnetic resonance imaging, and ultrasound scanning [3, 4]. The applications in oral and maxillofacial surgery include the fabrication of personalised implants, intermediate splints, and prostheses for maxillofacial reconstruction, as well as supporting the entire surgical process. This process comprises preoperative diagnosis, planning and simulation, intraoperative navigation, and postoperative evaluation. Rapid prototyping technologies are also utilised to produce precise physical models that, when combined with 3D virtual models, aid in and enhance the teaching and learning process for medical students and inexperienced doctors. Additionally, these models help facilitate communication between surgeons and patients [3].
Although the applications of 3D printing in trauma surgery are underutilized, with a scarcity of scientific studies in the area and, primarily, the availability of case reports, its potential is significant. With 3D printing, specific anatomical models can be created in a variety of sizes and viewed and manipulated from different angles according to the specificity and individual needs of each patient. These models can enhance the understanding of complex fracture geometries and better represent topographic and functional relationships [5].
Among the applications of additive manufacturing in surgery of the oromaxillofacial complex, documented cases primarily involve orbital trauma and the middle third of the face, where reconstruction of the orbital contour and volume is challenging due to its extremely complex structure [6]. Recent technical advances have enabled surgeons to perform treatment with greater precision using the mirror-image technique, in which the software can mirror a healthy hemiface and print the intended bone structure, enabling the surgeon to plan the procedure from a prototype that precisely matches the programmed, ideal shape for the patient [7]. Other cases described in the literature involve complex mandibular fractures requiring the use of a reconstruction plate, the shaping of which, for adaptation to the bone contour, is more difficult, increasing intraoperative time. Alternatively, these reconstruction plates can be manually modelled preoperatively using the patient's mandibular biomodel, significantly reducing the operating time during surgery [8-11].
The objective of this study was to evaluate the use of biomodels in the surgical planning of complex fractures in the oromaxillofacial region and to discuss the existing literature on the topic. Although three-dimensional (3D) printing is widely used in oromaxillofacial surgery, its application is limited in the context of acute trauma due to significant delays caused by outsourcing.
Thus, the study aims to correlate the duration of hospital stay with the time required to acquire the biomodel, to describe the intraoperative time for surgeries where biomodels were used for pre-modelling plates and meshes, to correlate the need for adjustments during surgery with the type of material chosen for the biomodels, and report 3D printed biomodels.
2. MATERIALS AND METHODS
2.1. Ethical Considerations
This study was conducted in accordance with the Declaration of Helsinki for research involving human subjects, with all participants signing an informed consent form. It was submitted for review to the Ethics Committee of the Department of Health in the state of Paraíba, SES/PB, through Plataforma Brazil and observed the ethical principles proposed in National Health Council Resolution 466/2012, ensuring the confidentiality of participant data. It was approved under CAAE number 58848422.5.0000.5186.
2.2. Population and Sample
This study was conducted at the “Dom Luiz Gonzaga Fernandes” Emergency and Trauma Hospital, located in the city of Campina Grande, Paraíba. The study population consisted of patients admitted with scheduled elective surgery, monitored by the Oral and Maxillofacial Surgery and Traumatology (OMFST) specialty, between November 2021 and November 2022. A total of eight patients who met the study criteria were included, with anonymity preserved in accordance with ethical considerations.
2.3. Inclusion Criteria
All medical records of patients admitted and monitored by the CTBMF specialty at the Campina Grande Trauma Hospital were included in the study. These patients were treated with 2.4 mm titanium plates and/or conventional titanium meshes that were manually pre-moulded using biomodels in the planning of complex fractures in the oromaxillofacial complex. All patients meeting this criterion were included in the study, regardless of age or sex, while ensuring confidentiality and privacy and following ethical principles.
2.4. Data Collection
The research instrument was a form filled out by a single researcher using the data from patients’ medical records and a questionnaire obtained between November 2021 and November 2022. An Informed Consent Form (ICF) was obtained after the research participants or their legal guardians had been sufficiently informed of all possible benefits, risks, and procedures to be carried out and were provided with all pertinent information about the research.
Thus, the sample was obtained via convenience and in line with data availability, considering the need for production of the prototype and the availability of materials for its manufacture.
2.5. Statistical Analysis
The data were analysed descriptively using absolute and percentage frequencies for categorical variables and measures: mean, standard deviation (mean ± SD), minimum value, P25, median, P75, and maximum value for numerical variables. Fisher’s exact test was used to assess the association between two categorical variables (provided the condition for using the chi-square test was not met). To evaluate the association between two numerical variables, the Spearman correlation coefficient and Student’s t-test, specific to the null correlation hypothesis, were obtained. The margin of error used in statistical tests was set at 5%. The data were entered into an EXCEL spreadsheet, and IBM SPSS version 25 was used for statistical calculations.
3. RESULTS
In this study, the age of the patients ranged from 16 to 90 years, with a mean of 32.63, a standard deviation of 23.94, a median of 27.50 years, and 25 and 75 percentiles equal to 18.50 and 32.00 years, respectively. Table 1 presents the results related to the demographic and clinical profile, emphasizing that: five patients were aged between 20 and 90 years, and the other three were aged between 16 and 20 years; except for one female patient, all others were male; half of the patients were from Campina Grande, and the other four were from different municipalities; the most frequent aetiology was motor vehicle accidents with five patients, while the other 3 cases included physical assault, gunshot wounds (GSW), and falls, each occurring once; regarding the site of injury, 62.5% involved the mandibular symphysis, with frontal, orbit and mandibular angle types each occurring once; acrylonitrile butadiene styrene (ABS) material was the most frequently used in six patients, while grey resin was used in the remaining two; fused deposition modelling (FDM) printing was the most frequent type, observed in six patients, with stereolithography (SLA) printing used in the other two cases; the most frequent access types were submental with four cases and submandibular with two cases, while hemicoronal and subtarsal accesses occurred once each; the Lock system was used in six patients; no grafting was performed and there were no complications; adjustments were required in three patients.
Variable | n (%) |
---|---|
- | |
Total | 8 (100.0) |
Age group | - |
Up to 20 years | 3 (37.5) |
Over 20 years | 5 (62.5) |
Sex | - |
Male | 7 (87.5) |
Female | 1 (12.5) |
City | - |
Cacimba | 1 (12.5) |
Campina Grande | 4 (50.0) |
Jaçanã | 1 (12.5) |
Picuí | 1 (12.5) |
Santa Cruz | 1 (12.5) |
Aetiology | - |
Motorcycle accident | 5 (62.5) |
Physical assault | 1 (12.5) |
Gunshot wound (GSW) | 1 (12.5) |
Fall | 1 (12.5) |
Location | - |
Mandibular symphysis | 5 (62.5) |
Frontal | 1 (12.5) |
Orbit | 1 (12.5) |
Mandibular angle | 1 (12.5) |
Material type | - |
Gray resin | 2 (25.0) |
ABS | 6 (75.0) |
Printing type | - |
SLA | 2 (25.0) |
FDM | 6 (75.0) |
Access type | - |
Submental | 4 (50.0) |
Hemicoronal | 1 (12.5) |
Subtarsal | 1 (12.5) |
Submandibular | 2 (25.0) |
Material type | - |
Plate | 6 (75.0) |
Screen | 2 (25.0) |
System | - |
Lock | 6 (75.0) |
Not applicable | 2 (25.0) |
Graft | - |
Yes | - |
No | 8 (100.0) |
Complications | - |
Yes | - |
No | 8 (100.0) |
Adjustments | - |
Adjustments Yes |
3 (37.5) |
Yes | 3 (37.5) |
No | 5 (62.5) |
Variable | N | Mean ± SD | Min. | P25 | Median | P75 | Max. |
---|---|---|---|---|---|---|---|
- | - | - | - | - | - | - | - |
Age (years) | 8 | 32.63 ± 23.94 |
16.00 | 18.50 | 27.50 | 32.00 | 90.00 |
- | - | - | - | - | - | - | - |
Length of Hospitalization | 8 | 17.00 ± 11.67 |
8.00 | 9.00 | 9.50 | 29.00 | 37.00 |
- | - | - | - | - | - | - | - |
Acquisition Time (days) | 8 | 4.75 ± 1.39 | 3.00 | 3.25 | 5.00 | 6.00 | 6.00 |
- | - | - | - | - | - | - | - |
Cost (R$) | 8 | 122.00 ± 89.09 |
42.00 | 65.63 | 91.00 | 187.63 | 300.00 |
- | - | - | - | - | - | - | - |
Material Quantity (mL) | 8 | 208.87 ± 57.75 |
120 | 153.75 | 205.00 | 265.00 | 280 |
- | - | - | - | - | - | - | - |
Printing Time (hours) | 8 | 22.00 ± 8.96 | 9.00 | 14.00 | 21.50 | 29.75 | 35.00 |
- | - | - | - | - | - | - | - |
Surgical Time (minutes) | 8 | 91.25 ± 28.00 |
60.00 | 67.50 | 90.00 | 97.50 | 150.00 |
- | - | - | - | - | - | - | - |
Adjustment Time (minutes) | 3 | 23.33 ± 2.89 | 20.00 | 20.00 | 25.00 | 0.00 | 25.00 |
Table 2 exhibits the numerical variable statistics. Notably, the variability, expressed through standard deviation, decreased for the following variables: time of acquisition, quantity of material, surgical time, and adjustment time. Since this measurement was less than 1/3 of the corresponding averages, it was not high for the printing time variable (standard deviation less than half of the corresponding average) and was reasonably high for the other listed variables.
Table 3 displays the brightness coefficient between the acquisition time and length of hospitalization, a measure whose result was low and was not statistically different from zero.
Variable | Acquisition Time |
---|---|
r (p) | |
Length of Hospitalization | -0.079 (0.852) |
- | Adjusted | - | - | |
---|---|---|---|---|
Material type | Yes | No | Total Group | P-value |
n (%) | n (%) | n (%) | - | |
- | - | - | - | - |
Grey Resin | - | 2 (100.0) | 2 (100.0) |
p(1) = 0.464 |
- | - | - | - | - |
ABS | 3 (50.0) | 3 (50.0) | 6 (100.0) | - |
- | - | - | - | - |
Group total | 3 (37.5) | 5 (62.5) | 8 (100.0) | - |
- | - | - | - | - |
Table 4 displays the evaluation of the need for intraoperative adjustments according to the type of material used.
Although there is a large percentage difference in the cases requiring adjustment, for grey resin, the percentage is null, whereas for ABS, it is 50.0%. However, there was no significant association, which can be explained by the small sample size of this technique. With only two cases of grey resin and six cases of ABS, the percentages are not reliable measures, necessitating a study with a higher sample size to better corroborate this issue.
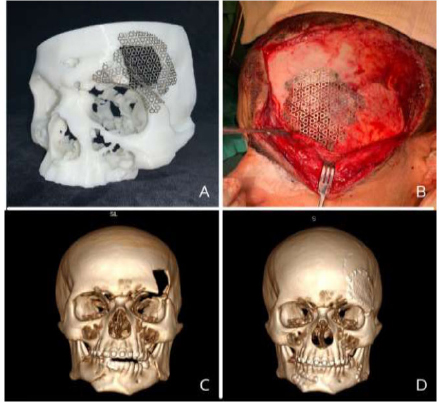
Pre-modelled titanium mesh under biomodel (A), Well-adapted and fixed titanium mesh under bone defect (B), 3D reconstruction of preoperative CT scan showing restoration of facial contour (C). 3D reconstruction of postoperative CT scan showing restoration of facial contour (D).
4. DISCUSSION
The use of 3D printed models in trauma surgery stemmed from the need to represent complex fracture patterns more realistically than those that are achieved through purely virtual representation on a computer screen. This approach addresses concerns, such as the lack of anatomical reference, adequate bone stock for reconstruction, challenges in restoring the original contour, and aesthetic considerations during facial reconstruction [5] (Fig. 1).
Additionally, in some cases, the use of load-bearing support systems is necessary, particularly in comminuted fractures. In such instances, the need to shape recons- truction plates intraoperatively further complicates an already complex surgery. Moreover, repeated bending may cause fatigue fractures, and inadequately adapted plates in the mandible may affect the position of the condyles and lead to primary reduction loss [12, 13]. (Fig. 2).
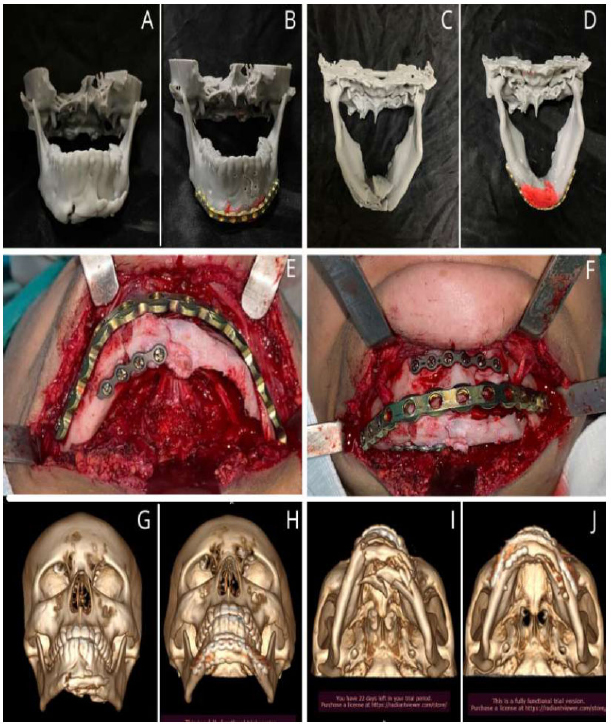
Patient biomodel, frontal and inferosuperior view (A and C), Biomodel with pre-moulded reconstruction plate after fracture reduction, frontal and inferosuperior view (B and D), Reduction and fixation of plates during surgery (E and F), Preoperative 3D CT scan reconstruction (G and I), Postoperative 3D CT scan reconstruction (H and J).
For orbital floor fractures, 3-D printing provides an accurate anatomical representation of the bone defect, allowing the clinician to preoperatively adopt a titanium mesh for orbital reconstruction. When the patient has a healthy orbit, it can also serve as a model for mirroring. This theoretically improves surgical outcomes by reducing operative time, decreasing the risk of malposition of the orbital mesh, and minimizing soft tissue trauma from multiple insertions during cutting and adaptation [14] (Fig. 3).
It is understandable, therefore, that operative time is often used by authors to assess this technology because it is an objective element, although this measure has rarely been evaluated or accurately measured [15]. In a series of four cases on the treatment of atrophic mandibular fractures using reconstruction plates, Brazil et al. (2021) reported an average length of surgery of 150 minutes. In the present study, we observed that the operative time generated a mean of 91.25 ± 28.00 minutes, a significantly lower value compared to similar cases reported in the literature. The shorter surgical time may be explained by the reduction in the time required to shape the osteosynthesis material over the bone defect. This is possible due to the opportunity for preoperative planning [16].
However, although CAD CAM options have existed for over a decade, there is still a gap in CAD CAM knowledge and its applicability to acute trauma. This is mainly due to delivery times of 1 to 2 weeks from the time of injury to plate delivery due to the various steps required for its manufacture [17]. In view of this, this study aimed to evaluate whether the prototype acquisition time would influence the patient's length of hospital stay, and we observed that the correlation coefficient between prototype acquisition time and length of hospitalization did not have a statistically significant value. In other words, the acquisition time of the prototypes had no influence on the length of hospital stay. This observation may be explained by the fact that the waiting time can often match the time required for the patient's readiness for elective surgical procedures, which necessitates regression of oedema, and systemic compensation, among other factors. The average length of hospital stay found in our study was approximately 9 days, which is consistent with the findings of Bergeron, Bonapace-Potvin, and Bergeron (2021), who reported an average of 8 days.
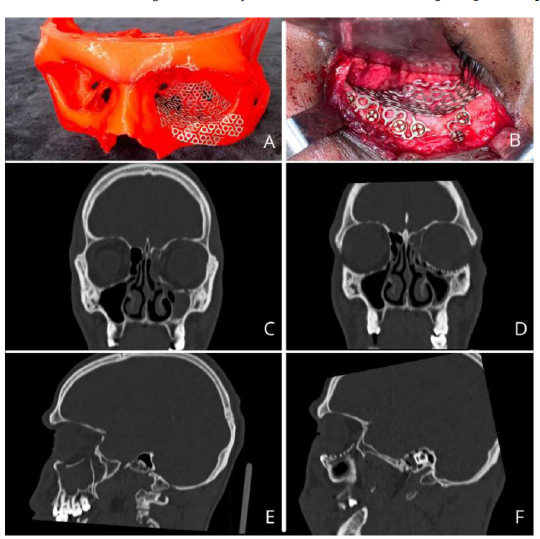
Pre-moulded titanium mesh in the mirrored orbit of the biomodel (A), Titanium mesh fixed intraoperatively (B), Preoperative CT scan in coronal and sagittal sections showing the fracture in the orbital floor (C and E), Postoperative CT scan in coronal and sagittal sections showing the adaptation of the mesh in the orbital floor (D and F).
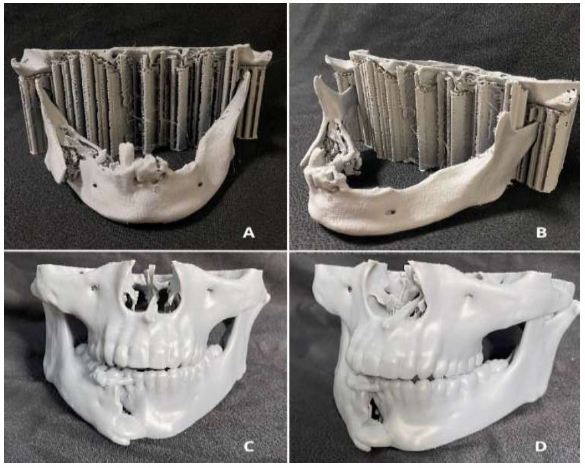
Patient's biomodel made of ABS (A and B), Patient's biomodel made of resin (C and D).
Several factors contribute to increased processing times. These include outsourcing the process, manually reducing the fracture on the printed biomodel, manually pre-modelling the material, waiting for sterilisation, and the type of material and printer used. It is worth noting that the lack of standardisation of the materials and printers used in this study may have introduced some bias. Elegbede et al. (2018), Marschall et al. (2019), and Alagarsamy et al. (2021) are among the studies that have highlighted virtual planning of fracture reduction and the printing of biomodels that already incorporate this reduction. This approach enables the printing of smaller volumes, as only the mandibular segment needs to be printed, given that condylar and occlusal references have already been checked virtually. Printing the biomodel with the reduced fracture allows for a shorter printing time, making it more cost-effective and providing better anatomical accuracy in fracture reduction [9, 12, 13].
This study also observed a lack of homogeneity in terms of the materials used. Although 3D printing techniques and the materials used are rarely described, they significantly impact the quality of the 3D printing device and, consequently, its effectiveness. Thus, this study proposed to investigate whether there is a correlation between the material used for printing the biomodel and the need for adjustments in the modelling of the osteosynthesis material during intraoperative procedures. In practice, ABS, the most frequently used material in the study, when worn down by the drill, is not good at withstanding overheating and melts, thereby losing the anatomical reference of the fractured margins. Therefore, we observed that, although the need for adjustment of the osteosynthesis material occurred in 50% of cases in which models made of ABS were used, at p(1) = 0.464, it would require a study with a higher sample size to better corroborate this issue. Therefore, in the future, it will be necessary to identify which materials and techniques for making the biomodels offer the best cost-benefit for each type of surgery and application. (Fig. 4).
Another limitation encountered in the study was the lack of raw material for the printing of the biomodels. Thus, although low-cost 3D printers are available, the additional costs associated with this technology continue to be a major limiting factor to its widespread use. The cost of 1 kg of filament is about 350.00 Brazilian reais, and 1 L of resin is about 1,500.00 Brazilian reais. The average cost in Brazilian reais of the material used for making the biomodels in this study was 122.00 reais ± 89.09 reais. However, it is worth noting that only 25% of the biomodels in this study were made using resin. Bergeron, Bonapace-Potvin, and Bergeron, in their studies conducted between 2021 and 2023, reported an average filament cost of $0.95 and $1.56, respectively. These prices are lower than those found in our study. Marschall, Oppenheim, and Kushner (2024) found that the average cost for 3D printing was $8.80, which is higher than the amounts reported by the aforementioned authors. However, this difference is due to the use of resin as the raw material, rather than filament. The variation in costs may also be attributed to the respective availability of raw materials in each country.
In summary, the use of three-dimensional (3D) printing in oromaxillofacial surgery for acute trauma is promising, but limited due to the long delays involved in obtaining commercially printed models. Until recently, 3D printing was primarily used for complex cases that could not be addressed using conventional methods. As a result, the literature consists predominantly of case reports. However, it should be noted that 3D printing has now become more accessible. Surgeons are now able to apply this technology to a greater number of patients, which opens up opportunities for research on the subject with greater academic value [18-22].
The study has its limitations regarding the difficulty of obtaining large samples. This is precisely because it is a convenience sample and depends on the production of biomodels, which requires specific equipment, specialized staff, and raw materials for manufacture, resulting in additional treatment costs.
CONCLUSION
It is recognized that trauma surgery prototypes are increasingly valuable for improving surgical planning and providing higher treatment quality, consequently enhancing patient comfort while ensuring the safety of the healthcare professional. Furthermore, their acquisition time was not found to be high, with an average of 4.75 ± 1.39 days, showing no increase in length of hospitalization. Moreover, the operating time was an average of 91.25 ± 28.00 minutes, significantly lower than surgical times reported in similar cases in the literature where biomodels were not used. However, the material used for making the prototype was a potential factor influencing the faithfulness of the biomodel. It is also worth noting that the average cost, in Brazilian reais, of the material used for making the biomodels in this study was 122.00 ± 89.09 reais; however, these values may vary depending on the type of material chosen and the quantity required.
AUTHORS’ CONTRIBUTIONS
It is hereby acknowledged that all authors have accepted responsibility for the manuscript's content and consented to its submission. They have meticulously reviewed all results and unanimously approved the final version of the manuscript.
LIST OF ABBREVIATIONS
ABS | = Acrylonitrile Butadiene Styrene |
R$ | = Brazilian Reais |
3D | = Three-dimensional |
2D | = Two-dimensional |
OMFST | = Oral and Maxillofacial Surgery and Traumatology |
GSW | = Gunshot Wound |
FDM | = Fused Deposition Modelling |
SLA | = Stereolithography |
CT | = Computed Tomography |
ETHICS APPROVAL AND CONSENT TO PARTICIPATE
The study was approved by the Research Ethics Committee at the Paraíba State Department of Health–(SES/PB), Brazil under protocol no. 58848422.5. 0000.5186.
HUMAN AND ANIMAL RIGHTS
All procedures performed in studies involving human participants were in accordance with the ethical standards of institutional and/or research committees and with the 1975 Declaration of Helsinki, as revised in 2013.
CONSENT FOR PUBLICATION
Informed consent was obtained from the patients/guardians for treatment and publication.
AVAILABILITY OF DATA AND MATERIALS
The data supporting the findings of this study are available from the corresponding author, [E.S.S.A], on specific request.