Mechanical Integrity of All-on-Four Dental Implant Systems: Finite Element Simulation of Material Properties of Zirconia, Titanium, and PEEK
Abstract
Background
Dental implants are critical for restoring functionality and aesthetics in patients with missing teeth. The all-on-four treatment concept utilizes four dental implants to support a full-arch prosthesis. Material choice for these implants plays a crucial role in the long-term success of the treatment, affecting everything from biomechanical stability to osseointegration and patient comfort.
Aim
The purpose of this study is to analyze the biomechanical performance of three different materials used in all-on-four dental implant designs through finite element analysis (FEA). The aim is to determine which material optimally balances stress and deformation under various loading conditions.
Objective
The main objective of this research is to evaluate the effects of stress, strain, and deformation on all-on-four dental implants made from titanium, zirconia, and polyether ether ketone (PEEK). The study seeks to identify which material demonstrates the best mechanical properties under simulated functional loads.
Methods
A 3D model simulating the dental implants integrated with cancellous and cortical bone was developed. Finite element analysis was conducted to assess the biomechanical performance of the implants made from titanium, zirconia, and PEEK. A perpendicular load of 100 N was applied to the tips of the implants, followed by an oblique load of 100 N at a 30-degree angle, to simulate different chewing forces.
Results
The deformation analysis indicated that implants made of zirconia exhibited significantly lower maximum and average deformation compared to those made from titanium and PEEK. Although PEEK implants showed lower maximum and average stress, they did not perform well in stress dissipation compared to zirconia. Similar patterns of stress and deformation were observed under both perpendicular and oblique loading conditions.
Conclusion
Zirconia implants outperformed titanium and PEEK in terms of deformation and stress distribution under simulated loading conditions. This suggests that zirconia could be a superior material for all-on-four dental implants, offering better mechanical stability and potentially enhancing the longevity and success of dental restorations. Further clinical trials are recommended to validate these findings and assess the long-term outcomes of zirconia-based implants.
1. INTRODUCTION
Many techniques have been used to monitor the performance of dental implants. One such method is hyperspectral imaging (HSI) where a spectrometer can be used to detect the biofilm contaminations on dental implants [1, 2]. Specifically, with the improvement in snap-shot-based HSI, which has been used in many other medical and other applications, dental implant performance analysis in the future will be much more convenient [3-9]. In recent years, many types of bio-sensors have also been developed [10-12]. One study that used a micro bio-sensor built inside the dental implant has already been proposed [13]. However, there are few studies on these methods, and all these methods can only be used after the dental implant has been fixed. Finite element analysis (FEA) has long been used to measure the effects of stress-strain and deformation in dental implants [14, 15]. In particular, the effectiveness of any dental implant is reliant on a wide range of variables that influence bone‒implant, implant‒abutment, and abutment‒prosthesis integrations [16, 17]. Examining the force distribution, including the stress-strain and deformation values, is essential in determining the success of any implant [18, 19]. One of the latest designs to overcome general failures is known as the “all-on-four” configuration, which uses only four implants, namely, two in the anterior region and two in the posterior region [20-22]. Many studies have performed FEA on all-on-four designs and proven its feasibility [23-27].
Another factor affecting the success of a dental impact is its material. Since the early 1960s, titanium has been used as a primary material for dental implants because of its economic viability, biocompatibility, extensive human clinical success, and bone bonding capacity [28-30]. Owing to aesthetics, many ceramic-based implants have been investigated in recent years [31, 32]. One such material is zirconia [33, 34]. Zirconia offers great resistance to oxidation, higher wear resistance, a high Weibull modulus, excellent flexural modulus, fracture toughness, and static fracture strength [35]. In addition, zirconia has a low thermal conductivity [36]. Although zirconia has exceptional short-term results, high-quality long-term studies are lacking [37]. Polyether ether ketone (PEEK) is a metal-free biocompatible thermoplastic polymer that is also currently being used in dental implants [38, 39]. PEEK has excellent mechanical qualities, high-temperature resistance, chemical stability, strong wear resistance, and high bond strength [40, 41]. However, PEEK has a much lower modulus of elasticity and low hardness [42, 43]. Comprehensive studies involving PEEK are also rare [44].
In our previous study, the effects of increasing the number of fences to support dental implants were investigated [45]. According to the results of the FEA analysis, 74% of the deformation and 80% of the stress occurred when the number of fences was increased from one to three. In general, as the number of fences increases, the stress dissipation results are enhanced. However, only titanium as a material for dental implants was considered in the study. In the current study, PEEK, zirconia, and titanium all-on-four dental implants were subject to a 100 N perpendicular load on four tips of dental implants. Then, the effects of stress-strain and deformation were investigated. An oblique load of 100 N at 30° was also applied, and comparative results were examined.
2. MATERIALS AND METHODS
2.1. Software
PEEK, zirconia, and titanium materials were compared for their deformation and strain‒stress results. Dassault Systemes' CATIA version 6 was utilized to construct the 3D model. The Finite Element Analysis (FEA) was conducted using Ansys version 2021 R2. The chosen design was simplified within limits that would not disrupt the local stress analysis, considering the intricate geometries of jawbones. The implemented design provides a foundation for conducting a comparative evaluation of three distinct materials and their corresponding deformation and strain-stress solutions, as derived from analytical investigations.
2.2. Design and FEA
The implant had a diameter of 4.5 mm and a total length of 8 mm. The radius of the bottom two fences was 2 mm, and the top fence had a diameter of 1.5 mm, following the configuration in our previous study 45. Given the dramatic shift in stress at the edges, the junctures were meshed three times as densely as the other locals. According to the classification system developed by Lekholm and Zarb, the model could represent a section of edentulous bone located in the posterior region of the mandible [46]. The bone that would be placed adjacent to the implant was 11 mm in height and 30 mm in width. The cortical bone, which had a thickness of 1 mm, was set to be in contact with the implant and jointly formed the top layer, whereas trabecular bone comprised the rest of the structure. The mesh had an average thickness of 0.05 µm, which resulted in 81,000 nodes and 27,000 elements.
2.3. Materials
As the sole purpose of this study was to compare the deformation and strain‒stress dissipation of the models, the jawbone designed for the analysis was assumed to be a homogeneous solid bone. This study also presumed that both the implant and the bone were isotropic, linearly elastic, and homogeneous. Then, the titanium, PEEK, and zirconia materials were compared. The properties of these three materials are listed in Table 1.
Material | Density (kg/cm3) | Young’s Modulus (GPa) | Poisson’s Ratio | Tensile Yield Strength (MPa) | Compressive Yield Strength (MPa) |
---|---|---|---|---|---|
Zirconia [47, 48] | 6.05 | 210 | 0.31 | 1000 | 2000 |
PEEK [49, 50] | 1.310 | 4 | 0.4 | 150 | 215 |
Titanium [51, 52] | 4.620 | 960 | 0.36 | 930 | 930 |
Cancellous Bone [53] | 2.08 | 71 | 0.3 | 280 | 280 |
Cortical Bone [54] | 1.2 | 2 | 0.3 | 112 | 112 |
Material | Minimum Deformation (µm) | Maximum Deformation (µm) | Average Deformation (µm) |
---|---|---|---|
Titanium | 0 | 1.7449 | 1.4594 |
Zirconia | 0 | 1.2673 | 0.19796 |
PEEK | 0 | 8.4035 | 5.5979 |
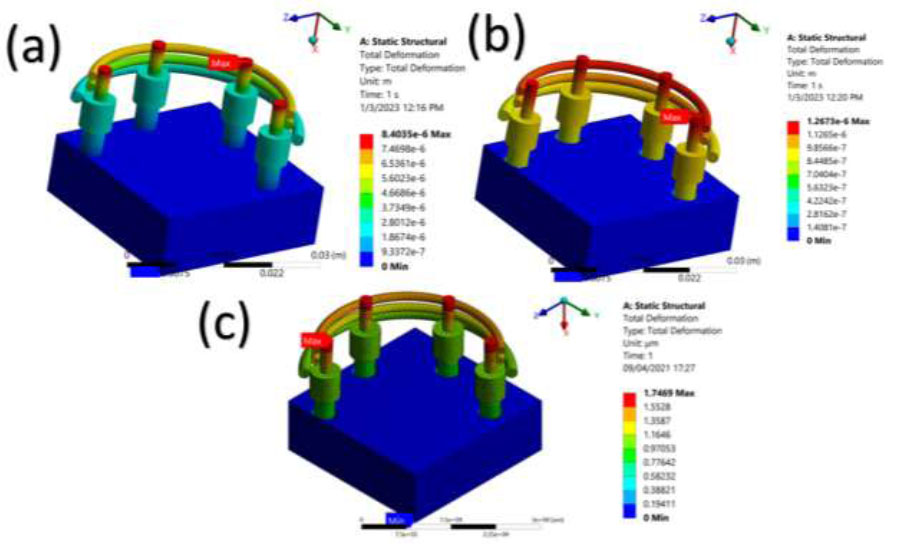
Total deformation of three different materials. (a, b and c) PEEK, zirconia, and titanium materials, respectively.
3. RESULTS
3.1. Deformation
The deformation results of the three materials are shown in Table 2, while Fig. (1) shows a comparison of the deformation results. A force of 100 N at the four tips of the dental implants was applied, totaling 400 N. The deformation results showed that zirconia had the lowest maximum deformation of 1.2673 µm, followed by titanium at 1.7449 µm. PEEK had the highest maximum deformation of 8.4035 µm. Although the maximum deformation results of titanium and zirconia were similar, the average deformation of the latter was much lower at 0.197 µm (titanium: 1.4594 µm). The obtained values indicate that zirconia was suitable for the three-fenced all-on-four implants and dissipated the deformation better than PEEK and titanium. Compared with the results of PEEK, the maximum total deformation of zirconia was reduced by 84.91% and compared with the results of titanium, the maximum total deformation of zirconia was reduced by 27.37%. This slight reduction in deformation over the years can increase the performance of the dental implant.
3.2. Stress
The results of the stress analysis (maximum, minimum, and average stresses) at 100 N for each tip of the dental implants for all three materials are shown in Table 3. A visual representation of the same results is shown in Fig. (2). The results showed that PEEK had the lowest maximum stress of 8.9674 MPa, whereas the highest maximum stress was for zirconia at 15.466 MPa. Titanium obtained a moderate maximum stress of 13.507 MPa. An interesting result was observed for the average stress. In particular, zirconia had the best average stress of 0.46083 MPa, whereas titanium and PEEK had average stresses of 4.5025 and 4.5528 MPa, respectively. The simulation results of the average stress indicate that although the maximum stress of zirconia is higher, its stress dissipation is much more efficient. The maximum stress of PEEK was much lower, while its average stress was much higher, indicating its poor stress dissipation capability. Effective stress dissipation can be calculated by comparing the average stress with the maximum stress. Here, PEEK had the poorest stress dissipation at 49.22%, whereas titanium had a better measure of 66.65%. Zirconia had the best stress dissipation measure at 97.0224%. These values indicate that zirconia can efficiently dissipate most of the stress.
3.3. Strain
The results of the strain analysis are shown in Table 4, in which a force of 100 N perpendicular to the dental implant was applied at the four tips. Fig. (3) shows the result of the strain analysis. Overall, the results of the strain analysis were similar to those of the stress analysis. Although PEEK had a lower strain, its average strain was much higher than those of zirconia and titanium. Although zirconia had a much higher strain rate, its average strain was the lowest among the three materials. Titanium had a moderate maximum strain and average strain.
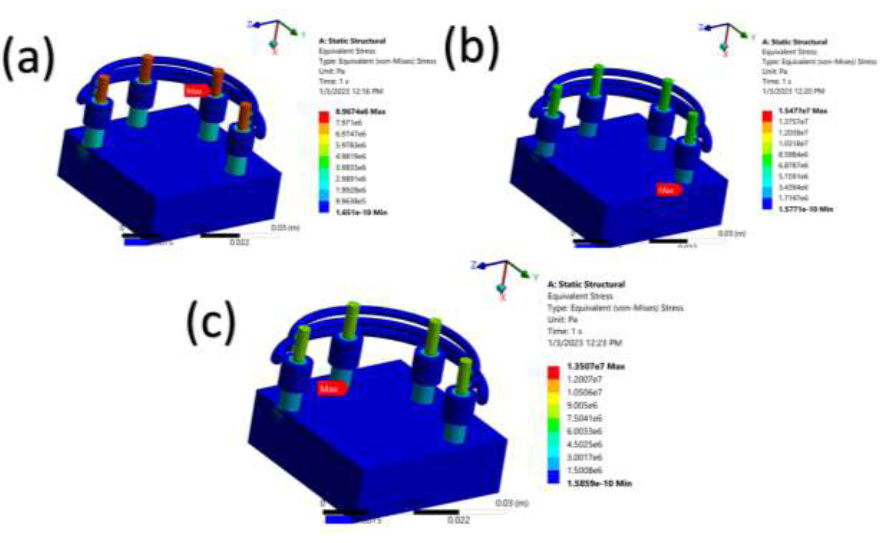
Equivalent von Mises stress of the three different materials. (a, b and c) PEEK, zirconia, and titanium materials, respectively.
Material | Minimum Stress (MPa) | Maximum Stress (MPa) | Average Stress (MPa) |
---|---|---|---|
Titanium | 1.5899×10−16 | 13.507 | 4.5025 |
Zirconia | 1.5772×10−16 | 15.477 | 0.46083 |
PEEK | 3.6469×10−16 | 8.9674 | 4.5528 |
Material | Minimum Strain | Maximum Strain | Average Strain |
---|---|---|---|
Titanium | 2.2346×10−21 | 2.9244×10−3 | 3.2493×10−5 |
Zirconia | 2.2205×10−21 | 2.986×10−3 | 8.9055×10−6 |
PEEK | 2.3261×10−21 | 2.6274×10−3 | 4.1713×10−5 |
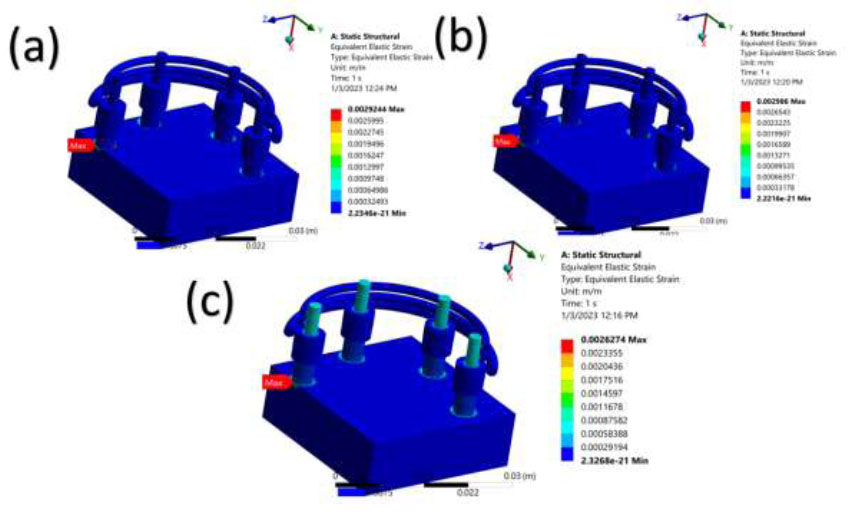
Equivalent von Mises strain of three different materials at a 30-degree oblique load of 100 N. (a, b and c) show titanium, zirconia, and PEEK materials, respectively.
3.4. Oblique Loading Analysis
Another factor considered in the FEA of dental implants is the process of mastication, also known as chewing. Here, the effect of an oblique load of 100 N, each at 30° on the four tips of the implant, was investigated. The deformation results of the oblique load of all three materials are shown in Table 5. A visual representation of the results is shown in Fig. (4). Overall, the results indicate that zirconia had a much lower maximum deformation and a lower average deformation, PEEK had the highest maximum deformation and a higher average deformation, and titanium performed reasonably.
The stress results of applying the oblique load on all three materials are shown in Table 6, and a visual representation of the results is shown in Fig. (5). The stress results of applying an oblique load of 100 N at 30° were similar to those under a perpendicular load. Zirconia had the maximum stress, whereas PEEK had a much lower stress value. The average stress of zirconia was much lower at 5.7217 MPa, whereas that of PEEK was 4.5 MPa. The stress dissipation of zirconia was 99.0644%, whereas that of PEEK was 98.1926%. The stress dissipation ratio of titanium was 98.805%. The maximum stress and average stress values were much lower for PEEK, whereas the stress dissipation measure was higher for zirconia.
Material | Minimum Deformation (µm) | Maximum Deformation (mm) | Average Deformation (µm) |
---|---|---|---|
Titanium | 0 | 0.27742 | 18.494 |
Zirconia | 0 | 0.20841 | 14.43 |
PEEK | 0 | 0.93911 | 45.893 |
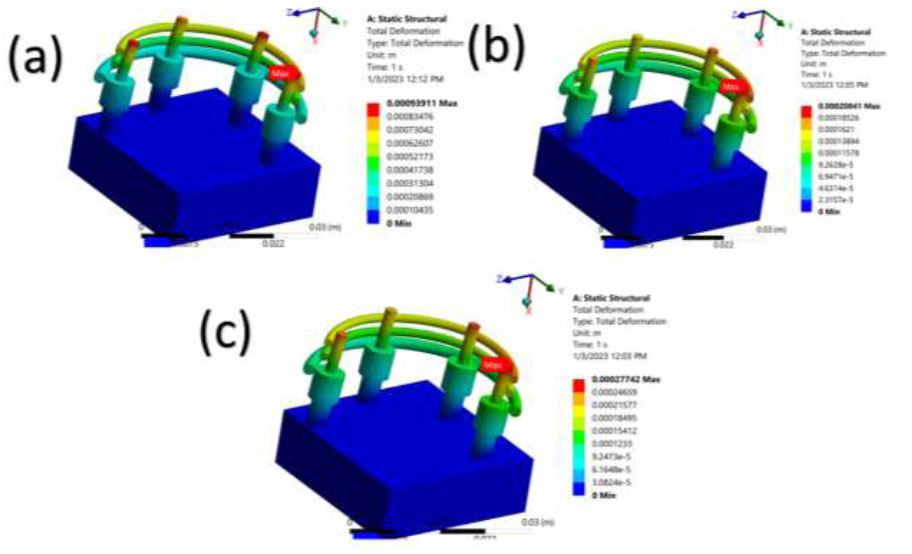
Total deformation of the three different materials at an oblique load of 30° of 100 N. (a, b and c) PEEK, zirconia, and titanium materials, respectively.
4. DISCUSSION
In this study, the FEA of three-fenced all-on-four dental implants was investigated based on three materials, namely, titanium, zirconia, and PEEK. The stress-strain and deformation results for a perpendicular load of 100 N applied at the four tips of the dental implant were obtained. An oblique load of 100 N at 30° was also applied on the four tips of the dental implant, and similar results were obtained. The deformation results showed that zirconia had less deformation than PEEK and titanium. However, the stress analysis showed that PEEK had a much lower maximum stress and average stress. The stress dissipation measure also showed that zirconia was much better at dissipating the stress to the surrounding fences.
Furthermore, deformation analysis showed that the maximum deformation was located at the tip of the dental implant, and a large amount of stress was found at the fences. Most of the higher stress for PEEK was found on the dental implant, whereas the maximum deformations for titanium and zirconia were found at the interjunction of the dental implant with the bone. The deformation of PEEK can cause an earlier failure of the dental implant because of the highly concentrated stress on the dental implants (Supplementary Material).
Material | Minimum Stress (MPa) | Maximum Stress (MPa) | Average Stress (MPa) |
---|---|---|---|
Titanium | 3.4808×10−16 | 422.6 | 5.048 |
Zirconia | 1.5772E-10 | 611.56 | 5.7217 |
PEEK | 3.6469E-10 | 248.99 | 4.5 |
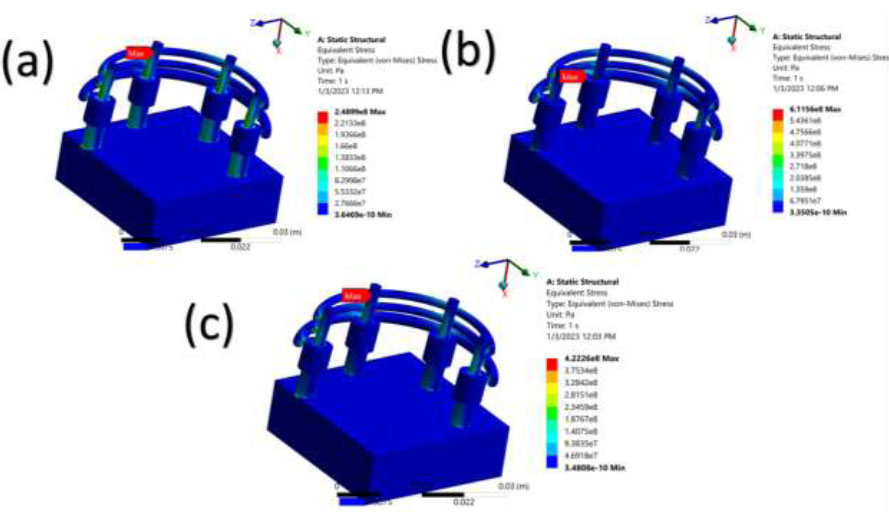
Equivalent von Mises stress of three different materials at a 30-degree oblique load of 100 N. (a, b and c) PEEK, zirconia, and titanium materials, respectively.
CONCLUSION
Titanium, zirconia, and PEEK materials were evaluated by FEA for a three-fenced all-on-four dental implant in this study. The stress-strain and deformation for a perpendicular load of 100 N applied at each of the four tips of the dental implant were studied. In addition, an oblique load of 100 N at 30° was applied on each of the four tips of the dental implant, and the same results were obtained. The results of the deformation test indicate that zirconia had a lower degree of deformation than PEEK and titanium. The stress study, on the other hand, revealed that PEEK had a significantly lower maximum stress and a significantly lower average stress. On the other hand, the stress dissipation result demonstrated that zirconia was significantly more effective for transferring stress to the fences in the surrounding area.
AUTHORS’ CONTRIBUTION
It is hereby acknowledged that all authors have accepted responsibility for the manuscript's content and consented to its submission. They have meticulously reviewed all results and unanimously approved the final version of the manuscript.
AVAILABILITY OF DATA AND MATERIALS
The data presented in this study are available in this article upon request to the corresponding author.
FUNDING
This research was financially supported by the National Science and Technology Council, the Republic of China (Taiwan), under grants NSTC 112-2221-E-194-036 and 112-2634-F-194-001. This work was financially/partially supported by the Kaohsiung Armed Forces General Hospital research project KAFGH_D_113030 and the Dalin Tzu Chi Hospital, Buddhist Tzu Chi Medical Foundation-National Chung Cheng University Joint Research Program in Taiwan.