All published articles of this journal are available on ScienceDirect.
The Effect of Gold Nanoparticles and Apricot Kernel Extract on the Osseointegration of Dental Implants - A Rabbit Model
Abstract
Introduction
The osseointegration of dental implants is critical to their longevity and success. Various factors such as surface modifications, both additive and subtractive, systemic drug administration, growth factors, and plasma derivatives can influence osseointegration.
Aims and Objectives
This study aimed to study the effects of gold nanoparticles and aqueous extract of apricot kernels on the osseointegration of titanium implants in the tibia of rabbits.
Materials and Methods
Apricot kernels were dried, grounded, and dissolved in water to obtain an aqueous extract. It was filtered and stored in the refrigerator at 4°C. Gold nanoparticles (AuNPs) were prepared by mixing 3 ml of 0.02 mM hydrogen tetrachloroaurate with 1 ml of olive leaf extract. The mixture was stirred vigorously for 15 minutes on a heater stirrer at 50°C. Titanium screw implants were sterilized in an autoclave at 120°C with 15 pounds per square inch for 30 minutes. Sterilized implants were stored in sealed containers until use.
The study was carried out at the College of Veterinary Medicine, University of Mosul, Iraq. The ethical approval for the study was obtained from the institutional animal care and use committee, College of Veterinary Medicine, University of Mosul, Mosul, Iraq, on 15/04/2023 on reference No. UM.VET.2023.013. Nine healthy male adult white New Zealand rabbits aged between 10-12 months were utilized for the study. Three pure titanium implants, each of a type, were placed in each tibia. The implants were categorized as follows: control group (18 uncoated implants) and experimental group (36 coated implants). The implant placement was divided into three groups: controls with uncoated implants (n = 18), AuNPs-coated implants (n = 18), and apricot kernel extract-coated implants (n = 18). General anesthesia was induced by intramuscular injection of ketamine hydrochloride 50mg (1ml /kg of body weight) plus xylazine 2% (0.2 ml /kg of body weight). The AuNPs-coated implants were implanted in the superior osteotomy site in the tibia. The apricot kernel extract-coated implants were implanted in the inferior osteotomy site and the uncoated implants were implanted in the middle osteotomy sites. Histological and histomorphometric tests were performed on all bone implant blocks at one-week, two-week, and three-week intervals after implant placement. The results were evaluated based on the amount of bone formation, cellular components, and inflammation. Tabulated data were statistically analyzed using SPSS v23.0 (IBM, Armonk, NY) using ANOVA with a statistical significance of p <0.05
Results
We observed a significant amount of osteogenesis with reduced inflammation in AuNPs-coated implants and apricot kernel extract-coated implants compared to untreated implants. The osteoclastic activity was significantly higher in untreated implants than the treated implant sites. A statistically significant association was observed between coated implants and untreated implants.
Conclusion
The application of gold nanoparticles and aqueous extract of apricot kernels appears to be promising in reducing inflammation and improving bone apposition on the titanium implant surface. However, this preliminary investigation warrants further research.
1. INTRODUCTION
The osseointegration process involves the direct structural and functional connection between living bone and the dental implant surface [1]. The choice of implant material also plays a crucial role in osseointegration [2]. Titanium and its alloys are widely used due to their biocompatibility and ability to achieve osseointegration [2, 3]. Surface modifications such as UV treatment, acid etching, bioactive surfaces, and incorporation of biological molecules have been shown to enhance osseointegration by altering the titanium dioxide on the surface [4-7]. The surface treatment of dental implants plays a crucial role in modifying the surface topography and energy, leading to improved wettability, improved cell migration, increased cell proliferation, increased cell attachment to the dental implant surface, and accelerated osseointegration processes [5]. The use of nanoparticles in dentistry, including gold nanoparticles, has been explored for various applications such as dental filling, reinforcement of dental implants, and prevention of caries [8]. While bulk gold is generally recognized as a benign and bioinert material with widespread applications in dentistry, the potential toxicity of gold nanostructures is still under investigation [9]. However, studies have confirmed the low cytotoxicity of cell labeling with gold nanoparticles in vitro, indicating their potential biocompatibility in dental applications [10]. Gold nanoparticles have been found to inhibit matrix metalloproteases without cytotoxicity, indicating their possible role in promoting a favorable environment for osseointegration [11]. Additionally, in a murine study, chitosan-gold nanoparticles have been shown to support osseointegration, even in osteoporotic conditions [12]. Park et al. 2017, highlighted the lack of rapid osseointegration and bone differentiation promotion by currently available implant surface treatments and suggested the need for further research in this area [13]. Hence, the application of gold nanoparticles on dental implant surfaces holds promise for enhancing osseointegration. While the biocompatibility and potential toxicity of gold nanoparticles are still under investigation, their low cytotoxicity and potential for gene delivery to promote osseointegration suggest their potential utility in dental implantology [12, 13].
Prunus armeniaca L., commonly known as the apricot, is a species of Prunus native to Central Asia and widely cultivated in many countries for its fruit [14]. The specific epithet “armeniaca” refers to the belief that apricots were first cultivated in Armenia, although their actual origin is still a matter of debate among botanists and historians [14]. The apricot tree is a small to medium-sized tree, reaching heights of 8–12 m, with a trunk up to 40 cm in diameter. The fruit of Prunus armeniaca L. is a drupe, typically 4–8 cm in diameter, with a smooth or velvety skin and a single seed enclosed within a hard shell and ripens from July to August [15]. Apricots are valued for their sweet and tangy flavor, as well as their nutritional benefits, and are consumed in various forms. They are a good source of vitamins A and C, as well as dietary fiber and antioxidants [14-16]. Prunus armeniaca L. has also been of interest in traditional medicine systems for its potential health benefits [17]. Various parts of the apricot tree, including the fruit, leaves, and kernels, have been used in traditional remedies for conditions such as constipation, cough, and skin disorders [18].
Ongoing research into the genetic diversity, cultivation practices, and phytochemical composition of apricots continues to enhance our understanding of this important species. Apricot kernels are known to contain various bioactive compounds, including proteins, antioxidants, polyphenols, amygdalin, and fatty acids [19-23]. The presence of amygdalin in apricot kernels has been a subject of interest due to its pharmacological effects, including hepatoprotective and anticancer activities [24]. Additionally, the high content of proteins and antioxidant molecules in apricot kernels makes them a valuable source of bioactive compounds [19, 25]. Apricot kernel oil has also been found to be rich in unsaturated fatty acids, tocopherols, beta-carotene, and citrostadienol, further contributing to its bioactive properties [23, 26].
To enhance osseointegration, it is imperative to explore newer bioactive molecules that can be utilized in conjunction with titanium and other metal implants. The quest for such molecules is driven by the potential to improve the interface between the bone and implant, thereby promoting the formation of excellent osseo-integration [27, 28]. In the context of traditional therapies, dietary components have been commonly used for wound healing and skin regeneration, particularly in Asian countries such as China and India [29]. This highlights the historical significance of natural sources of bioactive compounds in promoting healing and regeneration. Thus, apricot extract was selected to be used based on the historical significance of using natural sources of bioactive compounds to promote healing and regeneration.
The osseointegration of dental implants is influenced by various factors, including surface modifications, materials, medications, and medical conditions [23, 26-28]. While certain treatments and materials have been shown to enhance osseointegration, it is crucial to consider potential negative impacts and explore strategies to improve osseointegration in challenging conditions. Therefore, this study is a preliminary attempt to compare and study the effect of gold nanoparticles and apricot kernel extract (Prunus armeniaca L.) on the osseo-integration of dental implants in an animal model.
2. MATERIALS AND METHODS
2.1. Apricot Kernel Extract Preparation
Apricot seeds were procured from the local Iraqi market from July to Aug 2023. They were then dried under sunlight and ground by using a grinder (Fig. 1). 15 gm of ground kernels were dissolved in 150 ml of distilled water using the maceration method for 48 hours to obtain an aqueous extract (Fig. 1). The solution was filtered using Whatman® filter paper (Whatman No-1, UK), and the procured extract was then stored in the refrigerator (Fig. 1) for further use.
2.2. Gold Nanoparticles Preparation
0.3 ml of Au nanoparticles (Fig. 2) diluted in 10 ml of distal water was used.
2.2.1. Preparation of Au-NPs
A typical method for synthesizing gold nanoparticles (AuNPs) involves mixing 3 mL of 0.02 mM hydrogen tetrachloroaurate (III) (HAuCl4.4H2O, 99.99%) (Sigma-Aldrich, USA) with 1 mL of olive leaf extract in a test tube and stirring vigorously for 15 minutes at 50 °C on a heater stirrer. The change in color to dark yellow is a good indication of the formation of AuNPs [12, 14].
2.3. Implant Preparation
Readymade titanium screw implants of 1.5 mm width and 8 mm length (TAD Mini Orthodontic implants, China) were used in this study. They were sterilized in an autoclave at 120°C and 15 bar/cm for 30 minutes and placed in sealed containers until use.
2.4. Experimental Animal Description
The ethical approval for the study was obtained from the institutional animal care and use committee, College of Veterinary Medicine, University of Mosul, Mosul, Iraq, on 15/04/2023 with reference no UM.VET.2023.013. Nine male adult white New Zealand healthy rabbits were used in this study. Rabbits were purchased from the animal house of the College of Veterinary Medicine / University of Mosul, India. They aged between 10-12 months, thus assuring the complete closure of the proximal tibial epiphysis. Their weight ranged between 2-2.5 kg; they were kept in standardized separate cages, were fed with berseem (Trifolium alexandrinum), and had free access to tap water. Before surgical procedures, all animals were allowed to acclimate to the new environment for seven days. The animals were kept well fed, cared for, and under the supervision of a team of competent veterinary staff.
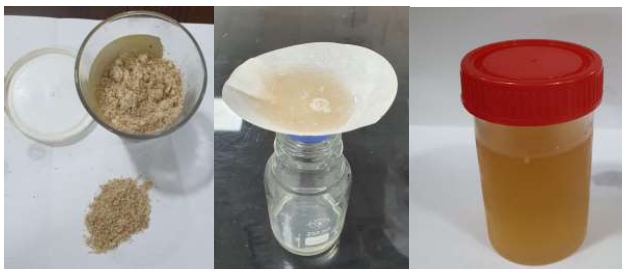
Preparation of apricot kernel extract - ground kernel, - filtration of the aqueous mixture of grounded apricot kernels, - aqueous extract of apricot kernels.
2.5. Sample Grouping
The animals were divided into three groups according to the time of sacrifice as follows: 1, 2, and 3 weeks after implantation (3 rabbits were sacrificed for each interval). Three pure Titanium (cpTi) implants were placed in each tibia (six implants in each rabbit).
The implants (Fig. 3) were categorized as follows:
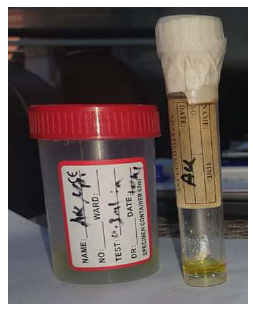
Gold nanoparticles.
2.5.1. Control Group (6 uncoated implants)
This group includes two implants for each healing interval (1,2 and 3 weeks).
2.5.2. Experimental Group (36 coated implants)
The experimental group was divided according to the coating material applied using a Pasteur pipette (one drop) into:
○ Implants coated with (one drop = 0.3 ml) Au nanoparticles.
○ Implants were coated with (one drop = 0.3 ml) apricot kernel extract solution during each healing interval).
Histological and histomorphometric tests were performed on all bone implant blocks for both control and experimental groups.
2.6. Surgical Implantation Procedure
Nine male adult white New Zealand healthy rabbits were used in this study. Each animal was weighed to determine the required dose of anesthesia. All instruments and towels used during the procedure were autoclaved at 120°C and 15 bar/cm for 30 minutes. General anesthesia was induced by intramuscular injection of ketamine hydrochloride 50mg (1ml /kg of body weight) plus xylazine 2% (0.2 ml /kg of body weight).
The coated implants with gold nanoparticles were implanted in the superior osteotomy site in the tibia. The implants coated with an aqueous solution of apricot kernel extract were implanted in the inferior osteotomy site, and the uncoated implants were implanted in the middle osteotomy sites.
Both tibiae were shaved on the ventral side (Fig. 4), and the skin was cleaned with a mixture of ethanol and iodine. Then, a piece of gauze damped with alcohol was left covering the shaved skin for 10 minutes. Surgery was performed gently under sterile conditions with the proper surgical technique. The gauze was removed, and the surgical towels were placed around the site of the operation. The proximal tibia metaphase was exposed after skin incision and reflection of the fascia and periosteal flap (Fig. 5). Bone preparation was performed by intermittent drilling under continuous cooling with normal saline irrigation. A physio-dispenser, along with its bur set at a rotary speed at 2500 rpm and a reduction ratio of 20/1, was used. The opening was made by a 1mm round bur, and then three holes were drilled with a 10 mm distance between them. The osteotomy was conducted- gradually with theappropriate drill. The operation site was washed with normal saline to remove the debris from the drilling sites (Fig. 6). The sterilized implants were placed in the prepared osteotomy sites. The insertion of the uncoated one was directlyperformed, while the insertion of the coated implants was performed after the application of experimental materials inside the holes using pasture pipettes (Fig. 7).
Then, the implants were placed and threaded in the osteotomies (Fig. 8) by using the appropriate screwdriver until the implants were seated properly (Fig. 9) into the prepared sites. Following this, the area was washed with saline, and the muscles were sutured with an absorbable 3-0 chromic catgut suture (Fig. 10). (Catgut suture, MAI Animal Health, USA). The skin was then sutured using 3-0 black silk sutures (Fig. 11) (Silk Sutures, Fisher Scientific, USA). The sutured site was then dressed with local antibiotic spray (Oxytetracycline spray). (Terramycin Wound Spray, Zoetis, USA).
Postoperative care was taken appropriately. Long-acting systemic antibiotic Oxytetracycline (0.7 ml/kg) was administered to the animals after surgery. Postoperative care was performed by giving an antibiotic (local oxytetracycline spray 2.5%) & systemic IM) once daily for 5 days after surgery.
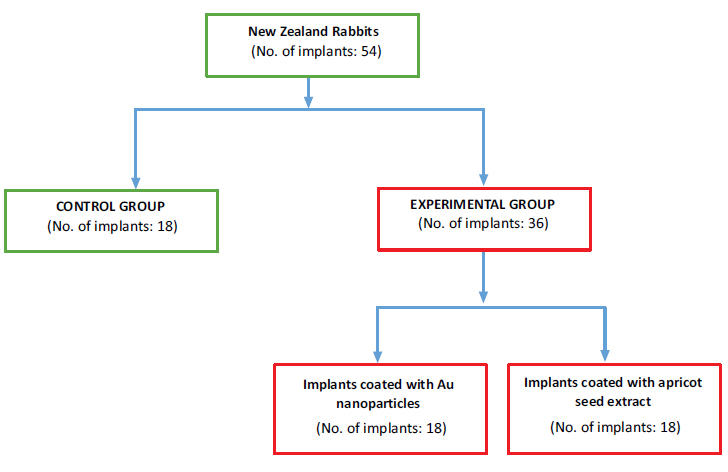
Distribution of the samples.
After the sacrificing of the animals, the tibia was dissected. The implant site was exposed, and the soft tissue was stripped. The implants were identified and separated from each other by cuts made by a carbide disc attached to the straight handpiece. A disc cutter with a low rotating speed and vigorous cooling was used to cut the bone around the implants. The osteotomy was made 5mm away from the head of the implant. Bone–implant block specimens were immediately stored in 10% freshly prepared formalin and left for 3 days for fixation. The specimens were left in 50% formic acid.
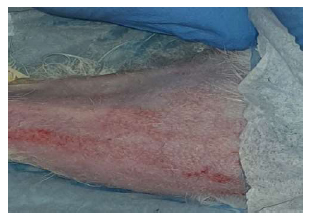
Shaved skin of the experimental animal.
The specimens were checked periodically using a fine needle. The solution was changed every 3-4 days. Once complete, decalcification was achieved, which was noted with the penetration of the needle deep into the specimen- deep without resistance. The bone implant block was then divided into two parts using a sharp scalpel along the entire length of the implant inside the bone and deep until the bone was divided into nearly two halves, one of them containing the implant. Then the implant was removed gently from its bed. Then, the specimens were put under running water for half an hour to remove the remaining acid, and then histological slides were prepared for histological and histomorphometric assessment.
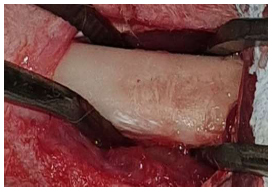
Reflection of skin and fascia.
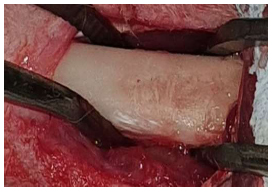
Bone bed preparation for implants.
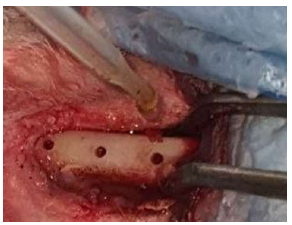
Application of experimental materials using a pipette.
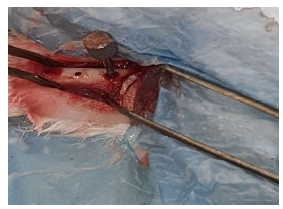
Placement of implant.
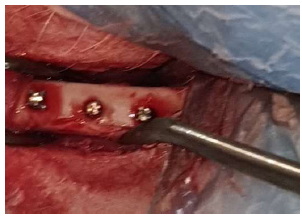
Implants in position.
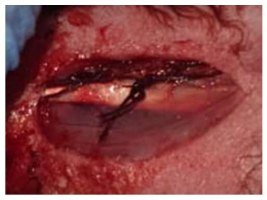
Muscles suturing.
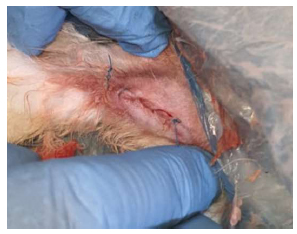
Skin suturing.
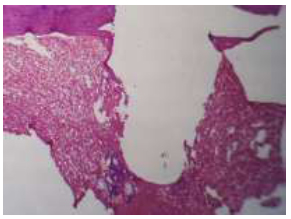
Illustrates the socket of the (A-coated) group in 1-week intervals followed by the screw shape H&E 4x.
3. RESULTS
3.1. Results after One Week
3.1.1. Apricot-coated Implant Group (A-coated)
After one week of implantation, the sample showed woven bone that followed the screw shape. The marrow space showed fibro-reticular tissue along with adipocytes and progenitor cells. In higher magnification, new bone formation was observed in the thread area of the implant, with numerous osteocytes within the lacunae. Few osteoclasts were also evident (Figs. 12, 13).
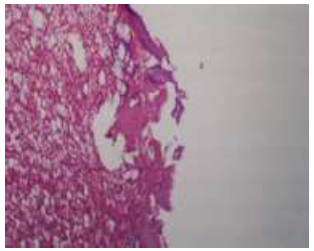
The view for the (A-coated) group illustrates woven bone in the thread area, the marrow tissue filled with fat, progenitor cells, and fibroreticular tissue, H&E X10.
3.1.2. Gold Nanoparticle Coated Implant (Au-coated)
The threaded area of the Au-coated implant has a large number of newly formed woven bone, fibroreticular tissue, and a low number of fatty cells. In higher magnification, bone trabeculae appeared, and the osteoblast cells arranged at the periphery of these trabeculae, a large number of osteocytes were embedded within the newly formed bone (Figs. 14, 15).
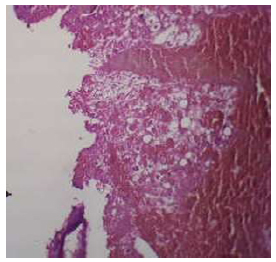
Illustrates the socket of (Au-coated) group in 1-week intervals, shows the threads following the screw shape H&E X4.
3.1.3. Control (uncoated group)
Threads in the marrow tissue area showed a large number of fatty cells and fibroreticular tissue with a large number of blood vessels. Higher magnification of thread area showed a large number of progenitors and fat cells (Fig. 16).
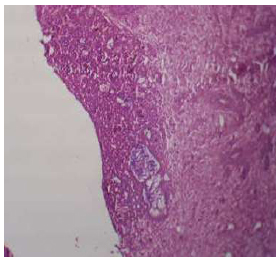
Shows the view for (Au-coated) group shows Woven bone was an Au-coated implant with fibroreticular tissue and fatty cells, H&E X4.
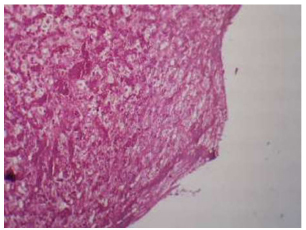
Illustrates the control group in 1 week shows a large number of fatty cells and fibroreticular tissue with a large number of blood vessels, H& E x10.
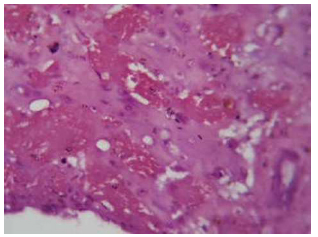
Illustrates the (A-coated) group in 2 weeks intervals showing bone trabeculae and fibroreticular tissue, osteocytes, and osteoblasts with large blood vessels H&E X40.
3.2. Results after Two Weeks
3.2.1. Apricot Coated Group
After two weeks of implantation, samples showed threads similar to the screw shape along with bony trabeculae, fibroreticular tissue, numerous osteocytes, osteoblasts, and a few osteoclasts. (Fig. 17).
3.2.2. Au-coated Group
The samples showed a bone formation that almost filled the region between threads of the implant. It had numerous osteocytes, osteoblasts, and osteoclasts along with fibroreticular tissue (Figs. 18, 19).
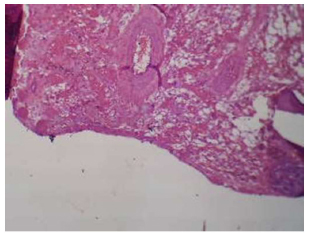
Illustrates of (A-coated) group in 2 weeks intervals shows bone trabeculae and fibroreticular tissue H&E X10.
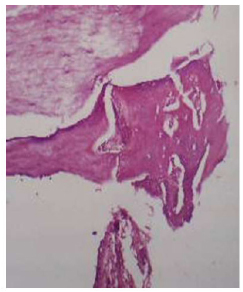
View illustrates the (Au-coated) group in 2 weeks intervals showing bone thread, osteocytes, and osteoblasts. H&E X10.
3.3. Results after Three Weeks
3.3.1. Apricot Coated Group
Microphotograph view of the bone section related to A-coated implants after 3 weeks of implantation showed thick bone trabeculae with fibroreticular tissue and a large number of osteocytes embedded within these trabeculae, a row of osteoblasts and osteoclasts.
3.3.2. Au-coated Group
Threads in the compact area show thick mature bone trabeculae, while the marrow tissue area showed more bone trabeculae with fibroreticular tissue in between (Fig. 20).
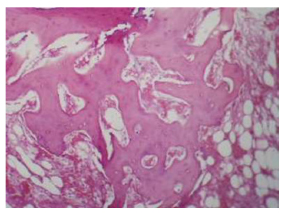
Illustrates the (Au-coated) group in 3 weeks interval thread of the implant showing thick bone trabeculae, fibroreticular tissue, osteocytes, and osteoblast cells. H& E40.
3.3.3. Control
Threads in the marrow tissue area showed a large number of fatty cells and fibroreticular tissue with a large number of blood vessels. Higher magnification of thread area showed a large number of progenitors and fat cells (Fig. 21).
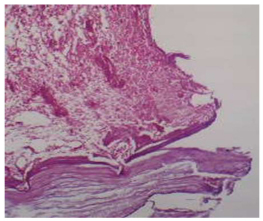
View illustrates the (uncoated) group in 3 weeks intervals, threads of the implant showing fatty cells, fibroreticular tissue, and blood vessels, H& Ex10.
The statistical comparisons among the study groups of the parameters are shown in Tables 1 and 2 and Figs. (22, 23, and 24).
Groups | Times | Inflammatory Cells | Blood Vessels | Osteoblast | Osteoclast |
---|---|---|---|---|---|
Control | Week 1 | 9.5 (1.78) | 8.5 (1.08) | 0.5 (0.71) | 2.5 (1.65) |
Week 2 | 2.3 (1.57) | 3.1 (0..88) | 5.5 (1.35) | 17.4 (5.30) | |
Week 3 | 2.5 (1.08)) | 3.7 (1.89) | 2.1 (1.85) | 19.6 (7.18) | |
Apricot | Week 1 | 3.5 (1.08) | 3.0 (1.05) | 0.6 (0.84) | 9.5 (44.93) |
Week 2 | 0.9 (0.77) | 5.1 (1.60) | 2.7 (2.45) | 21.0 (6.90) | |
Week 3 | 0.6 (0.84) | 2.9 (0.74) | 12.8 (4.16) | 21.9 (1.73) | |
Nano-Gold | Week 1 | 3.9 (2.69) | 3.6 (1.35) | 11.6 (8.04) | 24.4 (6.37) |
Week 2 | 3.6 (1.78) | 3.3 (1.70) | 13.8 (3.16) | 19.5 (2.27) | |
Week 3 | 1.0 (0.82) | 6.2 (1.96) | 23.4 (1.78) | 13.5 (2.12) |
Times | Groups | Inflammatory Cells | Blood Vessels | Osteoblast | Osteoclast |
---|---|---|---|---|---|
Week 1 | Control vs Apricot | 0.000 | 0.000 | 0..970 | 0.007 |
Control vs Nano-Gold | 0.000 | 0.000 | 0.000 | 0.000 | |
Apricot vs Nano-Gold | 0.941 | 0.494 | 0.000 | 0.000 | |
Week 2 | Control vs Apricot | 0.092 | 0.021 | 0.410 | 0.284 |
Control vs Nano-Gold | 0.124 | 0.948 | 0.000 | 0.642 | |
Apricot vs Nano-Gold | 0.001 | 0.025 | 0.000 | 0.796 | |
Week 3 | Control vs Apricot | 0.000 | 0.478 | 0.000 | 0.487 |
Control vs Nano-Gold | 0.003 | 0.003 | 0.000 | 0.013 | |
Apricot vs. Nano-Gold | 0.601 | 0.000 | 0.000 | 0.001 |
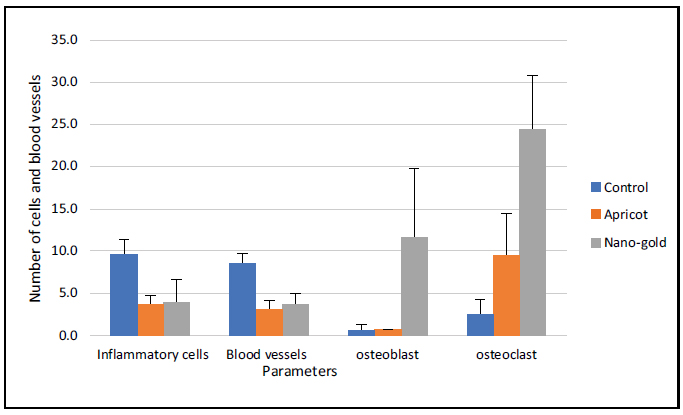
Bar chart showing the mean and SD of the number of cells for the tested groups after one week.
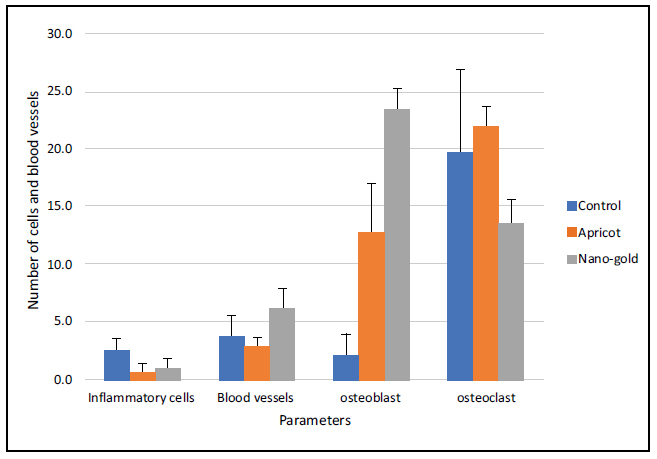
Bar chart showing the Mean and SD of the Number of cells in the tested groups after two weeks.
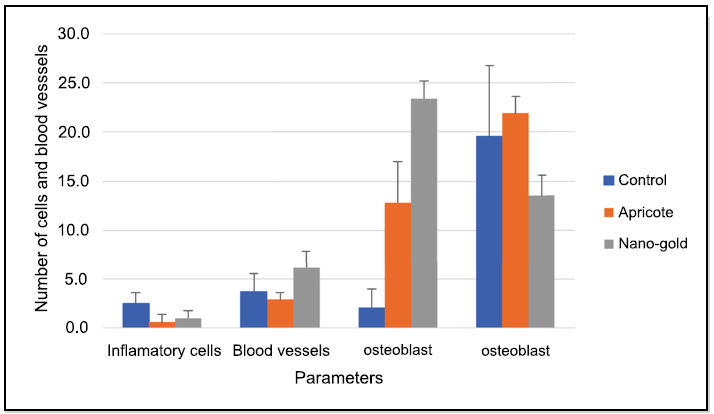
Bar chart showing the Mean and SD of the Number of cells in the tested groups after three weeks.
4. DISCUSSION
Orthopedic and dental implants have made extensive use of titanium and its alloys. However, bacterial infections and lack of adequate osseointegration due to poor bone quality have been linked frequently to result in implant failure. Gold nanoparticle integration on titanium implants has been earlier shown to have a bactericidal effect on the growth of Staphylococcus aureus (S. aureus) and Escherichia coli (E. coli). Furthermore, no cytotoxicity was observed in the animal model [31]. The authors attributed this effect to the photocatalytic memory effect of gold nanoparticle-impregnated titanium oxide nanotube implants.
The results of another study [32] in rabbits using titanium (Ti) implants immobilized with gold nanoparticles showed that the osteogenic parameters were better in the gold nanoparticles stabilized titanium implant group. This implies the potential benefit of gold nanoparticles in enhancing osteogenicity in low bone-density osteotomy sites, such as those in cases of osteopenia and osteoporosis.
In a study by Rendina et al. [33], it was observed that in osteopenic ovariectomized mice, dried apricots (twenty-five percent of the diet for eight weeks) increased bone mineral density in the body and spine. In another study by Kovacova et al. [34], they studied the effects of crushed bitter apricot kernel feed on rabbits over five months. The results showed that the consumption of apricot seeds did not reveal any significant impact on the rabbits' body weight or bone characteristics such as bone volume, density, thickness, and surface area according to 3D microcomputed tomography analysis. However, histo- logical analysis revealed changes in the cortical bone microstructure, indicating potential negative effects, including decreased density of secondary osteons and changes in vascularization and biomechanical properties. This shows a variation in the results in scientific literature [34]. This variation may be due to the differences in the amount and presence of bioactive compounds in plant products across plants grown in different geographical zones. The effects of apricot kernel extract on the osteotomy sites for implant placement and its effect on osseointegration are not mentioned in the searched literature. Hence, this is the first study, to the best of our knowledge, to deal with it in this regard. We observed that the control group without any additives had more inflammation and less bone formation. Au-coated implants and Apricot-coated implants showed enhanced osteogenesis with reduced inflammation. The role of gold particles in various therapeutic modalities [35-37] and their biocompatibility compared to osteoinductive potential [38] are still not known. Apricot kernels have been used for various medicinal purposes, including cancer, cerebral function, chronic inflammation, and hypertension [39, 40]. However, overdosage with apricot kernels can lead to cyanide poisoning [39]. The role of nanoparticles has been experimented on various implant types for their biological activities [41-43].
This histological study comparing the effect of gold nanoparticles and apricot kernel extract on the osseointegration of dental implants has a few limitations. Firstly, although gold nanoparticles are used in various emerging therapies, the biocompatibility and osseo- inductive potential of gold nanoparticles need to be further investigated. Thirdly, the study was short. Fourthly, a lack of Electron Microscopy (SEM) use, which would have revealed high-resolution details about the apposition between the groups. Finally, the bioactive ingredients of our study on the apricot kernel extract were not studied or characterized. However, the potential of these modifications to improve bone apposition around dental implants is immense and thus warrants attention.
CONCLUSION
This study deals with the application of gold nanoparticles and apricot kernel extract and compares and evaluates the inflammatory response at the dental implant site upon the application of these materials. It also evaluates the effects of these applications on the cell behavior at a histological level and the bone apposition at the surface of pure titanium dental implants in an animal model. These results appear to be promising. However, this preliminary study warrants further research.
AUTHORS’ CONTRIBUTION
It is hereby acknowledged that all authors have accepted responsibility for the manuscript's content and consented to its submission. They have meticulously reviewed all results and unanimously approved the final version of the manuscript.
ABBREVIATION
SEM | = Electron Microscopy |
ETHICS APPROVAL AND CONSENT TO PARTICIPATE
The ethical approval for the study was obtained from the institutional animal care and use committee, College of Veterinary Medicine, University of Mosul, Mosul, Iraq, on 15/04/2023 with reference no UM.VET.2023.013
HUMAN AND ANIMAL RIGHTS
This study adheres to internationally accepted standards for animal research, following the 3Rs principle. The ARRIVE guidelines were employed for reporting experiments involving live animals, promoting ethical research practices.