All published articles of this journal are available on ScienceDirect.
Glass Ionomer-Carboxymethyl Chitosan Cement: Setting Time, Microhardness, Mineral Phase, Crystallinity, and Dentin Remineralization Potential
Abstract
Background
The application of Glass Ionomer Cement (GIC) stimulates dentin remineralization. Carboxymethyl-Chitosan (CMC) can stabilize Amorphous Calcium Phosphate (ACP) nanoclusters that promote intrafibrillar remineralization. However, the effect of modifying GIC with CMC in dentin remineralization has not been widely reported.
Objective
This study aims to determine how supplementing GIC material with CMC affects its setting time and crystallinity and evaluate how applying 5% and 10% GIC-CMC to demineralized dentin affects the dentin’s microhardness, mineral phase, and degree of crystallinity.
Methods
GIC was mixed with CMC at 5% and 10% ratios to produce GIC-CMC5% and GIC-CMC10%. GIC, GIC-CMC5%, and GIC-CMC10% were applied to acrylic molds. Their setting times and crystallinity were tested using the Vicat needle test and X-Ray Diffraction (XRD), respectively. The dentin samples were made into cavities, demineralized, and treated with GIC, GIC-CMC5%, and GIC-CMC10%. The tooth roots were soaked in phosphate-buffered saline for 14 days. Remineralization in the dentin was evaluated based on microhardness values using the Vickers test, and its mineral phase and degree of crystallinity were assessed using XRD.
Results
GIC-CMC (5% and 10%) had shorter setting times than the GIC but the same crystallinity. The microhardness of the treated dentin in the GIC-CMC5% and GIC-CMC10% groups increased compared to the GIC group. Hydroxyapatite crystals formed in the GIC and GIC-CMC samples, with the highest crystallinity in the GIC-CMC10% sample.
Conclusion
GIC-CMC had a shorter setting time than GIC but the same crystallinity. Applying GIC-CMC10% to demineralized dentin increased the dentin’s microhardness and crystallinity and promoted hydroxyapatite crystal mineral formation.
1. INTRODUCTION
Treatment in the field of conservative dentistry uses the minimal intervention dentistry approach, which involves removing infected dentin tissue and maintaining affected dentin [1]. Affected dentin can be preserved because it can still be remineralized. Accordingly, various types of materials with remineralization capabilities have been developed, one of which is Glass Ionomer Cement (GIC) [2]. GIC can bond at the chemical level, as ionic bonds are formed between the carboxylate group present in the GIC and calcium ions in the teeth [2]. In this process, active ions such as phosphate, silicate, fluoride, and calcium are released, which can interact with the minerals in the teeth and enhance remineralization [3]. However, research shows that GIC application stimulates only classical remineralization, which causes interfibril remineralization but not intrafibril remineralization; thus, it does not restore the mechanical properties of dentin [2, 4].
Intrafibrillar remineralization requires non-collagen proteins [5, 6]. The non-collagen protein Dentin Matrix Protein 1 (DMP1) plays a role in stabilizing Amorphous Calcium Phosphate (ACP) so that it does not aggregate before entering the gap zone [7]. One of the non-collagen protein analogs is Carboxymethyl Chitosan (CMC) [3], which is rich in carboxyl and phosphate groups that can stabilize ACP nanoclusters [7]. Many modifications have been proposed to improve the clinical performance of this material. Soygun et al. added CMC to GIC liquid at ratios of 5% and 10% and found an increase in the microhardness of the modified material [8]. Chen et al. noted 14 days after initializing remineralization that CMC acts as a DMP1 analog and stabilizes the ACP nanoprecursor so that it can enter through the gap zone [3].
Sayed et al. showed that supplementing GIC with chitosan can reduce the setting time of the material compared to GIC alone, but the resulting crystallinity is not as high as that of GIC [9]. However, research on the effect of modifying GIC with CMC at ratios of 5% and 10% on setting time and crystallization has not yet been conducted. Moreover, no studies have evaluated whether the GIC-CMC blend promotes the remineralization of demineralized dentin. This study aims to fill this gap and evaluate treated dentin’s microhardness values quantitatively using the Vickers test and its mineral phase and crystallinity qualitatively using XRD. The null hypothesis of this study is that there is no difference between the materials and their application to dentin.
2. MATERIALS AND METHODS
The research tools were rectangular acrylic molds, 100-ml bottles, cylindrical diamond burs, high-speed handpieces, digital scales, mixing slabs, plastic cement spattle, plastic filling, cement plugger, dental loupe, vortex spinning machine, a shaking incubator, a Vicat tool needle, Vickers test equipment (a HMV-G21DT Micro Hardness Tester, Shimadzu, Japan), and XRD test equipment (a Rigaku Smartlab X-Ray Diffractometer, Rigaku, Japan). The inclusion criteria for tooth samples were caries free-premolar, never undergoing orthodontic treatment, extracted within 14 days, and stored in phosphate-buffered saline solution. The exclusion criteria were teeth that had defects in the coronal portion. The research materials were tooth samples that fit the inclusion criteria, Phosphate-Buffered Saline (PBS) solution, nail polish, 17% EDTA (Ethylenedia- minetetraacetic acid) solution, aquabidest, 1 M NaCl, alcohol, CMC powder (PUI Chitosan and Advanced Materials, University of Sumatra North, Indonesia) and GIC (Fuji IX, GC Corp, Japan). This study is a laboratory experimental test carried out at the laboratory in Universitas Indonesia and the National Innovation Research Agency in February–May 2023 with clearance from the committee (Nomor: 26/Ethical Exempted/ FKGUI/IX/2021 with protocol number 050710921 and Nomor: 03/Ethical Exempted/FKGUI/II/2023 with protocol number 050060123).
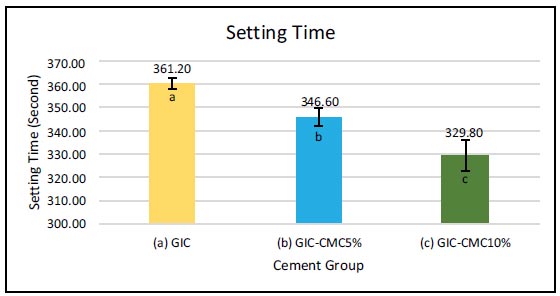
The modified material was made by adding CMC powder to GIC to yield GIC-CMC5% (5% CMC and 95% GIC) and GIC-CMC10% (10% CMC and 90% GIC). The material was mixed with a vortex-spinning machine until homogeneous. The GIC powder and liquid were mixed according to the manufacturer's instructions. In the material test, the results of the GIC mixture were placed in 10 acrylic molds for each GIC group and the GIC-CMC5% and GIC-CMC10% groups. To test setting times, each sample was placed on a Vicat needle. The needle on the tool was placed perpendicular to the sample’s surface and left for 5 seconds. This step was repeated at time intervals of 10 seconds. It was observed that the marks were formed by the needle on the surface of the sample. The setting time was determined when the needle stopped leaving marks. For the crystallinity test, the sample was observed after 24 hours and analyzed using X-Ray Diffraction (XRD). The XRD test results are presented in graphical form in Figs. (1 and 2).
In the dentin research, 28 caries-free premolar tooth samples were divided into four groups: the control group (demineralized dentin), Group I (demineralized dentin treated with GIC), Group II (demineralized dentin treated with GIC-CMC5%), and Group III (demineralized dentin treated with GIC-CMC10%). The sample size was determined based on previous studies [7, 8, 10]. Each tooth sample was prepared to form a cavity measuring 3 x 3 x 3 mm. The tooth root was cut 2–3 mm from the apical to facilitate PBS fluid delivery into the cavity. We demineralized the samples by soaking them in 17% EDTA for one week. The GIC-CMC5% and GIC-CMC10% were then applied to the cavities. The root surfaces of the samples were soaked in a shaking incubator containing PBS for 14 days to simulate the conditions of teeth in the oral cavity. For the Vickers microhardness test, the samples were cut in a midcoronal direction, the tooth was transversely divided into two parts, and the samples were cleaned using aquabidest. The samples were placed on the mold's base, filled with liquid acrylic resin, and left for 24 hours until hardened. Then, the samples were grounded with silicon carbide paper (800-, 1000-, 1500-, and 2000-) and polished. The prepared samples were evaluated using a microscope connected to a Vickers test device before indentation. For the XRD test, the cement material was removed using silicon carbide bur, and the interface area between the restoration and dentin was cleaned using aquabidest. The samples were then cut to produce a dentin block with a length and width of 5 mm x 5 mm and a thickness of 2 mm. The dentin’s microhardness was measured quantitatively using a Vickers Micro Hardness HMV-G21DT (Shimadzu, Japan). Indentations were created at five points on each sample, and the average was calculated as the Vickers Hardness Value (VHN). Each sample’s mineral phase and degree of crystallinity were determined using XRD (Rigaku Smartlab X-Ray Diffractometer, Japan). The resulting graphic data were then processed to determine the mineral phase and degree of dentin crystallinity for each sample.
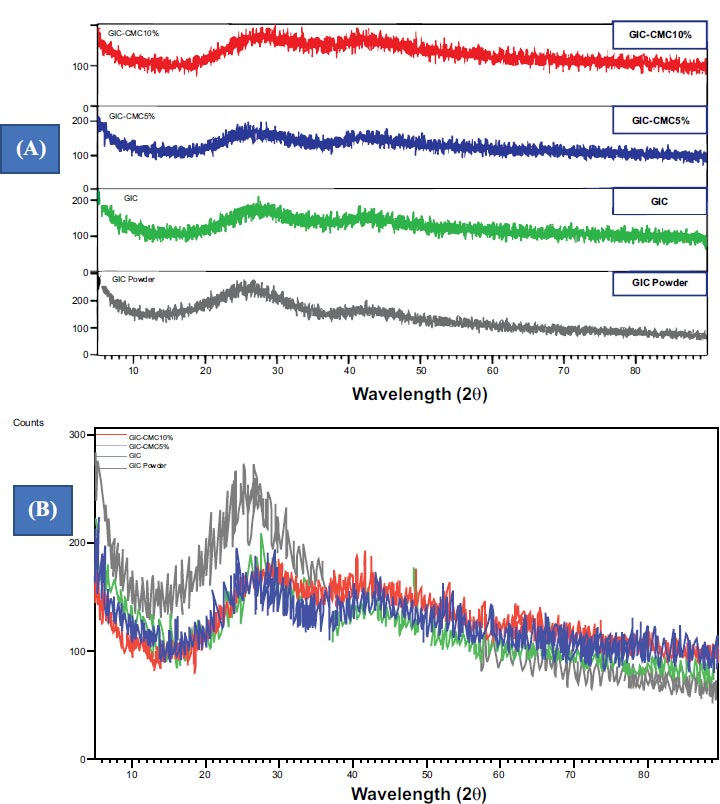
3. RESULTS
The material research was divided into three groups: Group 1 as a control group (GIC), Group 2 (GIC-CMC5%), and Group 3 (GIC-CMC10%). The data were analyzed statistically using SPSS Statistics 22.0 software.
It can be seen in Fig. (1) that the highest value for setting time was found in the GIC group (361 s), followed by GIC-CMC5% (347 s) and GIC-CMC10% (330 s). Tamhane’s post hoc test of setting time showed a significant difference between GIC and GIC-CMC5%, GIC and GIC-CMC10%, and the times of GIC-CMC5% and GIC-CMC10%. Crystallinity tests were also carried out on all groups, which were viewed using XRD PANalytical X'pert Pro (Netherlands), and the data were processed using HighScore Plus software.
Fig. (2) shows the results of XRD tests of GIC powder, liquid GIC, and the mixtures with CMC. GIC-CMC5% and GIC-CMC10% show a broad band pattern, wide peaks, and no peaks that are sharp, pointed, and narrow. A design like this is typical in the diffraction of amorphous structures (Fig. 2A). The pattern of GIC powder shows one sharp peak, while adding the solution to GIC powder decreases its intensity, and supplementing GIC with CMC5% and CMC10% decreases it further (Fig. 2B). This indicates the typical characteristics of amorphous structure diffraction.
The dentin samples were divided into four groups: demineralized dentin, demineralized dentin treated with GIC, demineralized dentin treated with GIC-CMC5%, and demineralized dentin treated with GIC-CMC10%.
In Fig. (3), the highest value for dentin microhardness was found in the GIC-CMC10% group (65.8 VHN), followed by GIC-CMC5% (62.5 VHN) and GIC (53.2 VHN). The lowest value was in the demineralized dentin group (5.7 VHN). Kruskal–Wallis test showed that there were statistically significant differences in microhardness between the four groups. We also tested for significant differences using the Mann–Whitney post hoc test. As Fig. (3) shows, the differences in microhardness between the GIC and GIC-CMC5% groups were statistically significant. Significant differences in microhardness were also identified between the GIC and GIC-CMC10% groups and between the GIC-CMC5% and GIC-CMC10% groups.
XRD analysis was carried out to determine the characteristics of the minerals that composed the dentin samples in each group. The XRD test results came in the form of diffraction spectra with intensity peaks (Fig. 4). These graphic XRD data were then processed to determine the mineral phase of each sample. Fig. (4) presents the XRD results in the form of graphic patterns that describe the characteristics of the minerals that make up each sample. The wide, sloping peak in the demineralization group indicates that the sample was amorphous and had a low degree of crystallinity. Its pattern was then analyzed, and it showed a match with the brushite and octa calcium phosphate phases. For the GIC, GIC-CMC5%, and GIC-CMC10% samples, the graphs show peaks at 2θ 26° and 32°. Their graphic pattern matches the hydroxyapatite phase. The sharp, narrow peaks in all three samples indicate higher crystallinity. However, the peaks of the GIC sample look wider than the peaks of the GIC-CMC5% and GIC-CMC10% samples, which are sharper and narrower, and the peak intensity of the GIC-CMC10% sample is higher than that of the GIC-CMC5% sample.
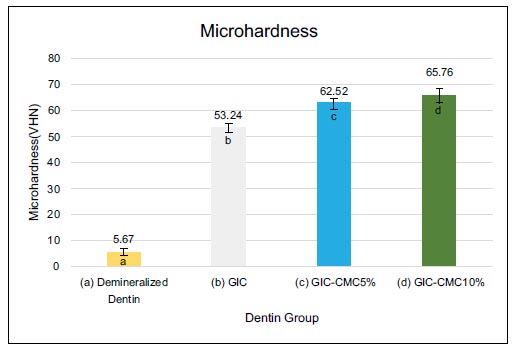
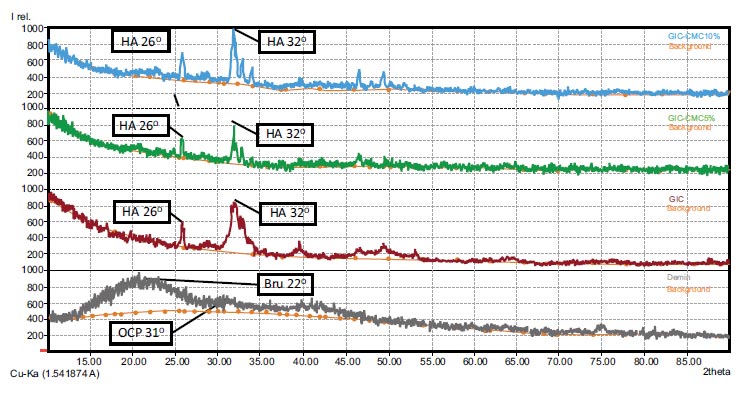
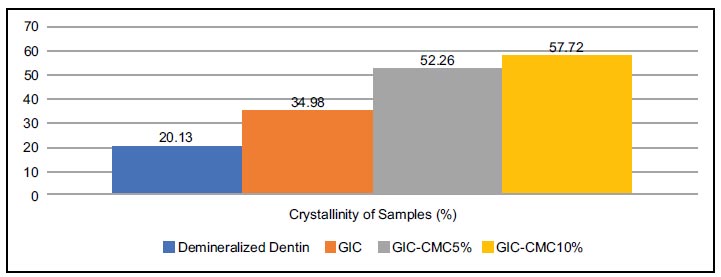
Apart from determining the mineral phases, the graph of the XRD test results was further analyzed to determine the crystallinity of each sample. The crystallinity of a sample is determined by the extent (represented in percentage) to which it exhibits a crystalline structure rather than an amorphous structure (Fig. 5). Fig. (5) shows the extent to which the four samples crystallized. The highest crystallinity was found in the GIC-CMC10% group (57.72%), followed by GIC-CMC5% (52.26%) and GIC (34.98%). Meanwhile, the lowest crystallinity was found in the demineralization group (20.13%).
4. DISCUSSION
We carried out a laboratory experiment by modifying GIC with CMC and found the impact of reducing its setting time, but it did not affect the crystallinity. We also found that applying GIC-CMC5% and GIC-CMC10% to demineralized dentin increased the dentin’s micro- hardness and, affected mineral phase and crystallinity. The null hypothesis in the study, which stated that there was no difference between the three materials and their application to dentin, was rejected. The results showed that there was a significant difference in the setting time of GIC compared to that of GIC-CMC. The setting time required for GIC was longer than that of GIC-CMC, and the time decreased as the CMC concentration increased. This phenomenon can be linked to the research of Sayed et al., who showed that adding chitosan to GIC yields reduced working and setting times compared with GIC alone [9]. This decrease in setting time may be due to the effects of the reaction that occurs when chitosan is mixed with polyacrylate in the liquid. Chitosan is considered a strong base because it has a free amino group (NH3+) when dissolved in polyacrylic acid. A reaction occurs between the amino group (-NH2) in chitosan and the functional groups (OH and C=O) in the GIC. Another factor that influences the reduction in setting time is that the hydroxyl and acetamide groups in chitosan bond with the hydroxyl and polycarboxylate groups in GIC powder through hydrogen bonds [9]. The chitosan groups react with the -Si-O-Si- chain units in the surface of the glass particles in GIC, which leads to the formation of -Si-O-OH groups [9, 10]. However, the results of this study differ from those of Putranto et al., who mixed CMC/ACP with gypsum and found an increase in the working time of the modified material [11].
The results of the XRD analysis show that there is no structural difference between GIC and CIG-CMC and that their structure is amorphous. This amorphous structure is explainable by the dominance of SiO2 in the composition of GIC (41.9%). Silica’s structure is divided into crystalline and amorphous areas. In quartz and cristobalite silica, the structure is crystalline, but silica glass particles have an amorphous structure. The dominant raw material of GIC, silica glass, thus produces amorphous XRD results. Even being mixed with liquid does not change its amorphous structure.
The results of this research are in accordance with a study by Maximilian et al. involving XRD tests of GIC powder and GIC mixtures, which showed amorphous graphic results [12]. Our results are also consistent with those of Melo et al., Tadiboyina et al., and De Mayer et al., who similarly conducted XRD analyses of GIC and found that the X-ray diffractograms had wide and irregular peaks characteristic of amorphous materials [13-15]. Melo et al. noted that the amorphous structure formed was related to the dominant percentage of silica in the composition of GIC [13].
In this study, combining GIC with CMC5% and CMC10% did not affect its amorphous phase. These results may be explained by the research of Sayed et al., who tested the crystallinity of modified GIC and chitosan, finding that the polymer structure of chitosan combines into a matrix network along with inorganic crystals [9]. This polymer has a lower surface hardness compared to glass particles. As a result, the surface hardness of inorganic crystals in GIC will be decreased as the percentage of added chitosan increases [9]. Using XRD testing, Putranto et al. found no change in the diffraction spectra of gypsum and modified gypsum samples containing CMC/ACP5% and CMC/ACP10% [11]. Bao et al. argued that the addition of CMC can play a role in improving the physical properties of GIC at certain concentrations, but excessive addition of CMC can reduce its mechanical performance [16].
We also examined how applying GIC-CMC5% and GIC-CMC10% to demineralized dentin affected the dentin’s microhardness, mineral phase, and crystallinity. We used non-carious extracted human premolars as samples. Post-extraction, the teeth were immediately soaked in Phosphate-Buffered Saline (PBS) before the research was carried out. Uquillas et al. noted that soaking teeth in PBS solution can ensure that type I collagen fibrils remain dense and retain mechanical strength [17]. The demineralization procedure was carried out by soaking the dentin in 17% liquid EDTA for 7 days and placing it in a shaking incubator at a temperature of 37°C [3]. Cao. et al. used EDTA for demineralization because it can maintain the dentin collagen matrix intact and produce a mineral-free dentin surface [18, 19]. Following the deminerali- zation process, the dentin should be rinsed with aquabidest for 30 minutes and soaked in 20 mL of 1M NaCl (pH 7.0) for 8 hours. The purpose of immersing the sample in NaCl is to remove non-collagen proteins in the dentin [3]. This procedure is intended to produce dentin that resembles the affected dentin [3, 20].
PBS solution contains various ions that resemble body fluids, such as sodium chloride, sodium bicarbonate, potassium chloride, and potassium phosphate. Thus, following the demineralization process, the reminerali- zation material was applied to the treatment group samples, and they were stored in a container containing PBS [21]. A remineralization evaluation was carried out 14 days later. Mousavinasab et al. found that a release of fluoride ions from GIC (Fuji IX, GC Japan) occurred during the first week after initiating remineralization and increased in the second week [22]. Meanwhile, Chen et al. found after two weeks that CMC had acted as a DMP1 analog that stabilized ACP nanoprecursors so that they could infiltrate collagen fibrils through the gap zone [3]. Meanwhile, following the application of GIC combined with CMC on demineralized dentin, Budiharjo et al. (2010) discovered the formation of hydroxyapatite 7 days after initiating remineralization, and this formation developed further after 14 days [23].
We used the Vickers test because it was easy, relatively fast, and only required a small area of the sample to be tested [24-27]. The Vickers test was carried out with a load of 50 grams (0.49 N) for 15 seconds in accordance with Hamama et al. [29] The smallest value was seen in the demineralization sample group, which had an average of 5.67 VHN (Vickers Hardness Number). The demineralization process, accomplished using EDTA, removed most of the minerals from the dentin’s surface [18, 19]. Following the application of GIC, the hardness value increased to 53.24 VHN. The use of GIC is known to be able to halt the demineralization process and restore lost minerals [2, 4, 30]. This phenomenon can be explained by the research from Gao et al., who explain that the hydrogen ions in the polyalkenoic acid in GIC liquid will dissolve GIC glass particles so that calcium and aluminum ions are released from the cement [2]. GIC is acidic, so calcium and phosphate ions are released from the enamel–dentin surface [2, 31]. Following this, polyacid buffering occurs; the previously acidic environment experiences an increase in pH, and precipitation of the fluoridated carbonatoapatite mineral occurs at the interface of the tooth and cement [2].
In the GIC-CMC5% and GIC-CMC10% groups, the dentin’s hardness was increased compared to the GIC group, reaching 62.52 and 65.76 VHN, respectively. This value is close to the normal value of dentin hardness, which ranges from 61 to 83 VHN [29, 32]. This phenomenon can be explained by the work of Nimbeni et al., who noted that the chitosan molecule has superior biological properties, including an antimicrobial function, can bind naturally, and act as a reservoir of calcium and phosphate ions that play a role in remineralization [33]. CMC forms not only hydrogen bonds but also ionic bonds with the inorganic particles in GIC [23]. Chitosan can penetrate the dentin structure and carry the ions needed to deeper areas [33]. Our results are also consistent with those of Elshenawy et al., who evaluated GIC treated with chitosan and found a significant increase in hardness [34].
In this study, XRD was used to evaluate the demineralization and remineralization phases of dentin qualitatively [35, 36]. The demineralized dentin samples showed wide sloping wave peaks, indicating the absence of hydroxyapatite crystals and, thus, demineralization [35]. Through XRD graphic analysis, we found that the mineral phase of the demineralized sample was brushite and octa calcium phosphate, which is the precrystalline phase of hydroxyapatite, that is, the main mineral that makes up dentin. The brushite phase, or dicalcium phosphate dihydrate (CaHPO4.2H2O), has a higher solubility than octa calcium phosphate or hydroxyapatite [37]. Octa calcium phosphate (Ca8.(HPO4)2(PO4)4.5H2O) is known to be a precursor to hydroxyapatite, with a stable structure [38]. Following the application of GIC, GIC-CMC5%, and GIC-CMC10%, several wave peaks were seen, namely, at 2θ 26° and 32°. The narrow and sharp wave peaks at 2θ 26° and 32° imply the hydroxyapatite phase. CMC induces a significant deposition of calcium and phosphate ions on the surface of demineralized dentin, thereby enhancing crystal nucleation [33].
Graphic XRD data can also be used to evaluate the crystallinity, which indicates the percentage of material that assumes a crystalline rather than amorphous form [5, 35]. In the demineralized dentin sample, a sloping graph could be seen, indicating an amorphous condition and low crystallinity [35]. The demineralized sample consisted of brushite and octa calcium phosphate, which had the lowest crystallinity at 20.13%. In the GIC sample, a peak could be seen, indicating that hydroxyapatite crystals had started to form; however, the peak state was wider than in the GIC-CMC groups. In the GIC group, we found increased crystallinity at 34.98%. In the GIC-CMC5% and GIC-CMC10% samples, the graph peaks were sharper and narrower than in the GIC sample, indicating a more complete precipitation of hydroxyapatite crystals in the samples. This is supported by the increase in their crystallinity values, which reached 52.26% and 57.72%, respectively.
Remineralization can increase microhardness, which is influenced by changes in mineral content [26, 39]. In turn, these changes have an effect on mineral phase and crystallinity value [38]. The denser the mineral phase and the higher the crystallinity, the higher the dentin’s microhardness value [4, 40]. In sum, in this study, demineralized dentin was successfully remineralized using GIC supplemented with CMC, and the treated dentin was evaluated using microhardness and XRD tests. This study managed to evaluate whether the GIC-CMC blend promotes the remineralization of demineralized dentin through several tests, both quantitative and qualitative. However, this is a laboratory study that had many weaknesses and limitations in simulating actual oral conditions. Further clinical tests are needed to evaluate the effect of GIC-CMC in demineralized dentin, as in the caries process.
CONCLUSION
Based on this research, it can be concluded that increasing the concentration of CMC added to GIC reduces the latter’s setting time significantly but does not affect its crystallinity. Supplementing glass ionomer cement with 10% carboxymethyl chitosan effectively increases the microhardness value, promoting the formation of hydroxyapatite crystal minerals, thereby increasing the crystallinity of demineralized dentin.
AUTHORS' CONTRIBUTIONS
It is hereby acknowledged that all authors have accepted responsibility for the manuscript's content and consented to itssubmission. They have meticulously reviewed all results and unanimously approved the final version of the manuscript.
LIST OF ABBREVIATIONS
ACP | = Amorphous Calcium Phosphate |
CMC | = Carboxymethyl Chitosan |
EDTA | = Ethylenediaminetetraacetic Acid) Solution |
GIC | = Glass Ionomer Cement |
GIC-CMC | = Glass Ionomer Cement-carboxymethyl Chitosan |
SD | = Standard Deviation |
VHN | = Vickers Hardness Number |
XRD | = X-Ray Diffraction |
ETHICAL STATEMENT
This study is a laboratory experimental test carried out at the laboratory in Universitas Indonesia and the National Innovation Research Agency in February–May 2023 with clearance from the committee (Nomor: 26/Ethical Exempted/FKGUI/IX/2021 with protocol number 050710921 and Nomor: 03/Ethical Exempted/FKGUI/II/ 2023 with protocol number 050060123).
AVAILABILITY OF DATA AND MATERIALS
The data and supportive information are available within the article.