In vitro Antibacterial Effects of Curcumin-loaded Mesoporous Silica Nanoparticles inside the Dental Implant Fixture
Abstract
Background:
Infection in implants is a critical problem in the assemblies of two-piece implants.
Aim:
The purpose of this study was to test in vitro antibacterial effects of curcumin-loaded silica nanoparticles inside the implant fixture.
Methods:
Forty-five implants in five groups were used for evaluation of the antibacterial effects against Escherichia coli, Staphylococcus aureus, and Enterococcus faecalis. The groups were curcumin-containing silica nanoparticles, curcumin-free silica nanoparticles, curcumin, distilled water (negative control), and chlorhexidine (positive control). The mentioned materials in each group were poured into the inner cavity of the implant and then the abutment was closed (torque of 35 Ncm). All implant-abutment sections were dipped in bacterial suspension and incubated for 24 hours at 37°C. The inside of each implant was cultured on the surface of the culture plates.
Results:
The results showed that curcumin-containing silica nanoparticles affected all three bacterial species and the number of colonies in all three bacteria was significantly reduced. The nanoparticles showed antibacterial effects on S. aureus, E. coli and E. faecalis, which was statistically significant (p-value <0.05).
Conclusion:
The outcomes of this study showed that the curcumin-loaded mesoporous silica nanoparticles can be used inside the implant fixture for benefiting from their antimicrobial properties and further success of implant therapy.
1. INTRODUCTION
The microbial leakage in the abutment implant interface is a critical issue in the assemblies of two-piece implants [1]. A varied group of bacteria can enter and contaminate micro-gaps of implants [2]. These bacteria have been identified in several investigations as pathogenic bacteria producing peri-implant infections and periodontal diseases such as Peptostreptococcus micros, Porphyromonas gingivalis, Prevotella intermedia, Tannerella forsythia, Escherichia coli, Staphylococcus aureus, Enterococcus faecalis, and Actinobacillus actinomycetem- comitans [2-4].
Curcumin as a phytochemical is the principal curcuminoid found in turmeric [5, 6]. Curcumin is used as a coloring and flavoring agent in foods. It has also been utilized in traditional medicine for numerous beneficial aims [7]. Several investigations have displayed the antiviral, antifungal, antibacterial, and antimalarial actions of curcumin [8-10]. Due to the broad antimicrobial activities of this beneficial herbal agent, it was applied as a basis for the production of antimicrobial agents [11, 12]. Previous investigations also displayed that the local applications of curcumin gel reduced gingival inflammation and decreased the disease severity [13-15]. In addition, there was evidence that supports curcumin efficiently inhibits the inflammatory mediator’s activation and has therapeutic influences on periodontal diseases [13, 14]. Also, Cirano et al. presented that curcumin enhanced bone volume and improved bone-implant interaction in animal models [16].
Regardless of all the outstanding beneficial effects of curcumin, the bioavailability of this helpful agent was low due to its low solubility in water [17]. The oral absorption of curcumin is poor and it can be rapidly removed from the body [18, 19]. The chief way to improve curcumin bioavailability is the application of curcumin nanomaterials [20, 21].
Nanotechnology is an advanced approach principally to the improvement of more effective drug delivery systems [22-24]. Mesoporous silica nanoparticles (MSNs) have been defined as promising drug delivery structures [25, 26]. The interactions of MSNs have been shown with (bacteria-mimicking) lipid bilayers for antimicrobial peptide delivery [27].
Nanoporous silica has a range of potentials that make it an attractive choice for a controlled-release system. It has a large surface area and huge pore volumes with contracted pore size distributions for drug loading. The initial burst release from nanoporous silica could be used for the treatment of acute infections, and its controlled release profile can stay quiescent for lengthy stages and distribute cargo when triggered. As a consequence, the drug rests in the pores and could be removed when needed [25-27]. Owing to the antimicrobial possessions of curcumin and the useful features of porous silica nanoparticles as a sustained-release carrier, the current study aimed to assess the antibacterial action of mesoporous silica nanoparticles containing curcumin inside the implant fixture against S. aureus, E. coli, and E. faecalis. Then, the null hypothesis of this study is that curcumin-containing silica nanoparticles will not affect all three bacterial species and the number of colonies in all three bacteria will not be significantly reduced.
2. MATERIALS AND METHODS
2.1. Preparation of Mesoporous Silica Nanoparticles containing Curcumin
The mixture of surfactant (CTAB, Sigma Aldrich, USA), distilled water, and sodium hydroxide (NaOH, Sigma Aldrich, USA) was stirred for 30 minutes at 80 °C. The pH of the solution was then adjusted to 12.2. In the next step, tetraethyl orthosilicate (Sigma Aldrich, USA) was added to the solution immediately under a 550 rpm stirrer. A white precipitate was formed, which was mesoporous silica nanoparticles. The prepared nanoparticles were kept at 80 °C for two hours.
For the preparation of silica nanoparticles containing curcumin, 100 mg curcumin powder (Sigma Aldrich, USA) was dissolved separately in its suitable solvent (ethanol). Then, 1000 mg of the prepared silica nanoparticle powder (Temadkala, Tehran, Iran) was added to this solution. The resulting mixture was gently stirred at room temperature on the stereo for 24 hours in a dark place. Due to the mesoporous nature of silica nanoparticles, curcumin was settled molecularly in its cavities.
2.2. Characterization of the Nanoparticles
Transmission electron microscopy (TEM) was also applied to check the mesoporous structure (JEM-2100F; JEOL, Tokyo, Japan).
2.3. Determination of Curcumin Loading Inside Nanoparticles
To determine the amount of curcumin loaded on mesoporous silica nanoparticles, 10 mg of the prepared nanoparticles were dissolved in dimethyl sulfoxide (Sigma Aldrich, USA). For this purpose, about one milliliter of the dissolved nanoparticle solution was poured into a special tube of an ultraviolet spectrophotometer, and by adjusting Lambda Max to 350 nm for curcumin, the adsorption number was read. The amount of adsorption was converted to concentration.
2.4. Evaluation of Curcumin release Pattern from Silica Nanoparticles
To determine the pattern of drug release from nanoparticles, drug dissolution device No. 2 (Apparatus 2) was used. The drug dissolution apparatus 2 (DISi Series, UK), is in fact the most extensively used technique in drug dissolution testing especially for nanomaterials. It includes 6 wells and a set process including pH, temperature, and rotation speed. These parameters should be set according to the body's condition for a dissolution test of a drug (pH of 7.4, temperature of 37 ºC, and stirring rate of 100 rpm). Phosphate buffer (300 ml) was poured into each of the 6 wells of the device. The amount of 5 mg of the prepared nanoparticles was poured into all 6 wells. The pH of the liquid inside the valves was adjusted to 7.4. The device temperature was set to 37 ºC. The stirrer was set to 100 rpm. The samples were taken from the wells every 5 days (one milliliter) for 35 days and the absorbance was read using a UV spectrophotometer. The sample taken from the wells was replaced with one milliliter of a new buffer medium. The amount of adsorption was converted to concentration.
2.5. Implant Experiment Groups
The sample size was determined based on a pilot study. We used a sample size calculation method based on a preliminary sample of data which allows us to increase the accuracy of our inference by some specified amount with a specified level of confidence. Forty-five implants (DIO CO., Busan, South Korea) were categorized into five groups for evaluation of the antibacterial effects (9 implants per group). The first group was curcumin-containing silica nanoparticles, the second group was curcumin-free silica nanoparticles, the third group was curcumin, the fourth group was a negative control group (distilled water inside the implant cavity) and the fifth group was a positive control group (liquid chlorhexidine 0.2%). To perform the test, the suspensions for all groups with a volume of 10 µl (60 mg/ml) were poured into the inner cavity of the implant and then the abutment heel was closed (torque of 35 Ncm). The bacterial strains were grown individually. After performing the test for each of the bacterial species, the implants were autoclaved and sterilized and used for other bacteria (45 implants), and the bacterial test was performed on each of the bacterial species separately. Specifically, 45 implants were used 3 times.
2.6. Sampling and Microbial Test
In this study, 3 bacterial strains were used including E. coli (Gram-negative, facultatively anaerobic, coliform, rod-shaped bacterium), S. aureus (Gram-positive spherical-shaped bacterium), and E. faecalis (aerobic Gram-positive coccus). Bacterial strains, S. aureus (ATCC: 6538), E. coli (ATCC: 25922) and E. faecalis (ATCC 29212), were prepared as reference strains from the Pasteur Institute of Iran (Tehran, Iran). A nutrient Agar medium was used for bacterial activation. The cultivation time was 12 h for bacterial activation before use. The stationary phase of bacterial growth was used for contamination. For the bacterial concentration for contamination, 0.5 Mcfarland was used and the McFarland scale was used for concentration measuring.
All implants were immersed in 5 ml of bacterial suspension and then were incubated for 24 h at 37°C. Afterward, abutments were separated from implants. Then, 0.01 ml of implant contents were inoculated on the TSA plates. In addition, the diluted suspension (1:10, 1:102, 1:103, 104, and 1:105) was prepared using 0.01 ml of implant content and inoculated on the surface of TSA plates. Plates were incubated at 35°C for 24 h and the following formula was used for the calculation of CFU: CFU/mL꞊ Samples dilution × Number of colony × 10-1 (the dilutions were ready by 0.1 mL (10-1 mL of implants content). Cultured plates were chosen for counting on which the bacteria colonies separately grow and did not overlap [28]. The log conversion of CFU/ml amount was performed and plotted for all bacteria.
2.7. Statistical Analysis
We used SPSS 20 (IBM Company, USA) to compare the data between groups with a P value of < 0.05 as a significant level. The Shapiro–Wilk test was applied to check the normality of data. A one-way ANOVA test was utilized to compare the groups. Microbial tests were repeated three times.
3. RESULTS AND DISCUSSION
3.1. Characterization of Mesoporous Silica Nanoparticles containing Curcumin
According to reports, the physicochemical properties (particle size, morphology, loading percent, and release pattern) of nanoparticles have special effects on their other properties, especially on their interactions in vitro and in vivo. As the particle size decreases and the surface area increases, the interaction of these nanoparticles with the environment increases and the ways of crossing the body barriers and entering the cell in them will be different. The results of our research on curcumin-containing silica nanoparticles showed that the prepared nanoparticles had rod-shaped and mesoporous structures (Fig. 1). Previous studies have shown that the morphology of mesoporous silica nanoparticles is longer in the body compared to spherical morphology (more blood circulation) and less detected by the reticuloendothelial system (RES) than other morphologies. Zhao and colleagues compared the morphologies of mesoporous silica nanoparticles in an animal model. According to their results, rod morphology showed more durability in blood circulation and was also less detected by the RES system than other morphologies and showed special resistance to clearance from the liver and kidney [29].
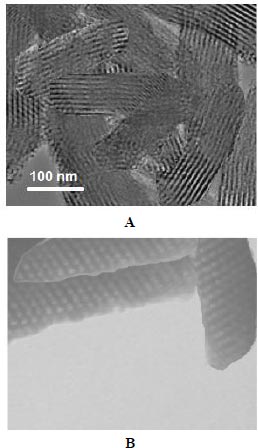
3.2. Pattern of Drug Release from Nanoparticles
The percentage of drug loading in nanoparticles for curcumin was obtained at 83%. Fig. (2) shows the pattern of release for curcumin from the prepared silica nanoparticles. According to the results, the nanoparticles showed a relatively rapid release pattern in the first 5 days (40 percent of loaded curcumin). The release of curcumin from the nanoparticles continued slowly until the 35th day (40 percent).
The pattern of rapid release of nanoparticles in the 5 days was related to adsorbed drugs with nanoparticles (drugs that are not in the cavities or have weak interactions (such as hydrogen-bonding and van der Waals) with the outer surface of the cavities) and continuous and slow release in the sixth to thirty-fifth days related to drug molecules are more internal (inside the cavities), which trapped in the cavities, also create electrostatic interactions with the cavity wall of nanoparticles and their release takes time. At pH 7.4, the phenolic groups of curcumin (pKa = 8.3) are deprotonated. Thus, there is an increase in the density of negative charges, resulting in an anion-type electrostatic repulsion between the −COOH groups of the silica nanoparticles and phenolic groups of curcumin [5].
The kinetic release model for the nanoparticles prepared in this study can be explained through the model reported by Zeng et al. [30]. In this model, the drug molecules are either adsorbed on the surface of the silica (resulting in fast release) or are trapped as free molecules inside the mesoporous part (resulting in sustained release) [31]. Memar et al. reported similar results for mesoporous silica nanoparticles containing the meropenem. According to their results, about 40% of meropenem was released from silica nanoparticles in two days and then continued its slow release until the 30th day [32]. Also in a study by Zhang et al., silica nanoparticles containing chlorhexidine (used in dental composites) showed a slow-release pattern [33].
3.3. Bacterial Effects
The results of our microbial studies showed that curcumin-containing silica nanoparticles affected all three bacterial species and the number of colonies in all three bacteria was significantly reduced (p<0.05). The effect of nanoparticles on bacteria (reduction of colony number) was E. coli (Fig. 1), E. faecalis (Fig. 2) and finally, S. aureus (Fig. 2), respectively, which was statistically significant (p<0.05).
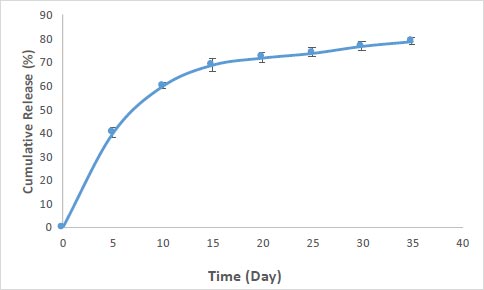
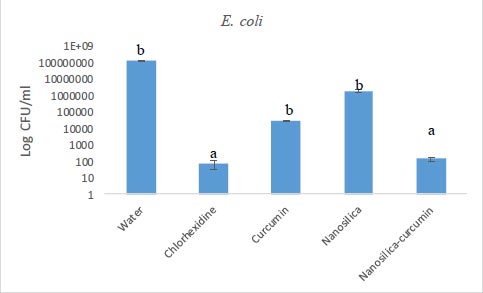
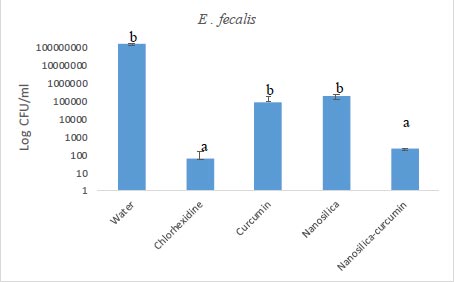
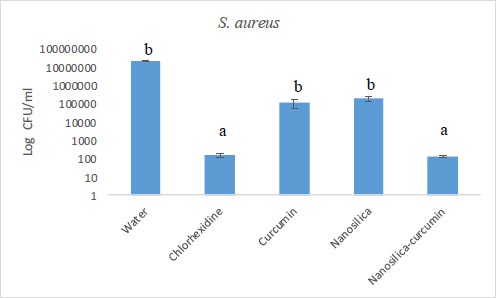
Chlorhexidine is the most widely utilized mouthwash and is considered the gold standard in dentistry, but it is not without certain disadvantages such as tooth discoloration, taste disturbance, mouth ulcers, and swelling of the unilateral or bilateral parotid [34, 35]. No significant difference was observed between the groups of chlorhexidine and silica-curcumin nanoparticles in all three bacterial strains (p>0.05) which means the silica-curcumin nanoparticles showed antimicrobial effects as potent as chlorhexidine, a strong antimicrobial agent. The herbal-based mouthwash formula works well in long-term use and nano-based herbal material can be better properties, which can be utilized as an alternative to overcome the drawbacks of chlorhexidine (Figs. 3-5).
Zhang et al. studied the role of mesoporous silica particles in the release of chlorhexidine by dental composites and their antibacterial effects. Their results showed that the use of these nanoparticles can improve the antibacterial effects of chlorhexidine and cause its long-term release (over 16 days) from the dental composite body [33]. In our study, the long-term release of curcumin from mesoporous silica nanoparticles was obtained (over 35 days) and also the antimicrobial effects of curcumin-containing nanoparticles were significantly increased compared to curcumin.
Memar et al. prepared and evaluated silica nanoparticles loaded with the antibiotic meropenem. Their results showed a two-step release for this antibiotic. Their microbial results also showed that the number of colonies for E. coli was significantly reduced for meropenem-loaded silica nanoparticles compared to free meropenem. In our study, there was also a significant difference between curcumin and curcumin-containing silica nanoparticles. In their work, similar to ours, drug-free silica nanoparticles showed little antimicrobial effect [36]. In another study, Koneru and colleagues developed silica nanoparticles containing tetracycline. In the study of this group, similar to our study, the antimicrobial effects of tetracycline-containing silica nanoparticles were significantly higher than that of free tetracycline against E. coli [37].
Indeed, the burst release phase of these nanoparticles can treat acute infections, and its controlled release outline can distribute cargo when triggered [25-27].
The mechanisms of nanoparticles are different from the mechanism of common antibiotics. Then, the application of antimicrobial nanoparticles is a beneficial method against drug-resistant microbes [38]. According to studies, the doses of nanoparticles and physicochemical properties (shape, size, surface properties) are effective in their antimicrobial activities [39, 40]. The investigations showed that nanoparticles have the capability to disrupt cell membrane functions by adhering to the cell membrane surface [41]. In smaller nanoparticles, this effect was more prevalent because of their larger surface area [40, 42]. The membrane-nanoparticle interaction causes local pores in the membrane and principally bacterial damage. The binding of nanoparticles to the membrane of bacteria, their gradual entrance into the cytoplasm, and disturbing the bacterial functions are other probable mechanisms for the antibacterial actions of antimicrobial nanoparticles. Some drug-loaded nanomaterials can also combine with the cell wall of bacteria and transfer their antimicrobial agent into the bacteria [42, 43].
CONCLUSION
Regardless of the point that treatment with implants is a commonly successful process, however, implant failure can happen owing to mechanical, and biological factors that cause bacterial infections.
The results showed that the use of silica nanoparticles containing curcumin with a slow-release pattern as well as significant antibacterial effects could be effective in controlling bacterial infection. Besides, there was no significant difference between the chlorhexidine and silica-curcumin nanoparticles groups in all three bacterial strains (p>0.05) indicating the similar antimicrobial effects for silica-curcumin nanoparticles and chlorhexidine as a known strong antimicrobial agent. The use of these nanoparticles in the optimal formulation and appropriate concentration can be used in the future to replace the use of chemical antimicrobials or to reduce bacterial resistance along with the standard gold chlorhexidine. It can be concluded that the delivery of silica nanoparticles improves the antibacterial effect of curcumin in dental implants.
FUTURE PERSPECTIVES
The sustained release pattern of the prepared silica-curcumin nanoparticles together with their antimicrobial effects can increase their clinical efficacy in the dentistry field. Improved formulations using recent advances in nanotechnology would benefit to show the true antibacterial and anti-inflammatory potential of curcumin. Different delivery formulations of curcumin need to be more reconnoitered. Further studies are vital to discover the effect of curcumin on dental and periodontal diseases clinically.
ETHICS APPROVAL AND CONSENT TO PARTICIPATE
This study was accepted by the Ethics Committee of the Tabriz University of Medical Sciences (IR.TBZMED.VCR.REC.1399.247).
HUMAN AND ANIMAL RIGHTS
No animals were used in this research. All procedures performed in studies involving human participants were in accordance with the ethical standards of institutional and/or research committees and with the 1975 Declaration of Helsinki, as revised in 2013.
AVAILABILITY OF DATA AND MATERIALS
The raw/processed data required to reproduce these findings can be shared at this time.
FUNDING
The Vice Chancellor for Research at Tabriz University of Medical Sciences provided financial support.
CONFLICT OF INTEREST
Dr. Solmaz Maleki Dizaj is the Editorial Advisory Board Member for The Open Dentistry Journal.
ACKNOWLEDGEMENTS
This article was written based on a dataset from a thesis registered at the Faculty of Dentistry, Tabriz University of Medical Sciences. The Vice Chancellor for Research at Tabriz University of Medical Sciences provided financial support for this research that was greatly acknowledged.