Comparative Evaluation of Microleakage of Two Variables of Glass-Ionomer Cement: An In vitro Study
Abstract
Introduction:
The emergence of nanotechnology, which has become an important research area in dentistry, has made it possible to obtain nano-structured materials with improved properties compared to their basic form. In this work, we have performed an experimental study on natural teeth to evaluate the microleakage of a Ketac™ Universal nano-filled GIG in box cavities and compare it to a Ketac™ Fil Plus (3M ESPE) conventional GIG.
Materials and Methods:
80 Class II cavities were prepared on the mesial and distal surfaces of forty teeth (twenty molars and twenty premolars). The sample was divided randomly into two groups: Group1 with conditioning and Group 2 without conditioning. The mesial cavities were obturated with Ketac™Universal nano-filled GIG and the distal cavities with Ketac™ Fil Plus GIG. The teeth underwent thermocycling, followed by infiltration with methylene blue. The teeth roots were inserted into methacrylate resin blocks and then sectioned with a chainsaw in the center of both restorations. After observation with a stereomicroscope, the extent of methylene blue infiltration in each restoration was recorded separately for the cervical surface (from 0 to 2) and the axial surface (from 0 to 3).The Chi-squared test with SPSS20.2 software was used to investigate the score distribution.
Results:
Results were considered significant if P<0.05. The Chi-square test showed a significant difference between the nano-filled GIG and the condensable GIG. The nano-filled GIG showed less infiltration, and it was found to be more tight than the conventional GIG.
Conclusion:
The results of the current study suggest that the use of nanotechnology is a promising strategy to improve the clinical performance of GIG. However, this study is limited to an experimental in vitro strategy, which cannot reliably reproduce clinical reality.
1. INTRODUCTION
Thanks to their improved aesthetic and functional properties, dental nano-filled resin composites are considered advanced materials in restorative dentistry [1].
Dental filling with direct posterior resin composites is used to substitute lost enamel and dentine tissues. Leakage and secondary caries often represent one of the main adhesive restoration failure reasons because they normally show a volumetric polymerization shrinkage, considered as post-gel shrinkage, from 1% up to 4.5% [2]. Various studies have suggested to not use shrinking adhesive dental materials, such as glass ionomers, in combination with composite, to reduce the critical resin-based materials stress distribution in enamel and dentine replacement [3].
Glass-ionomer cements (GICs) were introduced to the market at the end of the 1970s, and since then, their composition has been constantly modified in order to better adapt to different clinical situations.
The advent of nanotechnology has enabled researchers to incorporate nanoparticles into the composition of these materials [4] in order to overcome their poor mechanical and water properties [5]. GICs are characterized by spontaneous adhesion to calcified dental tissue, continuous release of fluorides (Czarnecka 2002), and low cytotoxicity [6]. These materials have three major drawbacks: poor mechanical properties, long setting time, and high sensitivity to humidity [7]. Due to these limitations, over the years, many researchers have become interested in optimizing the composition of glass to improve the clinical performance of cement [8].
In recent years, we have witnessed a rapid evolution of dental biomaterials through the emergence of nanotechnology, which has established itself as one of the most innovative techniques to improve the properties and clinical indications of GICs [9]. Nanotechnology has enabled researchers to incorporate nanoparticles into the composition of these materials in order to overcome their shortcomings [9], and to better adapt to hard dental tissues (enamel, dentin, cement), which are composed of the structural units at the nanoscale [10]. Nanotechnology can be defined as the science and engineering involved in the design, synthesis, characterization, and application of materials, whose smallest functional organization is at the nanometer scale (0.1 to 100 nanometers) [11, 12]. Indeed, glass ionomer nanocements are the subject of much research to study their improved properties. Our purpose is limited to the study of the tightness of coronary fillings, which is considered to be the keystone of the durability of restorations. In fact, the adhesion of the material to the dental substrate plays an important role in the clinical performance of GICs [13]. In this work, we propose to carry out an in vitro study on natural teeth in order to study the sealing of coronary restorations made with a nano-filled glass ionomer cement in comparison to conventional glass ionomer cement and the influence of conditioning on microleakage.
2. MATERIALS AND METHODS
2.1. Materials
In this study, two types of glass ionomer cements were used to compare in vitro the microleakage at the interfaces:
• A conventional GIC Ketac ™ Fil Plus (3M ESPE),
• A nano-charged GIC Ketac ™ Universal (3M ESPE) (Fig. 1 and Table 1).
2.2. Methods
Forty freshly extracted, caries-free human teeth (twenty maxillary and mandibular molars and twenty premolars) were used for this study. These teeth were stored in distilled water and in a refrigerator until the operation process. These teeth were cleaned using a brush head with water and pumice powder to remove tartar, soft tissue, and other debris. Each tooth was numbered.
On each tooth, two box-type enamel-dentinal cavities were prepared: one on the mesial face and the other on the distal face. Each cavity is located at the cement-enamel junction and has the following dimension:
- Height: 3mm;
- Width: 2 mm in the vestibulo-lingual direction;
- Width: 1.5 mm in the mesiodistal direction (Fig. 2).
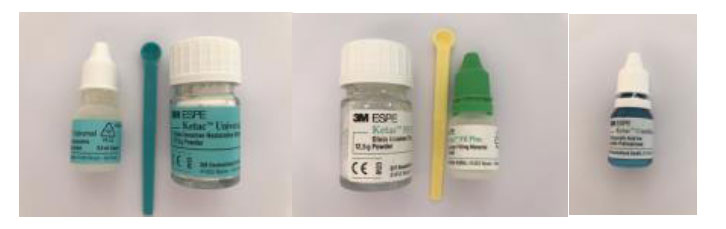
Type | Commercial Name | Manufacture | Lot Number | Composition / Teinte |
Nanocharged GICs | Ketac™ Universal | 3M ESPE | 3480409 | Oxide glass, water, copolymer of acrylic acid-malic acid, tartaric acid • A3 |
Conventional GICs | Ketac™ Fil Plus | 3M ESPE | 3695673 | Al-Ca-La fluorosilicate glass, 5% copolymer acid (acrylic and maleic acid), Polyalkenoic acid, tartaric acid, water • A3 |
Polyacrylic Acid | Ketac™ conditionner | 3M ESPE | 3302125 | • Water • Polyacrylic acid |
These measurements were verified with a periodontal probe to ensure uniformity of preparations, and the cervical angles were rounded. The cavities were prepared with a high-speed handpiece, using a round bur and a cylindrical tungsten carbide bur (diameter 1.2 and 1mm) under heavy water spray. Each bur was used to prepare five cavities; after each manipulation, the teeth have been stored in distilled water. The teeth were randomly divided into two groups of 20 teeth each: Group 1 and Group 2, as shown in Fig. (3).
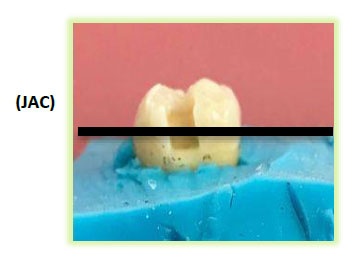
A mounted metal matrix and a matrix port were used as formwork to replace the missing wall and avoid any risk of the GIC overflowing during sealing. The preparations were gently dried with a blower and a cotton ball.
Ketac™ Universal Nanofilled GIC (3M ESPE) was prepared according to the manufacturer's recommendations. The GIC Ketac ™ Universal (3M ESPE) was inserted into the cavity with a mouth spatula. The formwork system was removed 7 minutes after filling the cavity and setting the material. The restoration was then finished and polished with medium and fine Sof-Lex™ discs (3M ESPE) and silicone tips and kept at 37° and 90% humidity for 24 h. The root tips of the teeth were sealed with a transparent orthodontic resin pellet (ORTHOSELF). Additional protection was provided by applying two coats of clear nail polish to each crown, leaving 1mm around the restoration.
The samples were stored in distilled water for 3 days at 37°C and then thermocycled in water baths. After removing them from the dye solution, the teeth were air-dried and cleaned with abrasive discs to remove all traces of the dye. The teeth were then stored in a 0.9% KCl solution.
Daily cycle: 45 min at 6° + 45 min at 60°, repeated four times in 16h at room temperature. The daily cycle was repeated for five consecutive days [14, 15]. A second application of two coats of varnish to each crown was performed. The crowns of the teeth were subsequently immersed in a 1% methylene blue solution for 48 hours at room temperature (Fig. 4).
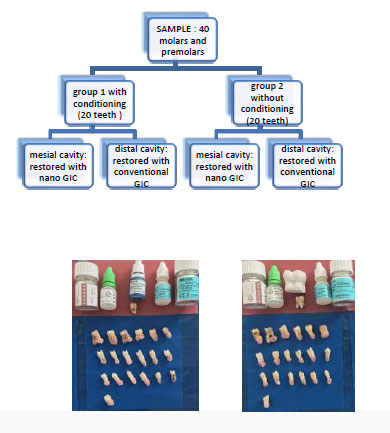
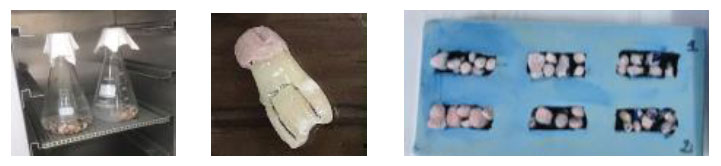
A 0.4 mm thick diamonded chain saw Isomet® (Buehler) was used at 7 rpm speed to separate the roots of each tooth from the crown and split each crown longitudinally in the mesiodistal direction at the middle of the mesial and distal restorations. After having separated the roots of each tooth from the crown, two sections were thus obtained, one vestibular and one lingual (Fig. 5).
Each section was photographed using a stereo microscope (Carl Zeiss™ STEMI 2000C ZOOM 6, 5 stereo microscope) under a magnification of 2 and with a digital camera. The degree of infiltration of methylene blue (micro infiltration) in each restoration was established according to a score; it was noted separately for the cervical wall and the axial wall. The observation was carried out separately by two observers.
The infiltration scores used at the axial wall were as follows:
- Score 0: Perfect adaptation/no penetration of the dye;
- Score 1: Infiltration affecting only the enamel.
- Score 2: Infiltration affecting enamel and dentin (without reaching the cervical wall).
- Score 3: Penetration of the dye beyond the cervical wall.
The infiltration scores used at the cervical wall were as follows:
- Score 0: Perfect adaptation/no penetration of the dye;
- Score 1: Infiltration affecting dentin only (without reaching the axial wall);
- Score 2: Penetration of the dye exceeding the axial wall (Fig. 6).
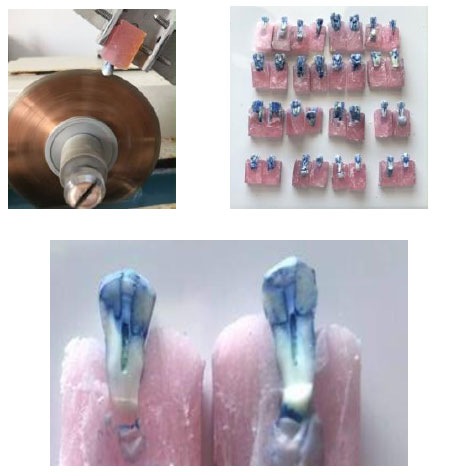
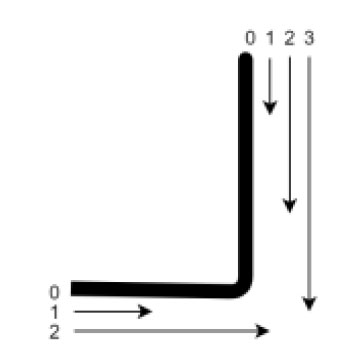
3. RESULTS AND DISCUSSION
In this work, we have studied the infiltration of a conventional GIC and a nanocharged CVI to assess their tightness at the level of the cervical wall and the axial wall of the box cavity.
The infiltration score of the two materials tested was calculated by half and not by cavity. None of the materials were found to be free from micro-leaks (Table 2).
3.1. Statistical Analysis
Chi-square test with SPSS 20.2 software was used to study the distribution of scores. The results were found to be significant if P <0.05. Microsoft office Excel 2013 was also used to carry out some numeric functions. The chi-square test showed a significant difference (P <0.05) between nanocharged GIC and conventional GIC. The nanocharged GIC presented less infiltration; it was more waterproof than the conventional GIC (P = 0.002 at the cervical level and P = 0.007 at the axial level). The chi-square test showed the conditioning operation to have no effect on the tightness since the test was not significant (P> 0.05).
A comparison of the cement and enamel wall within the same group provided an insignificant chi-square test result. So, there was no difference found between cementitious and enamel sealing (Table 4).
4. DISCUSSION
In this study, nanocharged GICs have been studied to assess their mechanical properties [16, 17], physico-chemical [18] and cariostatic properties [19, 20]. The aim of our work was to study the tightness of a conventional GIC (Ketac ™ Fil Plus) and a conventional nanocharged GIC (Ketac ™ Universal) at the level of the box cavities.
- | Axial wall | Cervical wall | ||
- | Score 2 | Score 3 | Score 1 | Score 2 |
Nanocharged GICs | 62% | 40.4% | 68% | 41.8% |
conventional GICs | 38% | 59.6% | 32% | 58.2% |
- | Axial Wall | Cervical Wall | ||
- | Score 2 | Score 3 | Score 1 | Score 2 |
Gr1: with conditioning | 46.5% | 52.8% | 58% | 46.4% |
Gr2: without conditioning | 53.5% | 47.2% | 42% | 53.6% |
• group 2: without conditioning
Scores | Axial Wall | Cervical Wall | ||
Score 2 | Score 3 | Score 1 | Score 2 | |
With conditioning | 33 41.3% |
47 58.8% |
29 36.3% |
51 63.8% |
Without conditioning | 38 47.5% |
42 52.5% |
21 26.3% |
59 73.8% |
P value | 0.426 | 0.172 |
4.1. Evaluating the Tightness of GICs
The evaluation of the tightness of a material requires the study of the factors on which this property depends, such as adhesion to calcified dental tissues, dimensional variations, the coefficient of thermal expansion, and the solubility of the material in the oral environment. Sealing is also operator-dependent [21].
Sealing is one of the most important properties of coronary restorative materials. Micro-infiltrations are the most common causes of failure of coronary restoration (Manhart et al., 2004), sensitivity, recurrence of caries, and inflammation of the pulp [22].
There are currently several methods for studying the micro-infiltration of a material (Alani and Toh, 1997) [23]. The most common and simplest methodology uses a dye as the infiltration solution (methylene blue, Fushine) [23]. It is a reliable and inexpensive technique (Raskin et al. 2001) [24]. The only downside is that the assessment is qualitative but the use of a non-parametric scale turns this technique into a semi-quantitative assessment method [25].
Our study showed that the two materials used presented micro infiltration. However, nanocharged GICs exhibited less infiltration on both the axial (enamel and dentin) and cervical (cementum) walls than conventional GIC. This is in agreement with the study by Moheet et al. [25]. A nanostructured material exhibits better properties compared to a conventional material [16]. Its properties are attributable to the nanosize of the components, which varies from 1 to 100 nm in dimension [26]. The glass-ionomer nanostructure allows excellent wetting and, therefore, excellent adaptability to the tooth surface, thus improving the chemical bond between the material and the cavity [27]. Polymerization shrinkage and coefficient of thermal expansion are lower, also improving long-term bonding to tooth structure [28].
The work of Shruthi [29] and Gorseta [30] showed the same results as our study: nanocharged and conventional GICs presented infiltration. These infiltrations are less significant for the nanocharged GIC. Nevertheless, Gorseta et al. have highlighted the effectiveness of GICs in daily dental practice [29]. Hussin's study [31] demonstrated the opposite result: conventional GICs are more airtight than nanocharged GICs, where several factors could be considered to have caused the infiltration: thermal cycling and improper handling.
Infiltration can be explained by cracks in the enamel that appear after extraction. We applied several coats of varnish before thermal cycling and infiltration with methylene blue to avoid this bias. The samples underwent several cycles of thermal cycling to simulate extreme temperatures similar to those in the oral cavity. The varying temperature produces thermal stresses at the tooth/restoration joint, which can lead to fatigue of this joint and, therefore, to infiltration [32].
4.2. Adhesion of Nanocharged GICs to Dental Walls
We chose the box cavities to study the infiltration both at the level of the cervical wall and at the level of the axial wall, thus approaching as much as possible the clinical conditions of site 2. The occlusal interface is enamel-dentinal, and the cervical interface is cemento-dentinal. Our study showed an insignificant result regarding the comparison between the axial wall (p = 0.42> 0.05) and the cervical wall (p = 0.17> 0.05). The majority of published works have studied micro-infiltrations at the level of the enamel-dentinal walls. It is the cavities of sites 1 and 3, which are most often studied.
The adhesion of GIC to the tooth is physicochemical and not mechanical; the bond is with calcium ions [33]. The chemical bond is obtained by the ionic interaction of the carboxyl groups of polyacrylic acid with the calcium of hydroxyapatite in dental tissues (COO-, Ca ++) [34]. Enamel is formed by a volume of 92% hydroxyapatite, dentin from 45 to 50%, and cement from 40%. Thus, the adhesion of GIC to enamel is superior to the adhesion to dentin, which is superior to the adhesion to cementum [35]. This would explain the greater infiltrations observed in the cervical wall.
4.3. Pre-treatment of the Cavity
In our study, the use of conditioning with polyacrylic acid did not give better results (P> 0.05). The protocol of the manufacturer of the Ketac™ Universal mentions that this step is not necessary. The nanocharged GIC can therefore be used without packaging, thus saving time and making handling easier. The free orthophosphoric acid contained in these glass ionomers is sufficient to dissolve the layer of dentin slurry [36]. Pretreatment (conditioning) of enamel and dentin with polyacrylic acid (20s, rinsing, drying) improves the adhesion of GIC.
4.4. Product Handling
The use of the powder-liquid version for the two materials (manual mixing) allowed us to draw the following observations:
• The handling of nanocharged GICs is more difficult than conventional GIC.
• The setting time of the nano-charged GIC is shorter than the conventional GIC.
Manual mixing requires strict adherence to the protocol and dosage mentioned by the manufacturer. The downside to this type of mixture is that it is operator-dependent. Improper handling of the material by the operator can cause poor marginal adaptation and, therefore, micro-infiltration. An inconsistent mixture of powder and liquid can result in a material that is either too liquid or too hard that will adhere poorly to calcified dental tissue. The presence of moisture when handling the material can also promote micro-infiltration [31].
Manual powder/liquid mixing can promote the incorporation of air bubbles inside the seal that would cause surface defects. Hussin et al. [31] explained the difficulty of manual manipulation of nanofilled GICs by the absence of tartaric acid because it induces a latency period in the setting process followed by an increase in viscosity. Thus, tartaric acid has been shown to have a dual effect on the setting, first inhibiting gelation and then accelerating it [31].
De Caluwé et al. [37] have shown that nanofilled GICs exhibit shorter setting time, better compressive strength and modulus of elasticity. Decreasing the setting time of direct restorative materials also allows easier handling and reduced treatment time, benefiting both the clinician and the patient [38].
Conventional GICs are not water stable. They are very sensitive to moisture during setting [39], hence they need to be protected with a varnish [40]. Consequently, the application of a coat of varnish would result in less infiltration. Nanocharged GICs are less sensitive to humidity according to some studies [40], so the application of varnish is not essential [40]. In our study, we did not use the varnish for both types of CVI to have less bias and the same conditions.
4.5. Finishing the Obturation with Nanocharged GIC
Finishing of the filling is a step that should not be overlooked. A polished restoration is more aesthetic and more biocompatible (does not promote the retention of dental plaque) [41]. Nanoparticles provide a better finish [13]. The restoration of a polished GIC is smoother and more aesthetic. In this, fluoride release and adhesion to enamel and dentin are better [26]; also, lower sensitivity to humidity and dehydration have been observed [39]. Clinically, removal of the outermost surface by finishing-polishing procedures would tend to make nanofilled GICs more resistant to biodegradation and, therefore, more aesthetically stable [41].
4.6. The Limitations of the Study
The sample size was one of the limitations of this in vitro study because using a statistically incorrect sample size may lead to inadequate results in both clinical and laboratory studies, and result in time loss, cost, and ethical problems. A larger sample is preferable [42]. Further, a single brand of GIC (3M ESPE) was used for both nanocharged GIC and conventional GIC. Comparing different brands of GIC would have been more interesting. Also, GIC modified by the addition of resin was not compared to nanocharged GIC.
CONCLUSION
Further in vitro studies and research are needed to improve the sealing and handling of nanocharged GICs. In vivo investigations are needed to clinically evaluate the short and long-term efficacy of these materials.
The use of nanoparticles in restorative dentistry is the way of the future. The next generations of materials will be nanocharged.
LIST OF ABBREVIATIONS
GICs | = Glass-ionomer Cements |
KCl | = Potassium Chloride |
CVI | = Cerebral Visual Impairment |
ESPE | = Electro-sensitive Protective Equipment |
ETHICS APPROVAL AND CONSENT TO PARTICIPATE
The ethics committie of the research project of the Faculty of Dentistry of the University of Monastir has reviewed and calculated the project
HUMAN AND ANIMAL RIGHTS
No animals were used in this research. All procedures performed in studies involving human participants were in accordance with the ethical standards of institutional and/or research committee and with the 1964 Declaration of Helsinki.
CONSENT FOR PUBLICATION
A written informed consent has been taken from the patients/volunteers before their teeth extraction for orthodontic reasons.
AVAILABILITY OF DATA AND MATERIAL
The data and supportive information are available within the article.
CONFLICT OF INTEREST
The authors declare no conflict of interest.
ACKNOWLEDGEMENTS
Declared none.