All published articles of this journal are available on ScienceDirect.
Temperature Rise at the Pulp-Dentin Junction for a Multi-Layered Composite Restoration using the Finite Element Method
Abstract
Objectives:
During the light-curing process of composite restoration, excessive heat can be produced, which can potentially lead to pulp necrosis (death). In this study, we aimed, based on the Finite Element Method (FEM), to assess the risk of pulp damage during the light-curing process by investigating the influence of light-curing devices, under various irradiation regimes, on the temperature increase at the pulp-dentin junction, during a one-layer or multi-layered deep composite restoration.
Methods:
A Three-dimensional finite element method model of typical geometry and material properties, as commonly reported in the literature, was employed in COMSOL Multiphysics simulations in order to determine the temperature increase in the pulp. Various combinations of light intensities, durations, and irradiation regimes were investigated for the two cases, of shallow and deep multi-layered composite restoration.
Results:
Results of light-curing composite resins within enamel; indicate that the temperature rise during the curing process was within the safety margins. Results of light-curing composite resin restorations closer to the pulp with thin remaining dentin, indicate a temperature increase that could be sufficient to cause thermal injury in the pulp. Modulating the light output marginally, reduced the temperature rise while reducing the intensity and increasing the curing duration which was consistently more effective in this respect.
Conclusion:
The results clearly demonstrate that with currently adopted standard procedures, there exists a risk of thermal injury during multi-layered composite restorations with thin remaining dentin; it is thus important to establish appropriate curing regimes that would lead to minimal temperature increase during deep composite restorations and hence reduce the risk of thermal injury to the pulp.
1. INTRODUCTION
Light-cured composite resins have been widely used and have been the standard of dental composites since the beginning of the 21st century [1]. With the technological advances in this field, various new light-curing units (LCUs) have been introduced, with higher energy output, aiming to decrease the polymerization period; however, concerns regarding the potential risks of pulp thermal injury from the short-time high energy exposure of such devices have been raised [2].
An in vivo study on rhesus monkeys is often cited [3], where the researchers Zach and Cohen carried out tests using a heated soldering iron placed on the surface of the tooth to determine the effects of thermal irritation in the pulp tissue. It was found that a temperature increase of 5.5°C in the pulp caused approximately 15% of the cases, irreversible damage to the pulp tissue, while a rise of 11.1°C caused about 60% of the cases, irreparable damage to the pulp tissue.
Whereas in a recent study on human teeth [4], where the thermal stimulus was administrated using a different variation of intensity and duration than the previous study, a temperature increase of approximately 11.2°C did not cause any irreversible damage to the pulp tissue. Hence, it can be hypothesized that this variation, allowing a more gradual temperature increase, may raise the temperature threshold for pulpal injury.
An essential factor for the temperature threshold beyond which pulp tissue is injured, was not taken into consideration in the previous two studies is the residual dentin thickness: this can be small at the spot where the light-curing process is being carried out, thus, not offering sufficient shielding to the pulp tissue [5]. However, thermal damage is not limited to the pulp tissue alone; a high-temperature increase on the outer root surface can also result in damage to the alveolar bone, the root cementum, and the periodontal ligament [6, 7].
Considering the universal use of LCUs in dental restorations and the fact that that a high percentage of the light energy is converted to heat; numerous works [7-14] have experimentally investigated the temperature increase during the light-curing process, taking into account the effects of light intensity, composite type and irradiation time, in order to assess the risk of thermal irritation in the pulp tissue. The results indicate a temperature increase that might adversely affect the pulp is possible.
Other researchers have used the Finite Element Method (FEM) [15-18] to simulate the thermal excursion during the light-curing process in dental restorations and to determine the temperature increase in the pulp. Many factors can affect the temperature increase, such as the LCU's intensity and wavelength, exposure duration, composite properties (density, heat capacity, thermalconductivity), composite depth, and different tooth types. In this respect, FEM affords a flexible tool, able to simulate various processes, taking the variation of multiple parameters into account for each case study and with a clear deployment time advantage, compared to experimental investigation.
Some research teams [19, 20] have employed pulse-width-modulated (PWM) LCUs to investigate the effects of intensity and cure time on the temperature change of the composite and dentin; it was found that a constant output LCU of lower intensity can minimize thermal transfer, which, in turn, decreases the risk of pulp damage.
In this study, we investigate the effects that LCUs of various light outputs have on the temperature increase at the Pulp-Dentine Junction (PDJ) during a one-layer of composite resin restoration, representing shallow cavity, and multi-layered composite curing, representing deep restoration closer to the pulp with thin remaining dentin, using a FEM model to simulate the temperature rise, in order to assess the risk of pulp thermal injury during the light-curing process.
2. MATERIALS AND METHODS
2.1. Materials Properties
For the FEM model to accurately represent the temperature increase at the PDJ, it is necessary to determine the standard material properties for each component, likedensity (rho), thermal conductivity (k), and heat capacity at constant pressure (Cp). Values used in this work were based on data found in [17, 21, 22] and are listed in Table 1.
Material | Density (kg m-3) | Thermal Conductivity (W m-1K-1) | Heat Capacity at Constant Pressure (J kg-1K-1) |
---|---|---|---|
Enamel | 2800 [22, 23] | 0.93 [18, 23] | 750 [18, 22, 23] |
Dentine | 2000 [22, 23] | 0.63 [18, 22, 23] | 1170 [18, 23] |
Pulp | 1000 [18, 22, 23] | 0.59 [18] | 4200 [18, 22] |
Cortical Bone | 1300 [23] | 0.58 [23] | 1840 [23] |
Composite | 2000 [22, 23] | 1.26 [22] | 820 [22, 23] |
Air(a) | rho(pA,T) | k(T) | Cp(T) |
(a) Air properties changes with the variation in Atmospheric pressure (pA) and Temperature (T) and are computed by COMSOL material library [24] |
2.2. Tooth Geometry
The FEM simulation was based on an approximated three-dimensional model of a Mandibular First Molar tooth, with a cervico-occlusal crown length of 7.5mm, root length of 14mm, enamel thickness varying from 1.3-3mm with 1.3 being at the cementoenamel junction and 3mm at the cusps, and dentin thickness of approximately 3.6mm from the enamel to the PDJ at the centre of the tooth [ 23-25], with overall two approximated model versions, were designed (Sketchup 3D [26]) and employed for the shallow composite restoration (Fig. 1A), and for the multi-layered composite restoration simulation (Fig. 1B).
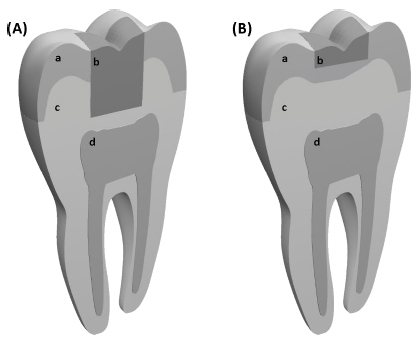
2.3. FEM Modelling
2.3.1. Heat Source
A time-dependent FEM model was developed using COMSOL Multiphysics, version 5.5 [27]. Three-dimensional geometry was used and several boundary conditions were applied. The approximations made consisted of assuming zero reflectance by the curing compound as well as neglecting the heat produced by the exothermal curing reaction. Research has shown that LCUs can generate an enormous amount of heat [28] and that the heat generated was influenced more by the LCU than the exothermal curing reaction [2]. Therefore, the thermal power input per unit area due to the light source was, in this respect, assumed equal to the incident light intensity, with the LCU considered, in effect, as a heat source.
2.3.2. Temperature Boundaries
The boundaries between the tooth and the surrounding material are essential to the FEM model. The tooth temperature was set to 35° Celsius, which is the average temperature of a tooth in the mouth [29], while the temperature of the space surrounding the tooth was set to 28° C, slightly higher than room temperature. The heat flux (Heat dissipation factor) of the air was taken equal to 12 (W m-1k-1) [17].
2.3.3. Tooth Temperature increase with a shallow one-layer composite restoration
To investigate the risks of reaching a hazardous temperature increase at the PDJ during a shallow composite restoration within the enamel, multiple time-dependent intensity variations were applied to the heat source as seen in Eq.1, where IT is the total intensity divided by curing duration T, multiplied by the wave function W(t). Since the composite curing process depends primarily on the total light energy and not the thermal energy [30-32], and the energy needed to cure the composite is usually between 16 - 24 J cm-2 depending on the composite type and shade [33], 24 J cm-2 was chosen as it represents the highest possible dose needed to cure the composite, and this total dose remained constant throughout all the configurations (Eq.1).
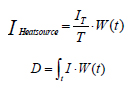
Where I(W.cm-2) is the light source’s intensity amplitude (assumed constant) and W(t) is a modulating function taking values from 0 to 1 as seen in Fig. (2). In the case of a saw-tooth variation of period T, for example:

The dose D(J.cm-2) within a period T will be in this case (Eq.2):

This is 50% of the dose under constant irradiation (IT)
A very small percentage of this energy per unit area will be employed in polymerizing the compound and can be ignored. Almost all of this energy per unit area can be assumed to contribute to the temperature rise of the target tooth + composite.
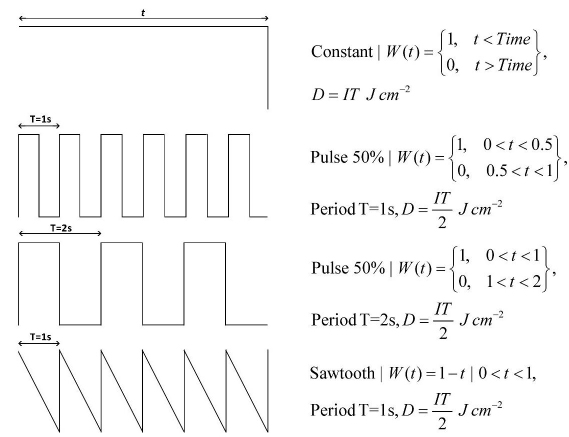
In the following, we have compared the temperature rise under different exposure regimes that result in an equal dose. Assuming the time under constant irradiation required for the dose leading to complete curing to be equal to tcc, The time under saw-tooth irradiation will be, according to the above, tcs = 2tcc and following the same reasoning, the time for achieving the complete curing dosage under a square wave of 50% duty cycle will be again tcp1_1 = 2tcc
Thus, to compare the temperature rise on an equal (complete curing) dose basis, the simulation based on saw-tooth irradiance as well as that on a square wave of 50% duty cycle, assumed an irradiation time, double that of the constant irradiation. Also, we have investigated the variation in temperature rise by increasing the on and off duration during the 50% duty cycle pulsed regime.
2.3.4. Tooth Temperature Increase with a Deep Multi-layered Composite Restoration
To evaluate the risk of pulp damage when the preparation distance to the pulp is small, and a layering technique is being employed, having to repeatedly cure the multiple layers of composite resin, the same temperature boundaries from the shallow restoration model were applied to a deep multi-layered composite restoration model. Since the composite resin material properties that were used are for a layered restoration, and research has shown that having incremental layered restoration can reduce shrinkage stress [33] and that thickness of the composite resin is a significant factor in the polymerization process [34], in order to allow the simulations to accurately represent clinical composite restorations, the composite was incrementally placed into four approximately 2mm layers, with each layer getting cured with the same curing duration and energy as the shallow composite restoration (2mm). Under the assumption that the operator will need 60 seconds to prepare the composite resin before curing; thus, after curing each layer, a duration of 60seconds was allowed before adding another layer. The maximum temperature rise was recorded for each layer.
3. RESULTS
3.1. Tooth Temperature Increase with a Shallow One-layer Composite Restoration
The total dose of 24 J cm-2 remained constant throughout all the configurations. A standard constant LCU light output (I=1200 mW cm-2, t=20s) produced a temperature increase of 6.56° C degrees at the PDJ from the starting temperature of 35° C as shown in Table 2, with (Fig. 3A) showing the temperature rise over time with the inset illustrating the temperature profile of the tooth at t=40s, with the temperature being higher on the cusp because of the very close contact to the curing light and because of the difference in the material composition, the enamel temperature rises as well. By increasing the intensity to (I=4800 mW cm-2, t=5s), the temperature rose to 6.68° C. Although decreasing the intensity and doubling the time did reduce the temperature, there was no big temperature difference between the constant intensity configurations.
Wave Function | Time (s)(a) | Intensity (mW cm-2)(b) | Maximum Temperature rise (°C)(c) |
---|---|---|---|
![]() |
5 | 4800 | 6.68 |
10 | 2400 | 6.64 | |
20 | 1200 | 6.56 | |
40 | 600 | 6.27 | |
![]() |
10 | 4800 | 6.38 |
20 | 2400 | 6.3 | |
40 | 1200 | 6.03 | |
![]() |
10 | 4800 | 6.53 |
20 | 2400 | 6.43 | |
40 | 1200 | 6.14 | |
![]() |
10 | 4800 | 6.66 |
20 | 2400 | 6.58 | |
40 | 1200 | 6.27 | |
Note that the total dose for all the configurations is constant D = 24 J cm-2 a) Total time the Wave function was active T(Curing Duration) b) Constant LCU Intensity c) Temperature increase at the PDJ from base temperature (35°C) |
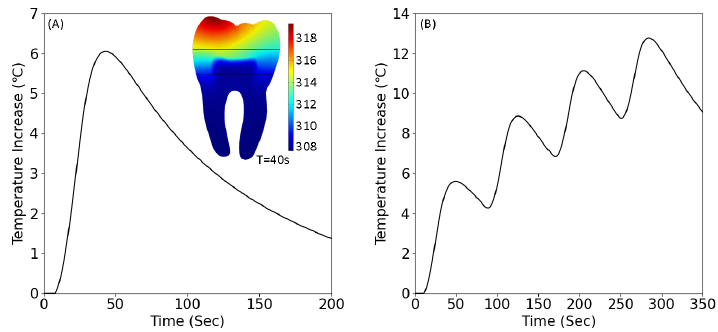
When modulating the light output (Pulse wave, 50% duty cycle, T=1s) with (I=4800mW cm-2, t= 10s), a temperature rise of 6.38° C was recorded at the PDJ. Reducing the intensity (I=1200 mW cm-2, t= 40s) produced a lower temperature raise of 6.03° C, which is the lowest recorded temperature rise among all configurations. Whereas increasing the wave time period (Pulse wave, 50% duty cycle, T=2s) resulted in a slightly higher temperature increase with (I=4800 mW cm-2, t= 10s) producing 6.53° C.
Modulating the light output in a sawtooth wave form resulted in a similar temperature increase to the constant configurations, with (I= 4800 mW cm-2, t= 10s) having a temperature increase of 6.66° C, and reducing the intensity (I= 1200 mW cm-2, t= 40s) produced a temperature increase of 6.27° C.
The simulated temperature increases for each configuration with different intensities and the same total doses are shown in (Figs. 4A, B) indicates the temperature increase in each configuration for different curing durations.
3.2. Tooth Temperature increase with a deep Multi-layered composite restoration
The FEM model PDJ temperature rise results for a deep layered composite restoration under different configurations are shown in Table 3. (Fig. 3B) illustrates the temperature increase over time for a constant light output with t=20s. As discussed in the preceding section, a total dose of 24 J cm-2 was used for curing each 2mm composite layer. The standard constant light output (I=1200mW cm-2, t=20s) reached a maximum temperature increase of 12.94° Cfrom the base temperature 35° C, and by increasing the intensity (I=4800mW cm-2, t=5s), the temperature rise increased to 14.38°C, while decreasing the intensity (I=600 mW cm-2, t=40s) lowered the maximum temperature rise to 11.30° C.
Wave Function | Time (s)(a) | Intensity (mW cm-2)(b) | 1st layer Temp. rise(°C)(c) | 2nd layer Temp. rise(°C)(c) | 3rd layer Temp. rise(°C)(c) | 4th layer Temp. rise(°C)(c) |
---|---|---|---|---|---|---|
![]() |
5 | 4800 | 5.93 | 9.81 | 12.43 | 14.38 |
10 | 2400 | 5.95 | 9.65 | 12.12 | 13.94 | |
20 | 1200 | 5.73 | 9.14 | 11.14 | 12.94 | |
40 | 600 | 5.44 | 8.28 | 10.03 | 11.30 | |
![]() |
10 | 4800 | 5.57 | 9.14 | 11.52 | 13.26 |
20 | 2400 | 5.47 | 8.74 | 10.85 | 12.36 | |
40 | 1200 | 5.19 | 7.92 | 9.59 | 10.79 | |
![]() |
10 | 4800 | 5.77 | 9.41 | 11.83 | 13.59 |
20 | 2400 | 5.63 | 8.96 | 11.11 | 12.68 | |
40 | 1200 | 5.31 | 8.1 | 9.82 | 11.04 | |
![]() |
10 | 4800 | 5.83 | 9.57 | 12.07 | 13.89 |
20 | 2400 | 5.73 | 9.13 | 11.33 | 12.95 | |
40 | 1200 | 5.44 | 8.29 | 10.04 | 11.30 | |
Note that the total dose for each layer in all the configurations is constant D = 24 J cm-2 a) Total time the Wave function was active T(Curing Duration) b) Constant LCU Intensity I c) Temperature increase at the PDJ from base temperature (35 C) |
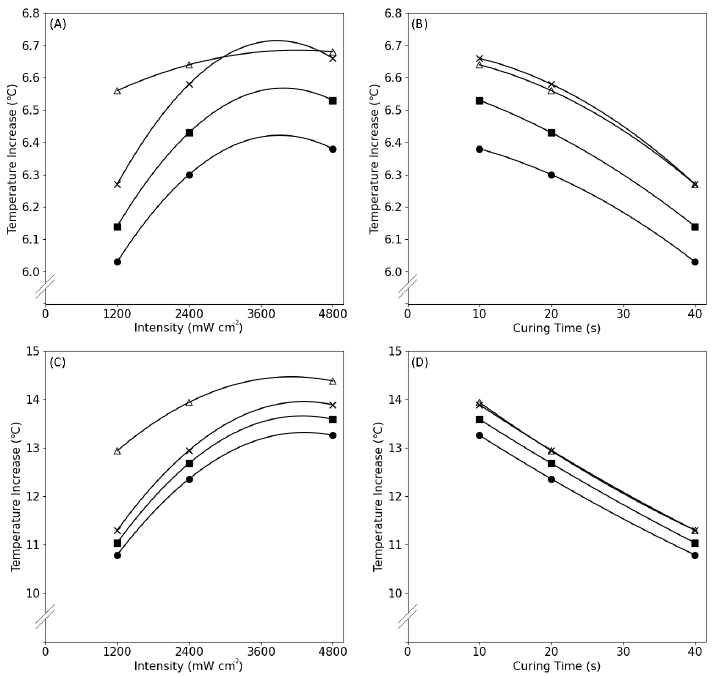
Modulating the light output (Pulse wave, 50% duty cycle, T=1s) with (I=4800 mW cm-2, t= 10s) a temperature rise of 13.26° C was recorded at the PDJ. Reducing the intensity (I=1200 mW cm-2, t= 40s) produced a lower temperature rise of 10.79° C, which is the lowest recorded temperature from all the configurations. Increasing the wave time period (Pulse wave, 50% duty cycle, T=2s) resulted in slightly higher temperature increases from the previous pulse configuration with (I=4800 mW cm-2, t= 10s) producing 13.59° C, and (I=1200 mW cm-2, t= 40s) resulted in a temperature increase of 11.04.
Modulating the light output to a saw-tooth waveform resulted in a similar temperature increase to the constant configurations when the intensity was the same, with (I= 4800 mW cm-2, t= 10s) having a temperature increase of 13.89° C, and reducing the intensity (I= 1200 mW cm-2, t= 40s) produced a temperature increase of 11.30° C. (Fig. 4C) illustrates the effects of light intensity on the temperature rise, and (Fig. 4D) shows the impact of curing duration on the temperature rise.
4. DISCUSSION
4.1. Tooth Temperature Increase with a Shallow One-layer Composite Restoration
In this study, we investigated the influence of different light output configurations on the temperature increase at the PDJ. The temperature increase results of the shallow composite restoration model for thirteen different configurations are shown in Table 2.
While there isn’t a universally accepted temperature that would cause irreversible damage to the pulp, and as this varies for each study case [3, 4], minimizing the temperature rise is desirable; hence, it was assumed that a temperature increase higher than 7.5 °C [3] from 35 °C [29] was considered hazardous. Therefore, the results show that for a composite restoration in a shallow cavity restored by one layer of composite only, all configurations, including the standard constant light output which is commonly used doesn’t reach that threshold. Additionally, raising the intensity increases the temperature at the PDJ, as shown in Fig. (3A), and expanding the curing duration reduces the temperature rise almost linearly, as shown in Fig. (3B). While a very long curing duration can potentially lower the temperature rise, it is not a practical solution as the operator has to hold the LCU for an extended period steadily. A compromise between the intensity and curing duration is the optimal solution toward a low-temperature rise.
Modulating the light output did result in a slight variation on the temperature rise and most configurations had similar temperature increases. Since none of the configurations resulted in a hazardous temperature with the highest recorded temperature rise being 6.68° C, the results indicate that for a small shallow composite restoration, the risk of pulpal damage is minimal. Additionally, Fig. (2A) shows the temperature increase at the PDJ overtime for constant exposure reached a maximum after the curing duration because of the distance from the composite to the pulp, thus allowing the possibility to cool the tooth down using cold air after curing, to decrease the temperature rise even further.
4.2. Tooth Temperature Increase with a Deep Multi-layered Composite Restoration
Although in the shallow composite restoration, the standard constant light output did not produce a hazardous temperature rise, in a deep cavity where the composite is incrementally placed, the temperature rise exceeded 7.5° C as shown in Table 3. Modulating the light output to pulse wave for a period of 1 second, reduced the temperature, resulting in the lowest temperature rise recorded (10.79° C), and increasing the time period to 2 seconds increased the temperature. Having a saw-tooth modulated light output, resulted in the same temperature rise as the constant light output of the same intensity.
The assumption that there is 60 seconds pause for preparation may actually be overestimating the time necessary and it becomes obvious that for composite restorations that is close to the pulp, there exists a risk of damaging the pulp; therefore, an increase to the preparation period or intermediate cooling of the tooth using cold air could limit the overall temperature rise as indicated in Fig. (2B), where the temperature decreases until another layer is applied and cured.
A study on high irradiance curing [35] demonstrated that high irradiance LCUs are more likely to under-cure the composite. Results presented here further indicate that increasing the intensity and having a short curing duration will also result in hazardous temperature levels (c.f. (Fig. 3C)), further strengthening the argument for longer curing times at lower intensities (c.f. (Fig. 3D)).
There are some factors whose inclusion may further enhance the accuracy and versatility of the FEM model presented here, such as the curing light distance and angle [36], cavity diameter and, cavity location [37], assumed here to be constant. Another factor is the wavelength of the composite polymerization [37, 38], the variation of which was not investigated in this study, on the assumptions that it wont significantly change the end results.
CONCLUSION
Results indicate that an incrementally placed composite restoration in a deep cavity with minimum dentin remaining might produce a temperature increase that can be sufficient to cause thermal injury to the pulp. The repeated curing of layers increases the temperature rise. It is thus important to establish appropriate curing protocols to minimize the temperature increase during deep cavity restorations using layered composite restorations that include modulating the curing light intensity while increasing the standard curing duration and an allowance for a cooling-down period to reduce the risk of thermal injury to the pulp.
ETHICS APPROVAL AND CONSENT TO PARTICIPATE
Not applicable.
HUMAN AND ANIMAL RIGHTS
Not applicable.
CONSENT FOR PUBLICATION
Not applicable.
AVAILIBILITY OF DATA AND MATERIALS
Not applicable.
FUNDING
This work was supported by Ajman University, United Arab Emirates, under grant agreement (2019-IDG-DEN-1).
CONFLICT OF INTEREST
The authors declare that they have no competing interests.
ACKNOWLEDGEMENTS
The authors thank Mr. Ahmed Ali Abdo for his assistance in dental research.