All published articles of this journal are available on ScienceDirect.
Indonesian Strain of Lactobacillus reuteri Probiotic Reduces the Initial Biofilm Colonization
Abstract
Background:
The benefits of probiotics for human health have long been proven. Probiotic Lactobacillus reuteri, can produce a beneficial broad-spectrum antibacterial compound called reuterin by metabolizing glycerol.
Objective:
The aim of the study was to investigate the effect of the Indonesian strain of L. reuteri LC382415 on mono- and dual-species Streptococcus mutans and Streptococcus sanguinis biofilms in vitro.
Methods:
Streptococcus mutans and S. sanguinis were cultured in BHI broth. Lactobacillus reuteri LC382415 was inoculated on MRS agar. The different concentrations effect of L. reuteri (1×104, 1×106, and 1×108 CFU/mL) with and without glycerol supplementation on microbial biofilms were examined using a biofilm assay after incubation for 1,3,6, and 24-h. The biofilm mass optical density was measured with a microplate spectrophotometer at 490 nm. Chlorhexidine gluconate (0.2%) was used as a positive control, and wells without treatment were used as negative controls.
Results:
A significant reduction in mono- and dual-species S. mutans and S. sanguinis biofilm formation was observed after treatment with all concentrations of L.reuteri and after all incubation periods (p<0.05) with or without glycerol supplementation. The concentration of 1×104 CFU/mL after 3-h incubation was the most effective in inhibiting biofilm formation, with 87.8% S. mutans, 95.9% S. sanguinis, and 80.4% dual-species biofilm reduction compared to the negative control (p<0.05).
Conclusion:
The Indonesian strain of L. reuteri effectively reduces mono- and dual-species S.mutans and S. sanguinis biofilms. This suggests that it may be useful in preventing biofilm formation in oral cavities. Future studies on the mechanism of action of this active component are warranted.
1. INTRODUCTION
Oral health issues in Indonesia still require meticulous attention on the part of dentists and the population [1]. Dental caries is one of the prevalent oral diseases, which is probably caused due to poor oral health [2]. According to the 2018 Indonesian National Report on Basic Health Research (Riskesdas), 57.6% of Indonesians have oral health problems, including dental caries [3]. Caries frequently occurs in children and is the main aetiology of adult tooth loss [4]. It is a slowly developing chronic disease caused by an ecological imbalance between tooth minerals and plaque accumulation [5, 6]. Some oral bacteria produce lactic acid by carbohydrate fermentation, which dissolves the crystal hydroxyapatites in the tooth structure, causing dental caries [7]. Gram-positive cocci, most commonly Streptococcus mutans and S. sanguinis, are the initial colonizers forming oral biofilms [6].
Streptococcus mutans is a member of the commensal microbes in the oral cavity. However, it becomes pathogenic in acidic environments due to its ability to ferment some carbohydrates, produce large amounts of acid, and participate in biofilm formation [8]. Its capacity to cause a disease depends on its ability to adhere to the tooth surface through extracellular glucan polymerization [9]. In the late initial stage of oral biofilm formation, the tooth surface is coated with a pellicle, followed by the adhesion of other species of bacteria, such as S. sanguinis [10, 11], which in turn, enables other bacteria to adhere to the pellicle and form a biofilm [10]. Therefore, a therapeutic agent that targets the biofilm in this stage might be effective in preventing dental caries [12].
Nowadays, due to the increasing resistance of pathogenic bacteria to antibiotics, genetic engineering studies are being conducted [13, 14]. Commensal and pathogenic bacteria have been modified into probiotic bacteria to eliminate many pathogenic conditions from the human body. Although such applications have been used in systemic conditions for some time, their use in oral diseases is relatively new [13].
The benefits of probiotics for human health have long been proven [15]. For example, Lactobacillus reuteri produces reuterin (3-hydroxypropionaldehyde; 3-HPA) by metabolizing glycerol. Reuterin is an anti-pathogenic compound that exerts activity against fungi and bacteria, such as S. mutans [16, 17]. Lactobacillus reuteri also produces substances that can suppress or stimulate the immune response, including the modulation of pro-inflammatory cytokines [17, 18]. Research suggests that L. reuteri probiotics can be used as prophylaxis against caries [19] due to their antimicrobial and anti-inflammatory properties [18, 20]. However, the activity of L. reuteri depends on the strain [17].
Previously, the first Indonesian strain of L. reuteri, obtained from GenBank with accession number LC382415, was found in the saliva of Indonesian healthy young adults [21]. However, its mechanism of action in dental caries prevention has not yet been reported. Thus, this study aimed to investigate the effect of the Indonesian strain of L. reuteri LC382415 on initial mono- and dual-species S. mutans and S. sanguinis biofilm colonization with and without glycerol supplementation.
2. MATERIALS AND METHODS
2.1. Bacterial Culture
Streptococcus mutans (ATCC® 25175™) and S. sanguinis (ATCC® 10556™) were cultured in brain heart infusion broth in anaerobic conditions at 37°C for 24 h. Lactobacillus reuteri LC382415 [21] was cultured in de Man, Rogosa, and Sharpe broth in anaerobic conditions at 37°C for 72 h. For L. reuteri with glycerol supplementation, glycerol (250 mM) was added to L. reuteri suspensions.
2.2. Biofilm Assay
Biofilm formation was analysed using a 96-well microtiter plate-based biofilm assay. The biofilm mass Optical Density (OD) of S. mutans and S. sanguinis cultures was measured. The bacteria were diluted until they reached 1.5 × 108 CFU/µL, equivalent to 0.5 McFarland, and homogenized with a vortexer. The cultures were incubated in a 96-well plate in anaerobic conditions at 37°C for 48 h. The well plate was rinsed with phosphate-buffered saline with no supernatant from the cultures.
Lactobacillus reuteri cultures in different concentrations (1 × 104, 1 × 106, and 1 × 108 CFU/mL) with and without glycerol supplementation were distributed in the well plate. Chlorhexidine gluconate (0.2%) was used as a positive control, and wells without treatment were used as negative controls. The biofilms were examined after incubation in anaerobic conditions for 1, 3, 6, and 24 h.
Crystal violet (0.5% w/v) was added in all wells, incubated for 15 min, and then removed. Absolute ethanol (200 µL) was also added in all wells, and the extraction of crystal violet from the wells was measured as biofilm mass. Absorbance measurements were performed using a microplate reader at a wavelength of 490 nm. All treatments were applied in triplicate.
2.3. Statistical Analysis
Parametric test was performed in data analysis. The Kolmogorov-Smirnov test was used to check the normality of the data. One-way analysis of variance and post hoc tests were performed to determine the significant value between categories (p < 0.05) (SPSS version 25, IBM, USA).
3. RESULTS
A reduction in mono-species S. mutans biofilm was observed after treatment with all L. reuteri LC382415 concentrations without glycerol supplementation and after all incubation periods compared to the negative control. OD measurements showed that the S. mutans mono-biofilm was drastically reduced with the L. reuteri LC382415 concentration of 1 × 104 CFU/mL after 1 h compared to the negative control and the other concentrations (p < 0.05). A reduction compared to the negative control was also observed with L. reuteri LC382415 concentrations of 1 × 104 and 1 × 106 CFU/mL after 3 h (p < 0.05). The S. mutans biofilm was reduced with L. reuteri LC382415 concentrations of 1 × 104, 1 × 106, and 1 × 108 CFU/mL by 56.5%, 55.3%, and 50.4%, respectively, compared to the negative control after incubation for 6 h (p < 0.05) and was further reduced by 82.2%, 81.9%, and 81.3%, respectively, after incubation for 24 h (p < 0.05). Fig. (1) shows the co-cultured L. reuteri LC382415 and S. mutans biofilm mass OD after incubation for 1, 3, 6, and 24 h without glycerol supplementation.
The presence of L. reuteri LC382415 without glycerol supplementation also reduced the mono-species S. sanguinis biofilm with all concentrations and after all incubation periods compared to the negative control.
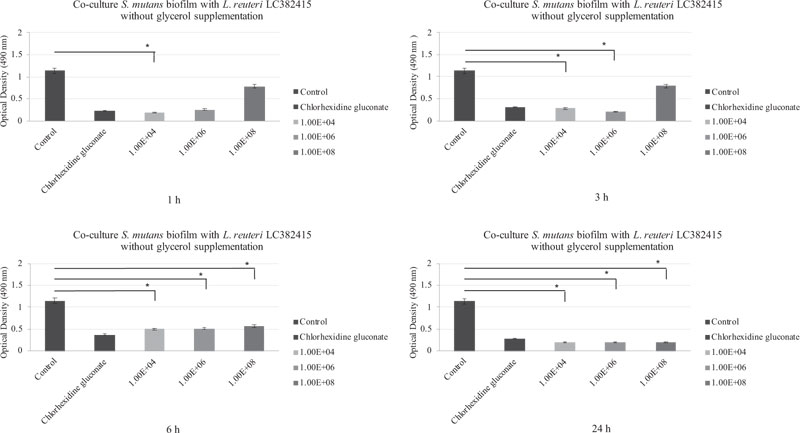
The biofilm was drastically reduced compared to the negative control with L. reuteri LC382415 concentrations of 1 × 104 and 1 × 108 CFU/mL after incubation for 1 h (p < 0.05). A significant reduction compared to the negative control was also observed with L. reuteri LC382415 concentrations of 1 × 104 and 1 × 106 CFU/mL after incubation for 3 h (p < 0.05) and a concentration of 1 × 108 CFU/mL after incubation for 6 h (p < 0.05). The S. sanguinis biofilm was reduced with L. reuteri LC382415 concentrations of 1 × 104, 1 × 106, and 1 × 108 CFU/mL by 94.8%, 98.1%, and 94.8%, respectively, compared to the negative control after incubation for 24 h. Fig. (2) shows the co-cultured L. reuteri LC382415 and S. sanguinis biofilm mass OD after incubation for 1, 3, 6, and 24 h without glycerol supplementation.
A significant reduction compared to the negative control was also observed in the dual-species S. mutans and S. sanguinis biofilm after treatment with all concentrations of L. reuteri LC382415 without glycerol supplementation and after all incubation periods (p < 0.05). Fig. (3) shows the co-cultured L. reuteri LC 382415 and dual-species biofilm mass OD after incubation for 1, 3, 6, and 24 h without glycerol supplementation.
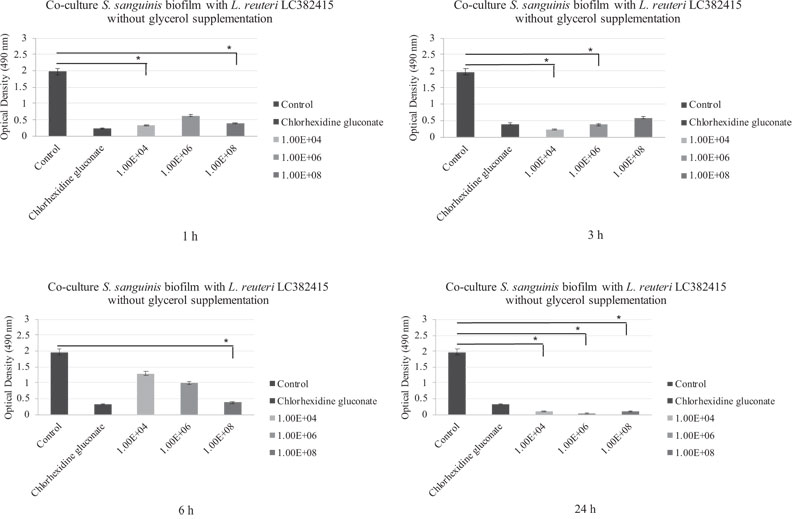
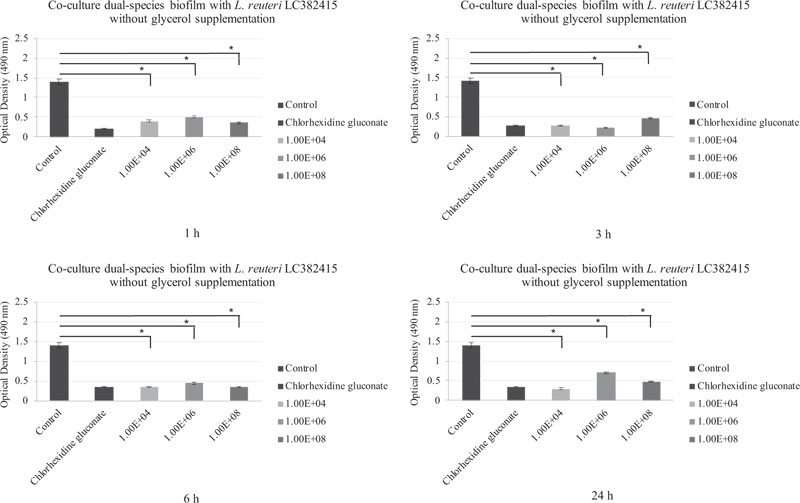
The mono-species S. mutans and S. sanguinis biofilms were also significantly reduced compared to the negative control after treatment with almost all concentrations of L. reuteri LC382415 with glycerol supplementation after all incubation periods (p < 0.05). The co-cultured L. reuteri LC382415 and mono-species S. mutans and S. sanguinis biofilm mass ODs after incubation for 1, 3, 6, and 24 h with glycerol supplementation are shown in Figs. (4) and (5), respectively.
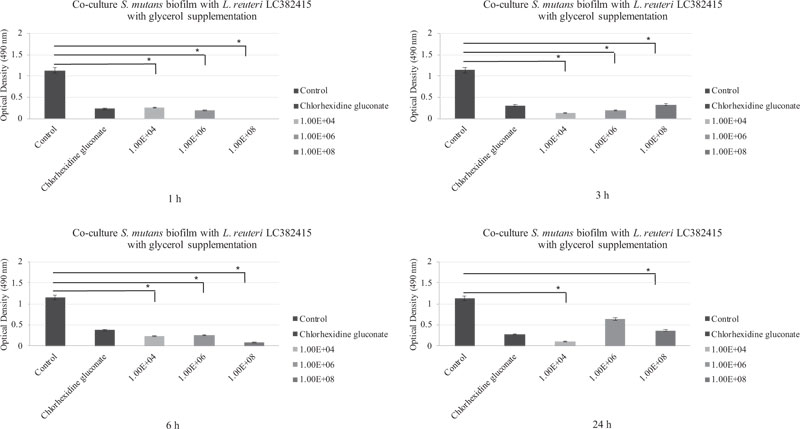
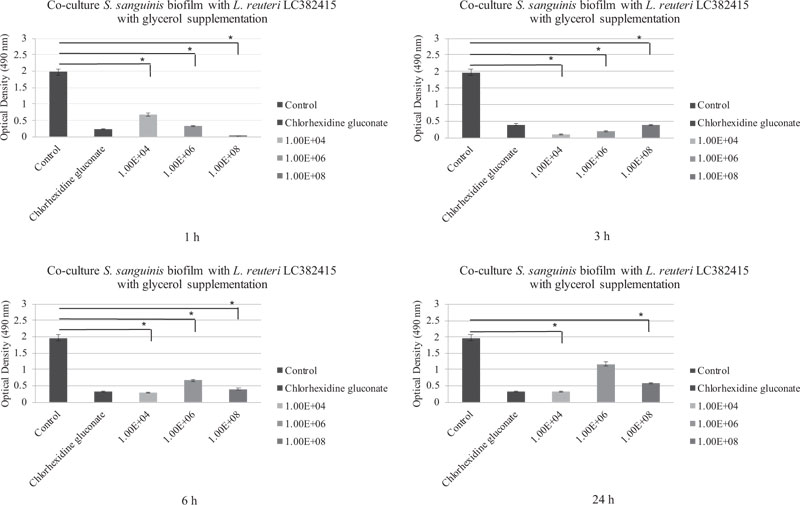
Comparisons of the effects of L. reuteri LC382415 with and without glycerol supplementation are shown in Fig. (6-9). Lactobacillus reuteri LC382415 in a concentration of 1 × 108 CFU/mL resulted in a significantly greater mono-species S. mutans biofilm reduction with than without glycerol supplementation after incubation for 1-6 h (Fig. 7). An L. reuteri LC382415 concentration of 1 × 108 CFU/mL also caused a greater mono-species S. sanguinis biofilm reduction with than without glycerol supplementation after incubation for 1 h (Fig. 8). Moreover, almost all concentrations of L. reuteri LC382415 resulted in a greater dual-species formation reduction with than without glycerol supplementation after incubation for 1-6 h (Fig. 9).
The dual-species biofilm was significantly reduced after treatment with all concentrations of L. reuteri LC382415 with glycerol supplementation after incubation for 3 and 6 h compared to the negative control (p < 0.05). Fig. (6) shows the co-cultured L. reuteri LC382415 and dual-species biofilm mass OD after incubation for 1, 3, 6, and 24 h with glycerol supplementation.
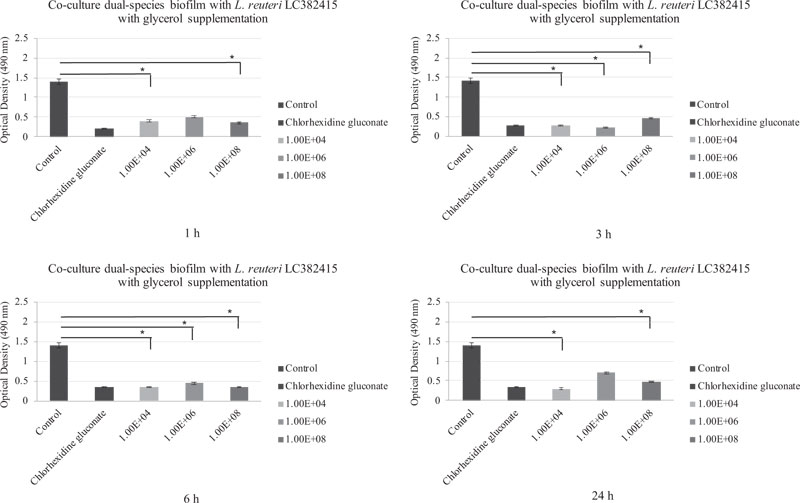
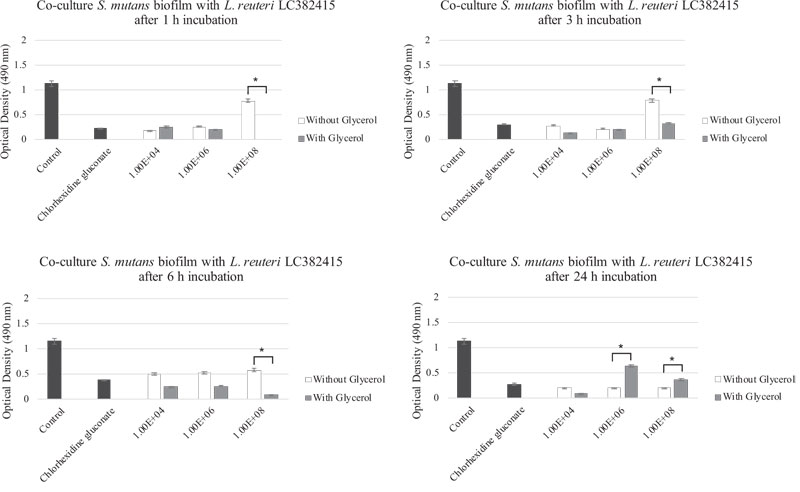
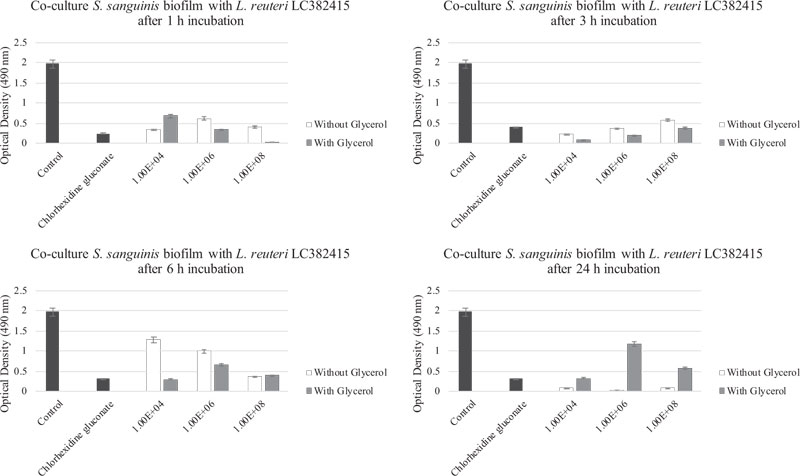
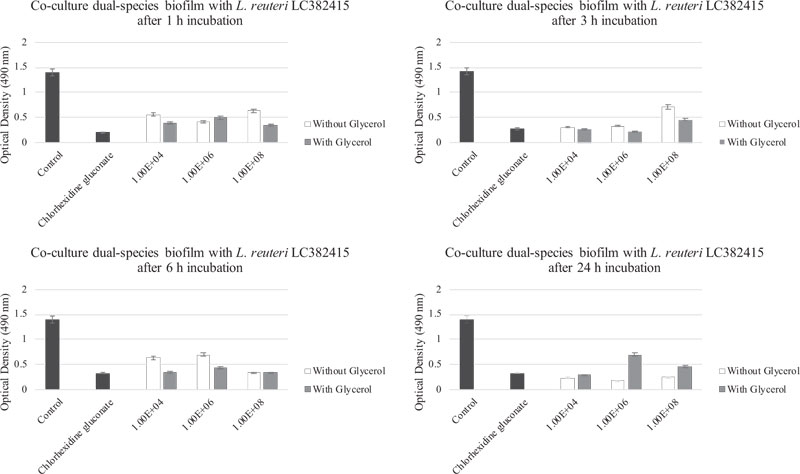
4. DISCUSSION
Dental caries is a multifactorial phenomenon representing a major problem of the oral cavity that affects a significant number of people worldwide. It may be caused by oral biofilm formation, which can lead to the destruction of the tooth [22].
The oral biofilm is formed by multi-species communities colonizing the oral cavity in stages (initial, early, middle, and late) [23]. The initial colonization process is dominated by oral streptococci, such as S. mutans and S. sanguinis, which account for over 80% of the early biofilm [11]. Lactobacillus species have known to be linked with dental caries for years, as they have been considered secondary invaders [24]. However, Lactobacillus species have also been reported to be beneficial for the oral cavity [25]. In this study, the Indonesian strain of L. reuteri LC382415 was found to decrease mono- and dual-species S. mutans and S. sanguinis biofilms. Although the exact mechanism has yet to be elucidated, it can be speculated that it is related to competition for nutrients and the ability of L. reuteri LC382415 to inhibit the adherence of competing species by producing antibacterial peptides.
Our results show that L. reuteri LC382415 in a concentration of 1 × 104 CFU/mL without glycerol supplementation effectively reduced the mono-species S. mutans biofilm after all incubation periods, even after 24 h. The mono-species S. sanguinis biofilm was also effectively reduced after incubation for 24 h. Lactobacillus reuteri may have the ability to produce hydrogen peroxide (H2O2), which can be toxic to organisms lacking hydrogen peroxide-scavenging enzymes, such as S. mutans, thus inhibiting their biofilm growth [12]. Moreover, H2O2 produced by L. reuteri or other streptococci can induce autolysis in S. sanguinis [26].
All concentrations of L. reuteri LC382415 with glycerol supplementation significantly reduced the mono-species S. mutans and S. sanguinis biofilms after incubation for 1–6 h. Similar effects were observed in the dual-species biofilm co-cultures. This might be due to the ability of L. reuteri to produce reuterin (3-HPA) by metabolizing glycerol. Reuterin is used as a broad-spectrum antimicrobial agent and has more advantages than other antimicrobial agents [27].
Different incubation periods were used in this study to determine the optimum time required for L. reuteri to inhibit biofilm formation. After 1 h of incubation, biofilm formation enters the adhesion stage, which is reversible, and the attached bacteria are easily removed from the pellicle [28]. After 24 h of incubation, the co-cultured L. reuteri LC382415 and mono-and dual-species biofilms with glycerol supplementation tended to increase, although still at a lower rate compared to the negative control. This suggests that the pathogenic bacteria gradually increased their antibacterial resistance and multiplied as the biofilm entered the maturation stage. It is harder for antimicrobial agents to penetrate thicker biofilms [29], as their glycocalyces protect the bacteria and enhance their immunity to antibiotic agents [30].
The L. reuteri LC382415 strain was isolated from young Indonesian adults. The sequence results and Basic Local Alignment Search Tool (BLAST) confirmed that the DNA sequence of this strain was similar to L. reuteri ATCC 55730 [31]. The evolution gap between two bacterial strains can be measured using their nucleotide gene identity through BLAST, with average nucleotide identity (ANI) of 95-98% [32]. Lactobacillus reuteri LC 382415 similarity to L. reuteri ATCC 55730, as suggested by an ANI of 96% [21].
The probiotic biofilm inhibition mechanism lies in enhancing the epithelial barrier by inducing mucus secretion, along with enhancing probiotic and inhibiting pathogenic microorganism adhesion, competing with pathogenic microorganisms for nutrients on the host cell surface, and producing other antimicrobial agents, such as lactic acid, acetate acid, and bacteriocin, thereby reinforcing the immune system [33]. Lactic acid and acetate acid produced by L. reuteri contribute to pathogenic bacterial growth inhibition due to their ability to penetrate the bacterial cell membrane, dissociate with the alkaline environment inside the cell, and lower the cytoplasm pH [34]. Bacteriocin and hydrogen peroxide can destroy pathogenic bacterial cell membranes and inhibit pathogenic DNA synthesis. Therefore, probiotics can prevent pathogenic microorganism adhesion and colonization [35]. Wasfi et al. (2018) also found that Lactobacillus spp. probiotics, including L. reuteri ATCC 23272, can prevent dental caries activity. Their anti-caries effect is related to the inhibition of S. mutans biofilm growth, especially because of the secretion of organic acid production of H2O2 [12].
The effectiveness of a probiotic depends on certain factors, such as its ability to survive in enzyme-rich and low-pH environments, its interaction with the host, its ability to compete with pathogenic microorganisms, and most importantly, its safety [36]. L. reuteri probiotics have been proven to meet all these conditions [37]. In recent years, the microbial activity of Lactobacillus has been found to depend on the specific strain and the pH environment [38]. Lactobacillus has been reported to reduce Streptococcus adhesion [39]. These explain how the presence of L. reuteri LC382415 significantly reduced the dual-species biofilm of S. mutans and S. sanguinis.
CONCLUSION
This study demonstrates the promising activity of L. reuteri LC382415 with glycerol supplementation against initial-stage biofilms. Future studies on the mechanism of action of this active component are warranted.
ETHICS APPROVAL AND CONSENT TO PARTICIPATE
Not applicable.
HUMAN AND ANIMAL RIGHTS
No animals/humans were used for studies that are the basis of this research.
CONSENT FOR PUBLICATION
Not applicable.
AVAILABILITY OF DATA AND MATERIALS
The data sets analysed during the current study are available from the corresponding author, [C.F.T] upon request.
FUNDING
This research was supported by Faculty of Dentistry, Trisakti University, Jakarta, Indonesia.
CONFLICT OF INTEREST
The authors declare no conflict of interest, financial or otherwise.
ACKNOWLEDGEMENTS
The authors wish to thank Mario Richi, S.Si and Aradhea Monica Dreastia, S.Si for their laboratory assistance.