All published articles of this journal are available on ScienceDirect.
The Affinity of Human Fetal Osteoblast to Laser-Modified Titanium Implant Fixtures
Abstract
Introduction:
Implant surface modification methods have recently involved laser treatment to achieve the desired implant surface characteristics. Meanwhile, surface modification could potentially introduce foreign elements to the implant surface during the manufacturing process.
Objectives:
The study aimed to investigate the surface chemistry and topography of commercially available laser-modified titanium implants, together with evaluating the cell morphology and cell adhesion of human fetal osteoblast (hFOB) seeded onto the same implants.
Method:
Six (6) samples of commercially available laser-modified titanium implants were investigated. These implants were manufactured by two different companies. Three (3) implants were made from commercially pure grade 4 Titanium (Brand X); and three were made from grade 5 Ti6Al4V (Brand Y). The surface topography of these implants was analyzed by scanning electron microscope (SEM) and the surface chemistry was evaluated with electron dispersive x-ray spectroscopy(EDS). Human fetal osteoblasts were seeded onto the implant fixtures to investigate the biocompatibility and adhesion.
Results & Discussion:
Brand X displayed dark areas under SEM while it was rarely found on brand Y. These dark areas were consistent with their organic matter. The hFOB cell experiments revealed cell adhesion with filopodia on Brand X samples which is consistent with cell maturation. The cells on Brand Y were morphologically round and lacked projections, one sample was devoid of any noticeable cells under SEM. Cell adhesion was observed early at 48 hrs in laser-irradiated titanium fixtures from both the brands.
Conclusion:
The presence of organic impurities in Brand X should not be overlooked because disruption of the osseointegration process may occur due to the rejection of the biomaterial in an in-vivo model. Nevertheless, there was insufficient evidence to link implant failure directly with carbon contaminated implant surfaces. Further studies to determine the toxicity of Vanadium from Ti6Al4V in an in-vivo environment should indicate the reason for different cell maturation.
1. INTRODUCTION
The success of dental implants hinges on an integral phenomenon where direct contact between bone and an implanted biomaterial occurs, this event is termed as osseointe gration. The current consensus supports the use of titanium implants with rough surfaces for more predictable peri-implant bone adhesion [1]. With this knowledge, dental implant manufacturers either use subtractive and/or additive methods to optimize the surface characteristics of titanium implants. Subtractive methods include machining, anodization, Sandblasted Large grit acid etching (SLA) and laser irradiation. Additive methods involve coating the surface of the implant with materials such as titanium plasma, hydroxyapatite, calcium phosphate or bone morphogenic protein. Apart from laser irradiation, all of the above-mentioned surface modification methods could potentially introduce foreign elements to the implant surface during the manufacturing process. Laser irradiation is a clean and economical option because the lack of direct contact with the titanium fixture during the surface modifying process could ensure a contaminant-free product [2].
Multiple animal studies have documented the superiority of laser modified (LM) titanium surfaces over other surface modifying techniques especially in terms of the removal torque post-implant insertion [3-5]. Therefore, there is a need for more information regarding the cellular events when cells come in contact with LM surfaces. LM titanium discs have displayed mature osteoblasts with filopodia in in-vitro experiments [6, 7]. However, these experiments employed osteosarcoma derived cell lines, which tend to exhibit abnormal cell growth patterns that are less representative of completely differentiated osteoblasts [8]. In contrast, primary cell lines like Human Fetal Osteoblast (hFOB) were reported to have minimal chromosome abnormalities alongside matrix producing properties similar to differentiated osteoblasts [8]. These properties make hFOB the ideal model for osteoblast biology experiments [8, 9].
Although the current literature related to laser-modified titanium implants exhibits rather promising results in terms of the surface purity of LM implants [2, 10], the actual product the clinician purchases could be tainted or contaminated during packaging. Duddeck et al., observed contamination in many commercially available implants. They found both organic (carbon, polyethylene) and inorganic (aluminum, nickel, steel, chromium) impurities via Scanning Electron Microscope (SEM) and Electron Dispersive X-ray Spectroscopy (EDS) analysis of supposedly sterile-packaged implants [11]. This alarming report reinforces the requirement for more scrutiny of supposedly pristine implants, even in LM Titanium implants.
Due to the lack of information regarding commercially available LM titanium implants and the reaction of hFOB to LM titanium surfaces, the aims of this study were to:
1. Analyze the surface chemistry and morphology of commercially available laser- modified titanium implants.
2. Visualize the cell morphology of hFOB seeded onto commercially available laser- modified titanium implants.
2. MATERIALS AND METHODS
2.1. SEM and EDS of Implants
Six (6) implants were purchased, 3 from biomate (Brand X) and 3 from biohorizon (Brand Y). It is important to note here that Brand X implants were manufactured from commercially pure grade 4 titanium, whereas brand Y implants were manufactured from grade 5 titanium Alloy (Ti6Al4V).
All the samples were in sterilized packages and opened only during sample analysis. To examine the surface morphology and chemistry of the implants, a scanning electron microscope with EDS (JSM 5410LV, JEOL, Tokyo, Japan) was used. Three (3) samples from each brand were analyzed under SEM and EDS. The visualization of the surface was performed at three sections, coronal, middle and apical sections in each one of the implants. The samples were placed on carbon discs for fixation and then inside the microscope chamber under vacuum conditions. EDS was used for qualitative and quantitative elemental analysis, and random spots were selected and analyzed.
2.2. Cell Culture
Human fetal osteoblast cell line hFOB 1.19 (ATCC® CRL11372TM), American Type Culture Collection (Manassas, VA, USA), following the guidelines mentioned in the study by Harris et al. [12] was cultured in a 1:1 mixture of Ham’s F12 Medium and Dulbecco’s Modified Eagle’s Medium with 2.5 mM L-glutamine (without phenol red) (Gibco, Life Technologies, Paisley, UK) supplemented with 10% (v/v) fetal bovine serum (FBS) and 0.3 mg/mL geneticin (G418, Serva Electrophoresis GmbH, Heidelberg, Germany). hFOB were maintained in proliferative stage at 34 ◦C under 5% CO2 in a humidified incubator. Culture media were refreshed every other day.
2.3. Cell Adhesion Experiments
All implants were cleaned and degreased with ethanol at different concentrations and sterilized in an autoclave at 134°C for 15 min. Each implant was then placed horizontally in a 6-well culture plate. The cells were seeded at a density of 100,000 cells per implant using a micropipette. After 48 hrs, the implants were removed from incubation and fixed with cold 4% glutaraldehyde in Dulbecco’s phosphate-buffered saline (DPBS) for 2 days. Then, implants were dehydrated in different concentrations of ethanol and were coated with gold for SEM analysis.
3. RESULTS
Two (2) samples from Brand X displayed dark areas around the collar and upper threads (Fig. 1) under SEM, which appeared to be indicative of organic elements. Carbon appears darker than titanium in the material viewed by the contrast image because carbon atoms have fewer electrons.
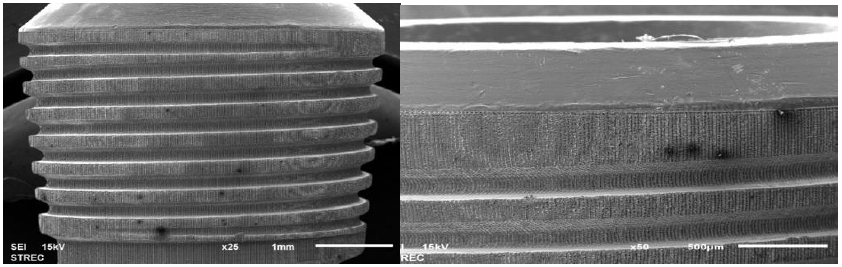
At x5000, the typical morphology of lasered surfaces is clear; the spherical structures with microcracks were the result of rapid melting (Fig. 2) and cooling of the metal immediately after laser. EDS results displayed oxygen, titanium and small amounts of carbon in all 3 samples (Fig. 3). The presence of carbon is consistent with the dark areas.
All brand Y samples were relatively clean and displayed no distinct dark areas under SEM (Fig. 4). This could be indicative of a cleaner packaging process post-laser-irradiation. At x2000, symmetrical rods and spheres could be visualized (Fig. 5). EDS results showed oxygen, titanium, aluminum and vanadium with a small amount of carbon in all 3 samples of brand Y (Fig. 6). The EDS results clearly showed the presence of vanadium ions which have been postulated to be non-biocompatible. The chemical composition (atomic %) of 2 brand samples is demonstrated in Table 1 (Mean ± SD).
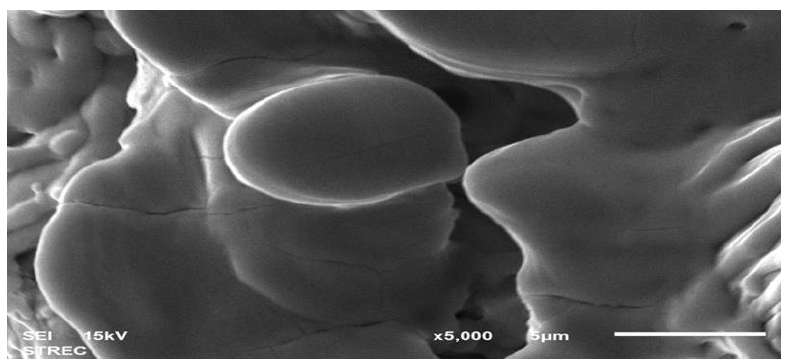
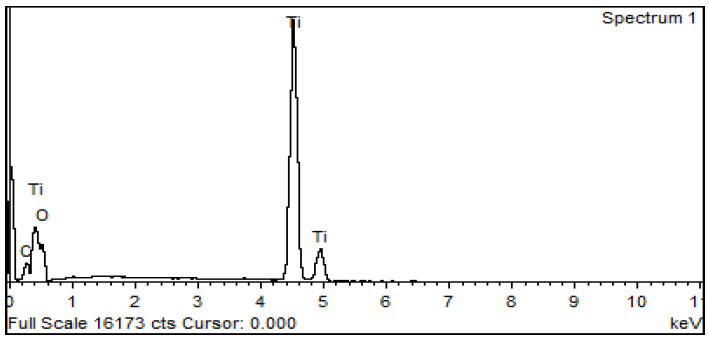
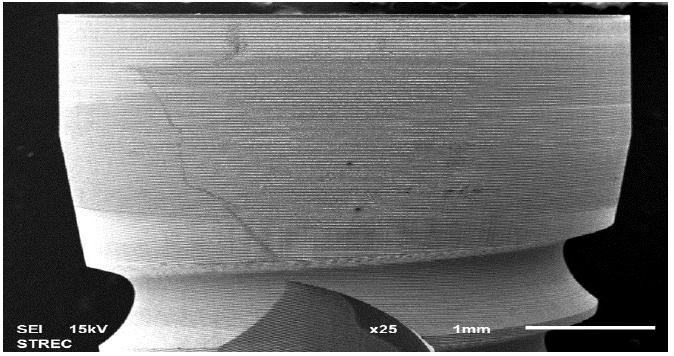
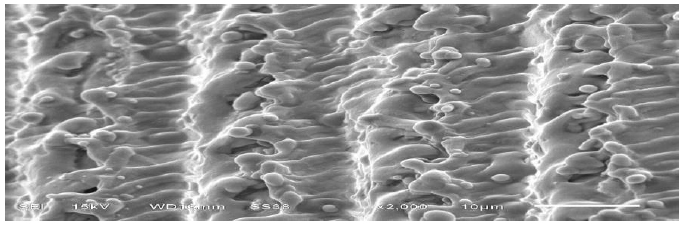
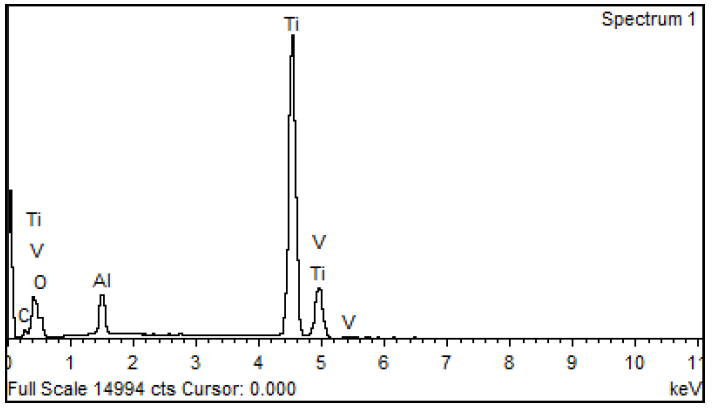
Elements | Brand X | Brand Y |
---|---|---|
Carbon | 8.32 ± 2.92 | 3.94 ± 4.43 |
Oxygen | 42.59 ± 9.37 | 20.71 ± 19.30 |
Titanium | 48.94 ± 8.92 | 66.325 ± 14.94 |
Aluminum | 0 | 6.03 ± 1.35 |
Vanadium | 0 | 2.99 ± 0.79 |
4. CELL ADHESION EXPERIMENTS
This experiment was a descriptive analysis with the help of images. After 48 hrs, all 6 samples were visualized under SEM; a startling finding was the lack of mature cells on brand Y surfaces. One sample of brand Y displayed zero cell adherence, while the other 2 brand Y samples displayed cells that were rounded and had short processes (Figs. 7a and 7b). The short processes of osteoblast cells displayed lack of maturation at 48 hrs. Although brand Y samples were free of contaminants, the overall lack of mature cell adhesion after 48hrs could indicate the need for a longer duration for cells to mature on titanium alloys. The presence of impurities in the form of organic debris on brand X surfaces showed no impact on cell adhesion experiments. Brand X implant samples displayed good cell maturation with filopodia (Fig. 8). The filopodia show a single osteoblast cell maturing and attachment of its arms to further sites on the implants and cell attachment with filopodia in different directions, even in the presence of darker areas, which are probably organic debris (Fig. 9).
5. DISCUSSION
Osseointegration is regarded as an essential indicator of success in oral rehabilitation with dental implants. Osseointegration is a dynamic process that involves multivariate factors that determine the direct union of bone with a biomaterial without concomitant unfavorable events such as marginal bone loss or peri-implantitis. Therefore, titanium should not be considered inert when used as an implant material, instead in an ideal scenario, after insertion into bone, it triggers a foreign body reaction which causes mild chronic inflammation, that is resolved with time by the formation of a dense bone, engulfing the implant (osseointegration) [13]. With this in mind, we can hypothesize that the introduction of additional foreign elements, whether organic or inorganic could disrupt the foreign body equilibrium, thereby, inducing more osteoclastic activity with minimal osteoblasts during bone remodeling.
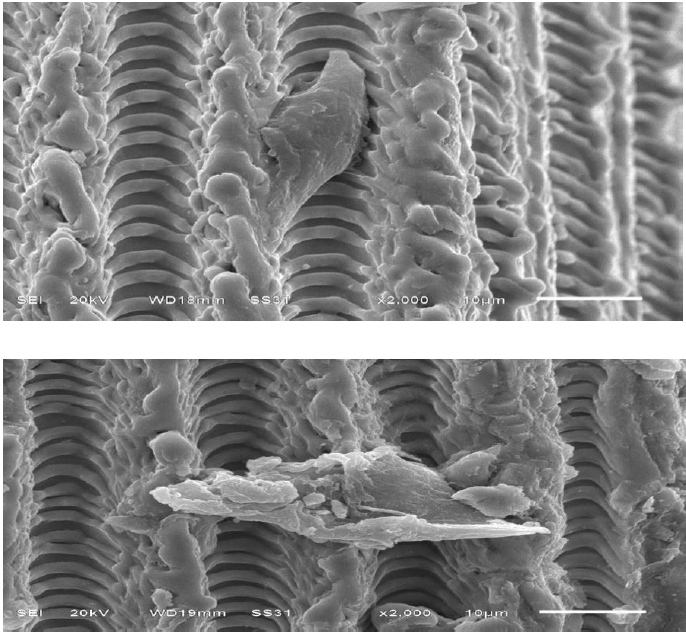
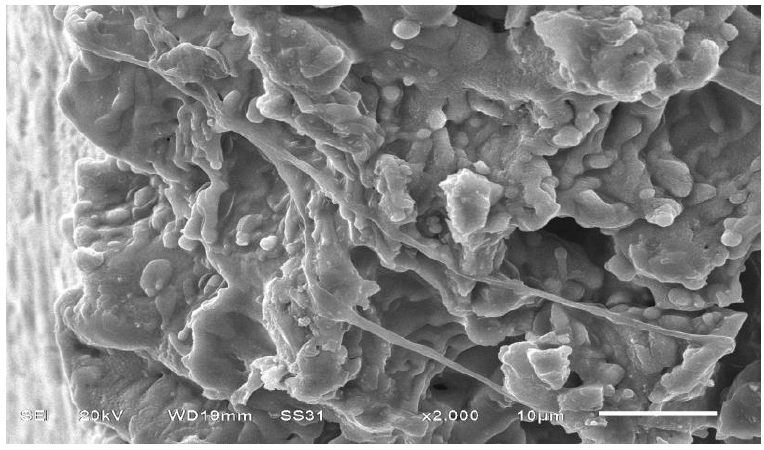
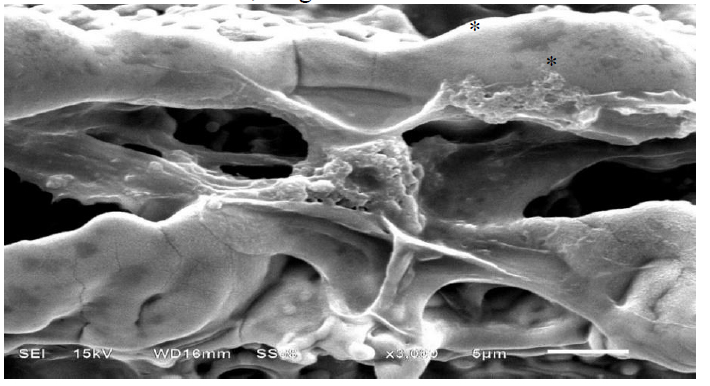
The impurities on the surface of titanium implants could occur due to residual elements generated by the surface alteration process [10] or organic contamination from the packaging of dental implants [11]. These contaminants could disrupt the healing of the implant to bone interface, thus delaying osseointegration or causing early marginal bone loss. Therefore, quality control during the manufacturing and packaging of commercially available dental implants should be monitored carefully. Brand X samples in our study displayed dark areas under SEM which is indicative of organic impurities. A likely source of the organic debris found on brand X implants could be from the contact of the implant surface with plastic elements during the packaging process. Although the organic matter on the implant surface did not hinder cell adhesion in this study, the in-vitro state of the cells here does not represent the actual process of healing following implantation of a biomaterial. The in-vitro environment discounts the presence of inflammatory and immunomodulatory events.
The organic debris on the surface of the brand X implants is not an uncommon finding. Carbon is the most widely found contaminant on commercial implants. Morra et al., reported carbon contamination in commercially available titanium implants regardless of the surface alteration process used by the manufacturers [14]. Although the exact minimum dose of carbon to elicit an unfavorable response is still nebulous, Hayashi R et al., reported concentration-dependent phenomena where a higher amount of carbon caused more unfavorable osteoblast attachment and differentiation on titanium discs [15]. It is evident in our research that the concentration of carbon in the brand X implants was not enough to deter the attachment and differentiation of hFOB to the laser-modified titanium surface.
In this experiment, the 48 hrs incubation period post-seeding of hFOB onto the implants produced good adhesion and cell maturation on brand X implant samples. A longer incubation period could have produced better cell adhesion or cell maturation on brand Y samples. Longer hFOB incubation periods (7days and 15days) with lasered zirconia implants were reported to produce cells with filopodia after 7days, while intercellular contacts, which converged into a single layer that adhered onto the surface of the implants, were observed after 15 days [16].
The vast majority of dental implants on the market are made from either titanium alloys (Ti6Al4V) or commercially pure titanium(Cp-Ti). Despite having different strength and elemental composition, Shah F et al., concluded that Ti6Al4V and Cp-Ti had no significant difference in their ability as an implant material to achieve osseointegration [17]. However, Steinemann documented the possibility of foreign body reactions characterized by an increase in inflammatory cells in tissues surrounding implanted Ti6Al4V as opposed to Cp-Ti implants [18]. He further attributed these findings to the leaching of vanadium ions from the Ti6Al4V implants [18]. Okazaki et al., discovered small amounts of vanadium in the tissues around Ti6Al4V implants that were inserted in rat tibia [19]. Because vanadium itself is toxic, the presence of vanadium particles even in trace amounts should be a matter of concern. In this study, brand Y implants were made from Ti6Al4V, while brand X implants were made from Cp-Ti grade 4. The lack of mature cells after 48 hours of hFOB cell culture in brand Y samples could be ascribed to the presence of vanadium ions which are highly toxic.
Another potential reason for the lack of mature attachment could be simply due to the perceived shallower depth between each groove on the brand Y implants. This highlights a limitation of this research because a quantitative value of the roughness of each implant was not assessed. The use of a confocal microscope or digital profilometer could give a quantitative value of the micro-roughness average of each implant.
CONCLUSION
Laser-modified titanium implants have the potential for increasing the chances of successful osseointegration. The brand X implant samples that displayed human fetal osteoblast with filopodia infer the possibility of successful osseointegration. Although, the results in our study showed that cell adhesion is possible after 48 hrs in laser-modified titanium, there are some limitations such as the low sample size and lack of statistical analysis to make a definitive conclusion. Additionally, the main reason for the lack of cell maturity and cell adhesion in brand Y samples remains unanswerable, further studies to determine the toxicity of vanadium from Ti6Al4V in an in-vivo environment and titanium alloys lasered to a similar roughness of brand X should indicate the reason for the disparity between the two implant brands. The presence of organic impurities in brand X samples should not be overlooked despite good cell adhesion results. Impurities on the surface of titanium implants could potentially be hazardous in an in-vivo model resulting in the disruption of the osseointegration process by the rejection of the biomaterial. Nonetheless, there is currently insufficient evidence to link implant failure directly to carbon contaminated implant surfaces.
ETHICS APPROVAL AND CONSENT TO PARTI-CIPATE
Not applicable.
HUMAN AND ANIMAL RIGHTS
No animals/humans were used for studies that are the basis of this research.
CONSENT FOR PUBLICATION
Not applicable.
AVAILABILITY OF DATA AND MATERIALS
The data that support the findings of this study are available from the corresponding author, [DS], upon reasonable request.
FUNDING
The research project was financially supported by Mahidol University and Master of Science program in Oral and Maxillofacial Surgery, Faculty of Dentistry, Mahidol University.
CONFLICT OF INTEREST
The authors declare no conflict of interest, financial or otherwise.
ACKNOWLEDGEMENTS
The authors would like to thank all the staff in the Department of Oral and Maxillofacial Surgery, Department of Oral Biology and Research Center of Faculty of Dentistry, Mahidol University.