All published articles of this journal are available on ScienceDirect.
Tooth Mobility Reproduction in Dental Material Research: A Scoping Review
Abstract
Background:
Introducing tooth mobility simulation in laboratory studies can provide results with high accuracy and predictability.
Objectives:
This study aims to review in vitro methodologies replicating tooth mobility and provide a recommended approach for future laboratory models.
Methods:
Databases, such as PubMed, Cochrane Database of Systematic Review, BioMed Central and Chinese databases are searched, and twelve articles are included in the final review.
Results:
Simulation methods of tooth mobility involving socket enlargement, screw loosening, alveolar bone loss simulation and a combination approach are identified from the extracted data. The materials used in preparing artificial teeth, artificial sockets and periodontal ligament simulator are discussed with a focus on their limitations. The achieved degrees of mobility and the presence of the centre of rotation are also evaluated. A timeline of the review articles is constructed to understand the trend of the preferred methods in tooth mobility simulation.
Conclusion:
Future in vitro investigations can achieve clinical reliability, particularly for materials tested in the field of dental traumatology and periodontology, by recognising the importance of incorporating tooth mobility in laboratory studies. Improvised methods are proposed to ensure that potential laboratory models can resemble the actual oral environment.
1. INTRODUCTION
Modern dental materials still underperform in clinical setting despite the promising results from laboratory studies. Clinicians often attributed these failures to the incomplete methodologies and the limitations of in vitro studies. Therefore, clinical trials are generally preferred due to their reliability research outcome in advancing biomaterial science [1]. However, their results are highly dependent on patient compliance and require years of follow-up examinations [2]. Besides, testing dental materials in clinical scenarios, such as periodontal disease or trauma, can be challenging and deemed unethical. Accordingly, in vitro studies are commonly conducted in this dental fraternity due to its reproducibility.
For decades, researchers have simulated the oral environment by using laboratory models that resemble the natural human mouth condition. A commonly used protocol is the placement of samples in a wet environment via a water bath at 32 °C [3] or artificial saliva [4]. Artificial ageing is also simulated using a thermocycling machine for a fixed number of cycles over a fixed transfer time [5]. Chewing simulator machines were later developed to enable mechanical loading on samples at different angles and imitate the chewing cycle during eating [2]. However, these methods rely on an external source with minimal focus on the sample itself. The behaviour of a tested stationary tooth might represent that of an implant rather than an intact tooth [6]. Overestimated results were reported because the resiliency of tested specimens is ignored [7]. Hence, instilling the presence of ‘periodontal ligament’ surrounding the tested tooth is recommended in various laboratory studies [7-12].
Periodontal ligament (PDL) is a piece of connective tissue that provides tooth support, encircles the root of the tooth and connects the teeth to the alveolar bone [13]. Its mechanism can be explained based on the simple spring (elastic) and dashpot (viscous) concept [14-17]. The constant roles of the two elements account for the ‘springy’ characteristic of the PDL when the load is briefly applied and removed. The following essential concepts can be inferred: (1) the viscoelastic properties of PDL during loading and their return to the original position and (2) tooth displacement during loading under the effect of the Modulus of Elasticity (MOE) of the PDL. Both points must be considered in laboratory simulations to accurately obtain the mechanical characteristics of PDL. Many PDL behaviours are replicated with several elastomeric dental materials due to their elastic properties. The roots of the teeth were coated with elastomers or rubber die spacer before being embedded into the artificial bone-like material during sample preparation [11, 12].
Increased tooth mobility can be reproduced by increasing the deflection of the simulated tooth within the artificial socket. The Glossary of Periodontics defines tooth mobility as ‘the movement of a tooth within its socket due to an applied force’. When a tooth moves within a healthy socket, a centre of rotation (CRO) commonly exists apical to the centroid of the tooth [18, 19]. When the bone level decreases, CRO moves apically, and the distance from CRO to the crown increases, resulting in an increased deflection degree, which is equivalent to the increase in tooth mobility.
To the best of our knowledge, advanced methodologies on increasing tooth mobility mimicking traumatic or periodontally compromised scenarios are limited and vague. Hence, this study primarily aimed to review laboratory methodologies that simulate increased mobility in relation to CRO. Additionally, this work provides suggestions on improving the established methods and developing a simplified laboratory model in the future.
2. MATERIALS AND METHODS
A scoping review was conducted to determine laboratory investigations presented with methodologies describing the simulation of tooth mobility in their sample preparations or newly invented models on the reproduction of tooth mobility. A scoping review allowed a substantial search on the body of literature on an interesting topic with focus on evidence that may still be unclear to others [20]. Having a broader scope of review, the available data on in vitro studies simulating tooth mobility can be mapped and key characteristic or biomaterial features of these investigations can be identified [21]. Following the preferred reporting items for systematic reviews extension for scoping reviews (PRISMA-ScR) [22], the question used as a guide for literature search was, “What are the methods used in laboratory studies to simulate an increase in tooth mobility?”.
A review of published literature from year 1990 until July 2020 was performed using PubMed, Cochrane Database of Systematic Review and BioMed Central databases with the following search terms: ((‘Tooth Mobility’ OR ‘Tooth Mobilities’ OR ‘Periodontal Diseases’ OR ‘Periodontal Ligament’ OR ‘Mobilities’ OR ‘Dental Trauma’ OR ‘Dental Splint’) AND (Simulation OR Reproduction OR Recover OR Replica OR Imitation) AND (Experimental OR Laboratory OR ‘In vitro’)). Chinese databases, such as China National Knowledge Infrastructure, Wanfang database and VIP information, were also explored using Chinese characters representing ‘tooth mobility’ and ‘simulation’. Articles related to the topic were also manually searched to retrieve other related studies. The search strategy was adapted and modified on the basis of the literature search in other databases with no limitations in regards to language.
Articles with irrelevant titles and abstracts to the laboratory or in vitro investigations and tooth mobility simulation were excluded. Finite element studies were also excluded due to the considerable digital formulation unrelated to the topic. Any animal or cell study on periodontal ligament was also excluded due to the differences in laboratory infrastructure. Only studies concentrating on artificial models or artificial teeth were included.
By using a predefined data extraction table, two authors (Y.A. and M.R.) independently extracted the data from each study. Disagreements were discussed among the authors until a consensus was reached. A third review author was consulted as needed. The extracted data consisting of author name, publication year, methods of tooth mobility simulation, materials used to fabricate the teeth, type of socket, materials used as the PDL simulator, type of mobility achieved and CRO application were collected and tabulated using Microsoft Excel 2017.
3. RESULTS
The search and citation retrieval process are presented in Fig. (1). A total of 125 unique citations, including four potential articles retrieved manually, were identified. After the preliminary screening of titles and abstracts, seventeen potentially eligible citations were considered in the full article review. Among these articles, five were removed from the full article review because they did not satisfy the inclusion criteria. Twelve articles were included in the final review. Literature synthesis was completed by the first author and subsequently verified by the co-author prior to abstraction into one data table (Table 1). Risk of bias and quality assessment based on evidence level was not performed because of the nature and scope of the review.
Simulation methods of tooth mobility were broadly reviewed under the following categories: (i) socket enlargement, (ii) screw loosening and (iii) simulation of alveolar bone loss. Fig. (2) summarises the articles reviewed according to the simulation methods of tooth mobility.
The earliest study on tooth mobility reproduction was conducted by SORNKUL et al. [23], who tested casted splints on multiple metal dies. Mobility was simulated by enlarging the sockets by a wax thickness of 1 mm in one group and 1 to 3 mm in another group. The wax was then replaced with regular body PVS. The degree of mobility achieved an axial intrusion of 1 mm to 3 mm without mentioning CRO in the text. A novel device using a ball-ended root system with various sizes of metal arresting plates was subsequently developed [24]. The authors applied a similar concept of socket enlargement by increasing the space allowance needed for the plastic tooth to deflect within the socket. Silicone gaskets with different bevelling dimensions were chambered around the plastic teeth to further simulate the various degrees of mobility. The geometries of different types of teeth (incisor, premolar and molar) are emphasised with CRO placed at the centre of the ball situated at the apical third of the root. This study was the only one in literature that discussed the importance of CRO in tooth mobility and explained the practicality of CRO in their design. The authors also highlighted the need to standardise the geometry and size of the root to increase the accuracy of tooth deflection. A new mobility classification was invented to cater to the horizontal movement of artificial teeth, which is comparable with the mobility curve of natural teeth.
Only three articles adopted a combination of two simulation methods in increasing tooth mobility [25-27]. Two of which involved the concept of socket enlargement in their artificial plastic models, chose to either enlarged the artificial socket using high-speed diamond bur [27] or replaced the plastic central incisor tooth with a small-sized lateral incisor [26]. In the former study, the dimension of socket enlargement via hand manipulation was not discussed in detail and hence, not reproducible. Both studies concentrated on increasing the degrees of mobilities replicating traumatic teeth but were less clinically accurate as horizontal tooth movement with an approximation to degree III mobility may differ in a severely traumatized tooth.
Another combination approach of socket enlargement and screw loosening with an innovative laboratory model was reviewed [25]. The authors customised the artificial teeth with apical adjusting screws and aluminium socket with and without an increased diameter. The degrees of mobility were specific at the PerioTest values of degrees II and III to resemble dentoalveolar injuries. Different from most works that used silicone as the PDL simulator, this study introduced rubber foam to mimic the injured periodontal fibres and early-stage hematoma. Rubber foam is superior to silicone because of its near-natural resetting properties. The accumulation of energy within the thick silicone accelerates the tooth return and reduces mobility. Another startling advancement was the incorporation of bovine teeth veneering over the stainless-steel artificial teeth to provide etchable tooth surfaces. The readily etchable enamel-veneered metal teeth show superior longevity and non-oxidation properties compared with plastic teeth. The customized aluminium jaw base is easily processed, durable and available at any time with adjustable tooth mobility. However, the developed model has limited application due to its shape and difficulty in obtaining information in a near-clinical condition. CRO was not discussed in any of the combination approach; assuming the fastening screws secured the artificial teeth within the socket, the screw could probably also act as the CRO.
Readily available artificial plastic models using screw loosening to increase tooth mobility was also described [28, 29]. One of them reported being able to achieve only 1 mm horizontal mobility at the incisal edge without any specific degree of mobility after loosening the screw for five full turns [28]. In contrast, another group of researchers did not specify the number of turns to unscrew as long as PTV Degree III was obtained [29]. PDL simulator and CRO application were not mentioned in their study.
In a natural periodontium, a mobile tooth commonly occurs with clinical attachment loss or periodontal pocket. Researchers typically simulate alveolar bone loss to reproduce periodontally compromised teeth. The height of artificial bone is manipulated to replicate the increase in tooth mobility [30-34]. Interesting, all except one [34] of the reviewed articles on alveolar bone loss simulation adopted such methods in testing dental prosthesis rather than periodontal related materials. Furthermore, the authors investigating the effect of bone loss simulation and periodontal splint on bone strain did not clarify the degrees of mobility achieved after removing 5mm of simulated bone [34]. In the same article, an effort was made to replicate the mandible and its jaw sockets via polystyrene resin after the wax pattern was obtained from an impression taken on a mandible.
Most of the articles on simulating tooth mobility using the principle of alveolar bone loss preferred collected human sound teeth [31-34]. Only in the latest study [30] that altered the height of the PDL simulator to simulate alveolar bone loss, utilized a plastic model jaw with the tooth of interest made up of epoxy resin. The amount of silicone removal was unspecified as long as Degrees II mobility was achieved. One group of authors successfully induced degrees III mobility with 50% bone loss [31] and is in accordance with another recent paper [32]. However, another report concluded that they were only able to achieve degrees II mobility with 50% bone loss [33]. A timeline of methods used in simulating tooth mobility is shown in Fig. (3) to visualise the trend of laboratory methods.
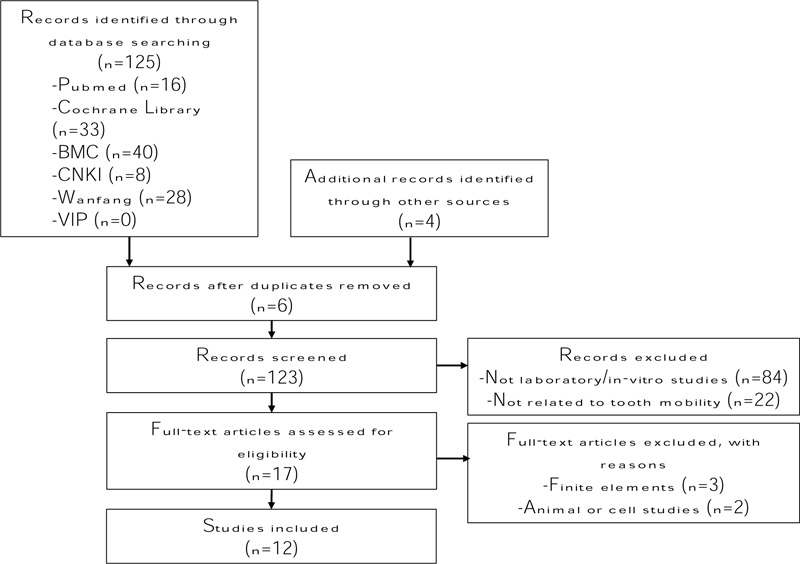
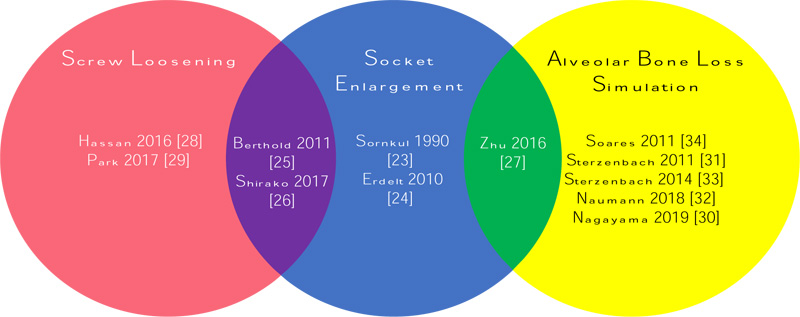
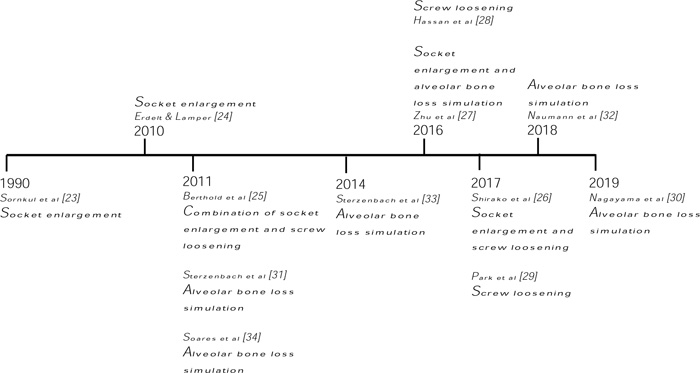
4. DISCUSSION
Elastomeric silicone is a dental material with remarkable elasticity that achieves 74% to 95% recovery within an hour [35]. Polyether is the preferred material over polysulfide [36] as the stress transferring to all the root surfaces are found to be more uniform in polyether simulated PDL. Many researchers also considered this material due to its high MOE, instantaneous elasticity and facile handling [7, 37]. Besides polyether, the new addition-type silicone provides a recovery phase of 92%, which is similar to that obtained in a human study (~90%) [6]. In this scoping review, majority of PDL simulators were composed of silicone materials, except for the articles that advocate plastic models with the screw loosening method [26, 28, 29]. In the absence of a simulated PDL, the final mobility achieved was an imitation of traumatic appearance with damaged PDL. However, without the ‘recovery’ or elastic properties of a simulated PDL, the tested material can only represent a split second of the traumatic moment, which may not be replicable in any clinical situation. Furthermore, PDL remained responsive in a traumatic tooth with early stage hematoma [10]. Hence, the PDL simulator is necessary in all laboratory models replicating an increase in tooth mobility when testing dental materials on traumatology or periodontology. An exception of PDL simulation can be taken into consideration when investigating mechanical properties of an experimented tooth with fiber post [38] or posterior full crowns behavior [39]. It was reported in their results that there were no significant differences in groups with and without PDL simulation.
In this study, it can be deduced that most researchers tend to increase the deflection or movement of the experimented tooth within the socket to increase tooth mobility. A simple technique involves enlarging the volume of the socket to increase the allowance for tooth movement. However, standardising the capacity of the newly enlarged socket in all the samples may be difficult, especially when the sockets are pre-enlarged with different wax thicknesses [23] or widened using a high-speed diamond bur [27]. The exact cutting efficiency of the plastic socket is also subjected to human error. This difficulty could explain why the specific degrees of mobility was not mentioned in previous studies.
In addition to expanding the socket size, decreasing the socket height or alveolar bone loss simulation was also discussed in multiple articles [30-34]. This technique attempts to simulate the alveolar bone loss which is usually observed in periodontally compromised dentition. Similar to socket enlargement, the reduction of artificial bone height could lead to an unpredictable and irreproducible outcome. It was observed that some of the studies achieved degree III mobility [31, 32] while another achieved degree II mobility [33]. One noticeable difference is the variation in selecting human teeth as their specimen where mandibular premolars were used in the first two studies obtained degree III mobility and maxillary central incisors gained only degree II mobility. The discrepancy in the surface area of the roots involved in the embedding procedure may be the rationale for the dissimilar mobilities as it was also reported that initial displacement of the tooth was highly dependent on the root length and diameter [40]. An increase in root diameter moved the CRO away from the apex but increasing the root length and PDL thickness brings the CRO closer to the apex.
The crown-to-root ratio (CRR) could also be an important factor in determining the final degrees of mobility; however, this value was not examined in these studies, except for one that claimed a significant difference between CRR and the different values of simulated bone loss [32]. Despite the inconsistency, human teeth are favoured by many researchers as they are readily available, inexpensive in an extensive collection and able to obtain better material adherence to the enamel surface. Standardizing the geometry of the roots to provide a uniform PDL layer can further increase the accuracy in tooth deflection [24, 41].
Custom-made artificial models designed with a computer-aided design system overcame the abovementioned problems [24, 25]. This rapid prototyping and manufacturing technologies improved the practicality of testing. However, the customized abutment teeth still lack the sufficient bonding properties for dental materials, unless sophisticated methods of instilling bovine teeth as veneers over the modified abutment teeth were performed. Such experiments may be too complex and expensive to reproduce by other researchers.
Most artificial models reviewed in this study positioned CROs at the apex of the tooth rather than at the middle third possibly because the increased distance from CRO to the crown allowed the teeth to have high degrees of deflection. This phenomenon can be observed naturally in periodontal bone loss, wherein the CRO moves apically with increasing mobility. An equation was formulated to calculate the coordinates of the rigid point of CRO under applied force and assess the characteristics of elastic properties in PDL [42]. As such, mathematical calculations may be recommended in future studies to predetermine the position of the CRO and finalise tooth mobility [19].
The findings in this scoping review provide a clear overview of the methods and evidence available in simulating an increase in tooth mobility, providing a platform for future researchers to commence further in this field of study. As the limitation of this review, it was challenging to detect the relevant investigations with the resources available and thus, more ideology of simulating tooth mobility in laboratory studies should be explored.
A combination approach may be recommended to improve future methodologies in the simulation of tooth mobility. Easily collectible natural sound teeth are the optimal choice in providing bonding surfaces for restoration and cementations of prosthesis. However, root surfaces must be standardised, and a simple composite build-up with a custom-made transparent acrylic template can easily overcome the variability in teeth selection. The space within the socket and root surface can be replaced with polysiloxane material and rubber foam to resemble the mobility of degrees I and II, respectively. The option of simulating alveolar bone loss with CRO placed apically can be considered for degrees III mobility.
Author (Year) | Methods in simulating tooth mobility | Materials used for abutment teeth | Materials used for socket fabrication | Materials used for periodontal ligament simulation | Type of mobility achieved | Center of Rotation |
---|---|---|---|---|---|---|
Sornkul et al 1990 [23] |
Socket enlargement via 1-3mm wax thickness | Metal dies (Will-Ceram Litecast B, Williams Gold) |
Autopolymerising acrylic resin block (Perm, The Hygienic Co, Akron, Ohio) | Regular body polyvinyl siloxane (Presiden Regular Body, Coltene, Hudson, Massachusetts) |
Axial intrusion of 1-3mm | Not Reported |
Erdelt and Lamper 2010 [24] |
Socket enlargement with various size of arresting plates and gasket types | High- performance polymer plastic (Everest C- Temp, KaVo, Biberach, Germany) |
Metal arresting plates and metal base | Silicone gasket (Xantropren L blau, Heraeus Kulzer GmbH, Hanau, Germany) | Self-designed mobility curves representing horizontal movements via Universal Testing Machine (0.15, 0.30, 0.60 and 1.00mm) | Centre of the ball (one-third of the length of the root) |
Sterzenbach et al 2011 [31] |
Alveolar bone loss simulation by reducing simulated acrylic socket up to 50% of root length | Human sound teeth (Lower premolars) | Acrylic resin block (Technovit 4004, Heraeus Kulzer, Germany) | Polyurethane (Anti-Rutschlack, Kaddi-Lack, Germany), polyether (ImpregumTM PentaTM, 3M ESPE, Seefeld, Germany), Polysiloxane soft cushion material (Mollosil®, DETAX, Germany) | PerioTest value (Degrees 0 to III) | Not Reported |
Berthold et al 2011 [25] |
Socket enlargement and screw loosening | Custom made V2A stainless steel teeth (REMAG AG, Nuremberg, Germany) veneered with bovine tooth | Customized jaw based with aluminum (AlCuMgPb; Metallstore, Dornburg, Germany) | Low viscosity silicone (Panasil Contact Plus; Kettenbach, Eschenburg, Germany) and rubber foam (Flexan, Waghaeusel, Germany) | PerioTest value (Degrees II to III) | Not Reported |
Soares et al 2011 [34] |
Alveolar bone loss simulation by removing 5mm of the simulated acrylic socket | Human sound teeth (Lower central incisors, lateral incisors, canines and first premolars) | Mandibular replica via polystyrene resin (Aerojet, Sao Paulo, Brazil) | Polyether (Impregum F, 3M ESPE, St. Paul, MN) | Not Reported | Not Reported |
Sterzenbach et al 2014 [33] |
Alveolar bone loss simulation by reducing simulated acrylic socket up to 25% and 50% of root length | Human sound teeth (Upper central incisors) | Acrylic resin block (Technovit 4004, Heraeus Kulzer, Germany) | Polysiloxane soft cushion material (Mollosil®, DETAX, Germany) | PerioTest value (Degrees I and II) | Not Reported |
Hassan et al 2016 [28] |
Unscrewed 5 full turns to the central incisor | Frasaco plastic teeth (Frasaco GmbH, Tettnang, Germany) | Frasaco plastic model (Frasaco GmbH, Tettnang, Germany) | None | Horizontal mobility of 1mm | Not Reported |
Zhu et al 2016 [27] |
Socket enlargement using diamond bur (TR-11; Mani Inc., Tochigi, Japan) and alveolar bone loss simulation by removing 1mm of the plastic model | Nissin plastic teeth (500H.1-M, Nissin Dental Products INC, Kyoto Japan) | Nissin plastic models (500H.1-M, Nissin Dental Products INC, Kyoto Japan) | Silicone rubber (Express Garant Light Body Regular, 3M ESPE, St. Paul, MN, USA) | Horizontal (~2.8mm) and vertical (~0.5mm) tooth movement via Universal Testing Machine as degree III mobility | Not Reported |
Shirako et al 2017 [26] |
Socket enlargement by placing a lateral incisor in the central incisor socket and removal of fastening screw | Melamine resin teeth (A5A- 500, Nissin) | Nissin plastic models (D18FE-500A-QF, Nissin Dental Products, Kyoto, Japan) | None | Moderate and severe level of trauma with PerioTest values ~31 and 50 | Not Reported |
Park et al 2017 [29] |
Screw loosening until degree III PerioTest values were achieved | Nissin plastic teeth (D85DP-500B. 1, Nissin Dental, Kyoto, Japan) | Nissin plastic models (D85DP-500B. 1, Nissin Dental, Kyoto, Japan) |
None | PerioTest value (Degrees III) | Not Reported |
Naumann et al 2018 [32] |
Alveolar bone loss simulation by reducing simulated acrylic socket up to 4mm (~25%) and 6mm (~50%) apical from the cementoena-mel junction | Human sound teeth (Lower premolars) | Acrylic resin block (Technovit 4004, Heraeus Kulzer, Germany) |
Polysiloxane soft cushion material (Mollosil®, DETAX, Germany) | PerioTest value (Degrees III) | Not Reported |
Nagayama et al 2019 [30] |
Alveolar bone loss simulation until degree II PerioTest values were achieved | Epoxy resin (Crystal Resin, Nissin Resin) covered with Co-Cr crown (Cobaltan, Shofu, Kyoto, Japan) | Nissin plastic models (E50-522 and E50- 550, Nissin Dental Products, Kyoto, Japan) | Silicone (Examixfine regular type, GC) | PerioTest value (Degrees II) | Not Reported |
CONCLUSION
Tooth mobility simulation is a suggested method of examining the physical properties of dental materials in in vitro studies which involve periodontally compromised teeth or traumatic situation. Each laboratory technique discussed in this scoping review presents its advantages and drawbacks. Three major principles are applied according to the study objectives as reviewed in the articles above: socket enlargement, screw loosening and alveolar bone loss simulation. An improvised model in replicating an increase in tooth mobility can be a feasible approach to provide repeatable and predictable results.
CONSENT FOR PUBLICATION
Not applicable.
FUNDING
This research was funded by the National University of Malaysia (UKM) Faculty of Dentistry [TAF316].
CONFLICTS OF INTEREST
The author declares no conflict of interest, financial or otherwise.
ACKNOWLEDGEMENTS
Declared none