All published articles of this journal are available on ScienceDirect.
Assessment of Peri-implant Buccal Bone Thickness Using Digital Imaging Techniques: A Systematic Review and Meta-analysis
Abstract
Objectives:
This systematic review aimed to answer the following focused question: Do the currently available imaging techniques provide accuracy in the assessment of peri-implant buccal bone thickness?
Methods:
A search strategy was conducted in eight electronic databases, followed by an additional manual search in grey literature and references of selected articles. Studies evaluating the accuracy of imaging techniques to measure peri-implant buccal bone thickness were included. Individual risk of bias was assessed by the Quality Assessment Tool for Diagnostic Accuracy Studies-2 (QUADAS-2). Meta-analysis was performed to evaluate CBCT accuracy. The overall effect size was determined by means of the Z-test. Q test was used to evaluate the homogeneity of effect sizes among studies and I2 was applied to determine the variance within studies.
Results:
After an initial screening, 83 studies were further selected for full reading and 13 of them were considered eligible for this review. In sum, the accuracy of Cone-beam Computed Tomography (CBCT), of ultrasound, and of computed tomography were assessed. There was no statistically significant difference between CBCT and the gold standard (p=0.81). The mean difference between measurements of bone thickness obtained by CBCT and the goldstandard was -0.0.3mm [95%CI -0.29;0.253mm].
Conclusion:
CBCT showed acceptable accuracy for assessing peri-implant bone. No meaningful conclusion could be drawn about other techniques.
1. INTRODUCTION
As far as the long-term outcome of dental implants is concerned, the health of soft and hard peri-implant tissues is essential to ensure a successful result [1, 2]. In this sense, one of the main factors responsible for their integrity is the bone volume surrounding dental implants [3-8]. A thin buccal bone is known to be a risk factor for the long-term stability of peri-implant tissues and may jeopardize esthetic and biological outcomes [9-11].Thus, assessment of buccal bone thickness during maintenance consultations is essential to prevent complications [1, 12].
Recently, Cone-beam Computed Tomography (CBCT) has allowed the non-invasive assessment of buccal bone thickness after implant placement [9, 13] Despite the well-validated reliability of CBCT, limitations regarding image quality have been shown as a result of artifacts associated with dental implants [14]. Hence, especially in cases in which bone is thinner than 1mm, this may not be accurately detectable in tomographic images [15]. An additional aspect to consider is the ionizing radiation-induced by CBCT [16]which prevents its use for periodical monitoring of dental implants [17].
In this regard, novel digital modalities focusing on peri-implant bone assessment have been evaluated to overcome these limitations [18-20]. Despite the promising results, the accuracy of new tridimensional techniques has not yet been validated in the literature. Previous systematic reviews have addressed the use of digital technologies for pre- and post-operative assessment of dental implants [17, 21, 22].Their accuracy in measuring peri-implant buccal bone thickness, however, has not yet been determined.
Considering that limiting factors can hamper the accuracy of CBCT, and that efforts have been made to find new techniques for measuring peri-implant bone thickness, the present study aimed to answer the following focused question: do the currently available tridimensional imaging techniques provide enough accuracy to assess peri-implant buccal bone thickness?
2. METHODS
2.1. Protocol and Registration
This systematic review was reported according to recommendations of “The Preferred Reporting Items for Systematic Reviews and Meta-Analyses”(PRISMA [23] and it is registered at PROSPERO under the reference number CRD42016044049.
2.2. Eligibility Criteria
PICO (P= patients; I= intervention; C= comparison; O= outcome) was defined as: tridimensional digital images of peri-implant bone (P), in which buccal bone thickness was measured (I) and compared with a physical or histological gold standard (C) in order to determine the accuracy (O) of the imaging device.Accuracy was determined as the measurement error between the evaluated technique and a gold standard. In turn, this was defined as direct or histological measurements of peri-implant bone thickness.
Inclusion criteria comprised studies evaluating the accuracy of tridimensional imaging techniques to assess peri-implant buccal bone thickness. No restriction on time or language was applied. The following parameters were considered as the exclusion criteria: 1) Case reports, literature reviews, letters or abstracts; 2) Assessment of further bone parameters other than buccal bone thickness; 3) Assessment of diagnostic methods not considered reliable for clinical use.
2.3. Information Sources
A main search strategy was developed according to PICO as following:
(((((“Dental Implants”[Mesh]) OR”Dental Implants, Single-Tooth”[Mesh] OR “Dental Implantation” OR “Dental Implant”)) AND (“Bone-Implant Interface”[Mesh] OR bone OR “peri-implant bone” OR “buccal bone” OR “cortical bone”OR “bone dimension” OR “bone thickness”)) AND (“Tomography, X-Ray Computed”[Mesh] OR “Tomography”[Mesh] OR “Spiral Cone-Beam Computed Tomography”[Mesh] OR “Ultrasonography”[Mesh] OR “Radiography, Dental”[Mesh] OR ultrasound OR “magnetic resonance” OR “CBCT” OR “digital volume tomography” OR “MRT” OR “Tridimensional Image”)) AND ((“dimensional measurement accuracy” OR accuracy)).
This search wasapplied in PubMed (Medline)databases and used as a reference for the additional Science Direct, Scopus, Embase and Web of Science databases. In addition, gray literature was explored in ProQuest, Scholar Google and OpenGrey sources. All searches were conducted from July until November 2019, and updated in February 2020. The authors did not contact study authors to identify additional studies. A reference manager software (EndNote Web, Thomson Reuters) was used to organize references and remove duplicates.
2.4. Study selection and Data collection process
Study selection was performed in duplicate by two independent reviewers. First, studies were screened according to titles and abstracts. Those studies that met the inclusion criteria were selected for full-text reading. Based on the inclusion and exclusion criteria defined, articles considered eligible for this review were selected. When sufficient data were provided, studies presenting a similar methodology were includedin the quantitative analysis. In case of disagreement between two reviewers, a third reviewer was consulted to achieve a decision.
Data were extracted from the included articles by the first reviewer and checked by the second reviewer.
2.5. Data Items
The following data were considered of interest to answer the focused question: study data (author, year and country), sample features (sample size and type) and assessment (imaging technique, exposure parameters, bone thickness definition, gold standard), and measurement details (points of evaluation, number of examiners and repeatability), findings and conclusion.
2.6. Risk of Bias in Individual Studies
The methodological risk of bias assessment was carried out using the “Quality Assessment Tool for Diagnostic Accuracy Studies-2” (QUADAS-2) [24]. The validated tool consisted of checking questions related to the study design regarding four domains: “patient selection”, “index test”, “reference standard”, and “flow and timing”. The risk of bias is determined by checking each question as “yes”, “no” or “unclear”. The final result categorized the risk of bias as “high”, “low” or “unclear” [24]. Review Manager 5.3 software (RevMan 5.3, The Nordic Cochrane Centre, Copenhagen, Denmark) was used to perform the quality assessment.
2.7. Summary of Measures
Continuous data (mean and standard deviation) were extracted from studies reporting the bone thickness measured by an imaging technique and by the gold standard.The difference in means was used for statistical analysis.In cases in which the study described the accuracy of different imaging devices, these were described separately.
2.8. Synthesis of Results
Meta-analysis was performed with studies comparing CBCT with the physical measurements using the same software reported above (RevMan 5.3). A random-effect model was assumed. Averaging was weighted by inverse variance and standardized mean difference was defined as effect size. The overall effect size was determined by means of the Z-test, and Q test was used to evaluate the homogeneity of effect sizes among studies. Heterogeneity within studies was evaluated by using inconsistency indexes (I2). I2 greater than 50% was considered an indicator of substantial heterogeneity between studies (p≤0.05) [25].
3. RESULTS
3.1. Study Selection
A flow chart describing the selection process is shown in Fig. (1). The initial search resulted in 1106 studies. Subsequently, 357 duplicated articles were removed and 740 studies remained. After reading titles and abstracts, 70 articles were selected for a complete reading. Furthermore, nine out of 524 articles extracted from grey literature, in addition to four papers selected from manual search, were chosen for a more detailed evaluation. Thus, out of the total of 83 studies selected for full-reading analysis, 70 were excluded and 13 studies were considered eligible for this review. The list of excluded articles and their respective reasons for exclusion are listed in Appendix 1.
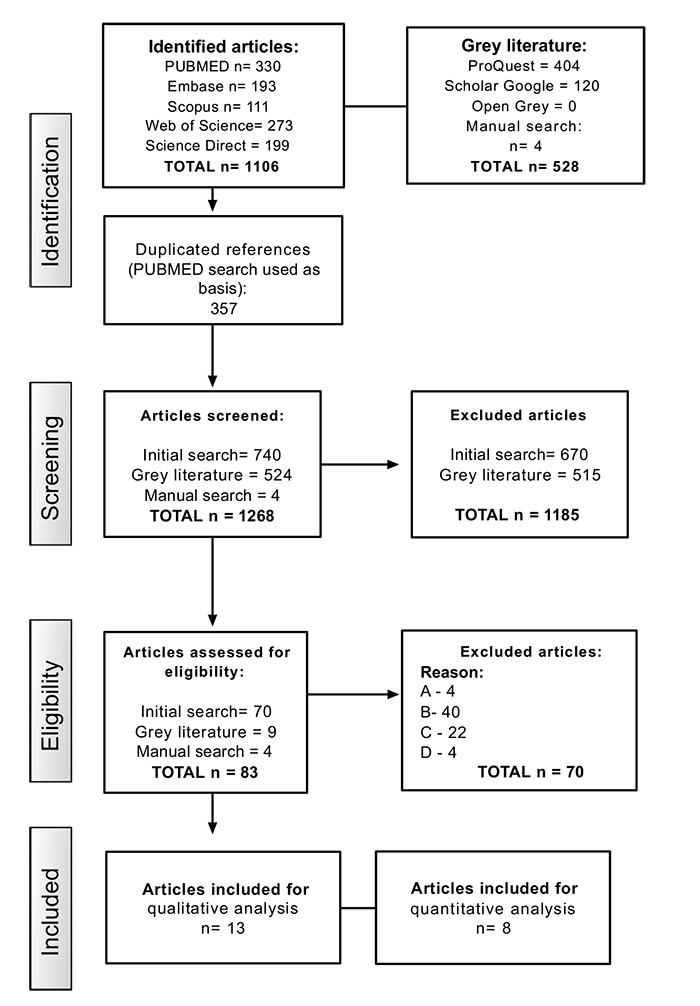
3.2. Study Characteristics
A summary of study characteristics is described in Tables 1 and 2. Accuracy of CBCT was assessed by all studies. Additionally, one study evaluated computed tomography (CT) [26] and 4 studies evaluated the use of ultrasound-based techniques (US) [27-30].
All included studies selected in this review were laboratory studies, in which a total of 219 implants were placed in cadaver jaws [28-31] dry skulls [29, 32, 33], porcine or bovine bone [27, 26, 30, 34-36] or dogs [12].
As a reference standard, one study used histological samples, [12] five studies used light microscopy [27, 29, 32-34] and 2 studies performed physical measurements using a caliper [26, 28]. Digital images, used as a pre-operative scan without implants [31, 35, 37], optical scanner [30]or digital photograph [36] were also used as a reference.
3.3. Risk of Bias within Studies
Fig. (2) shows the QUADAS-2 assessment. In general, all studies presented a low risk of bias and few concerns about applicability. The patient selection domain was considered poor, since these were laboratory studies and a randomization process was not possible. For the same reason, the QUADAS-2 question “was a case-control avoided?” was considered not applicable to these studies.
Study | Sample | - | - | Bone Assessment | ||||
---|---|---|---|---|---|---|---|---|
Author Year | Country | Bone Sample (n) | Type | Implants (n) | Implant Dimension (diameter x height) | Imaging Technique | Exposure Parameters | Gold Standard |
Bohner et al., 2017 | Brazil | 6 | Dry skulls | 18 | 3.75x9mm | CBCT (1:Carestream 9300; 2: R100 Veraview) | 1: 70kV, 6.3mA, 8.03s, 0.18mm voxel size; 2: 75kV, 5mA, 9.40s, 125µm voxel size | Direct measurements (microscope) |
Bohner et al., 2019 | Brazil | 3 | Dry skulls | 10 | 3.75x9mm | CBCT (1:Carestream 9300), US | CBCT: 70kV, 6.3mA, 8.03s, 180µm voxel size; US: 28MHz, bandwidth 84% | Direct measurements (microscope) |
Chan et al., 2018 | China | 7 | Cadavers | 17 | 3.7x13mm | CBCT (3D Accuitomo 170), US | CBCT: 120kV, 18.66mA, 20s, 80 µm; US: 25MHz | Direct measurements (caliper) |
Degen et al., 2016 | Germany | 10 | Bovine ribs | 10 | 3.8x11mm | CBCT (Galileos, Sirona), US | 85kV, 10mA, 30s; US: 42MHz, bandwidth 95% | Direct measurements (microscope) |
Gonzáles-Martín et al., 2015 | USA | 10 | Bovine ribs | 20 | - | CT (Brightspeed); CBCT (iCAT, NewTom) | CT: 120kV, 60mA, 4s 625µm; iCAT: 110kV, 4.8s, 2-3.2mA, 300µm; NewTom: 110kV, 4mA, 5,4s, 200µm | Direct measurements (caliper) |
Liedke et al., 2018 | Brazil | 40 | Dry porcine mandible | 3 | 4x9mm | CBCT (Scanora) | 1) 90 kVp, 6.3mA, 13s, 200µm; 2) 90 kVp, 6.3mA, 20s, 130µm; | Digital photograph |
Marotti et al., 2019 | Germany | 8 | Porcine ribs | 8 | 3.8x11mm | CBCT (Galileos, Sirona); US | CBCT: 85kV, 10 ma, 30S, 300µm; US: 75MHz, aperture 6.35mm, focus 2 | Optical scanner |
Rásko et al., 2016 | Hungary | 1 | Porcine mandible | 3 | 4.3x11mm; 3.8x11mm;3.8x13mm | CBCT (iCAT) | 120kV, 18mA, 8,9s, 200µm, | CBCT without implants |
Razavi et al., 2010 | United Kingdom | 10 | Bovine ribs | 10 | 4x11mm | CBCT (i-CAT NG and Accuitomo 3D, Morita) | i-CAT: 120kV, 18.54mA, 0.3mm voxel size; Accuitomo: 80kV, 4mA, 125µm voxel size | Direct measurements (microscope) |
Ritter et al., 2014 | Germany | 12 | Dogs | 26 | - | CBCT (Galileos, Sirona) | CBCT: 85kV, 28mA, 300µm voxel size | Direct measurements (Histology) |
Sheridan et al., 2018 | USA | 9 | Cadavers | 19 | 4x10mm 4x12mm |
CBCT (3D Accuitomo 170) | 120kV, 18.66mA, 20s, 400 µm; | CBCT without implants |
Shiratori et al., 2012 | Brazil | 8 | Skulls | 31 | 3.75x13mm | CBCT (i-CAT) | 120kV, 46.72mA, 40s, 250µm voxel size | Direct measurements (microscope) |
Vanderstuyft et al., 2019 | Belgium | 5 | Cadavers | 44 | 3.6x13mm | CBCT (1: NewTom, Verona; 2: Accuitomo 170, Morita) | NewTom: 110kVp, 5.2mA,150µm; Accuitomo: 90kVp, 5mA, 160µm | CBCT without implants |
Author, year | Measurements | Findings | Conclusion | ||
---|---|---|---|---|---|
Points | Examiner | Repeteability | |||
Bohner et al., 2017 | Dental implant to bone surface at apical and 5mm above | 1 | 3 | No difference between CBCT and physical measurements | CBCT is accurate |
Bohner et al., 2019 | Dental implant to bone surface at apical and 5mm above | 1 | 3 | No difference between CBCT and US to the control group | Trueness of US was similar to the one of CBCT |
Chan et al., 2018 | Outsurface of bone crest and implant surface 1mm from the bone crest | 1 | 1 | The mean absolute difference among groups ranged from 0.033 to 0.24 mm. |
Ultrasound can accurately measure bone dimensions |
Degen et al., 2016 | Distance between dental implant and bone surface | 1 | 1 | Median deviation was higher for ultrasound (US) (0.23mm) compared to CBCT measurements (0.19mm) | US showed a high potential for bone evaluation |
Gonzáles-Martín et al., 2015 | 1mm apical to the bone crest | 2 | 1 | CBCT underestimated buccal bone | Devices presented low accuracy to measure bone |
Liedke et al., 2018 | Distance between bone and implant surface from occlusal view | 3 | 1 | Low resolution jeopardize bone detection | CBCT overestimated bone thickness |
Marotti et al., 2019 | Distance between implant and bone surface along the long axis of the implant | 2 | 1 | US and CBCT showed similar measurement values to optical scanner | US presented a higher accuracy in comparison to CBCT |
Rásko et al., 2016 | Measurements were made at each thickness level | 1 | 1 | Deviation increased with a reduced bone thickness | CBCT was not accurate, especially for thin bone |
Razavi et al., 2010 | Distance between dental implant and bone surface at implant threads 3, 6 and 9mm from the top of the implant | 10 | 2 | Bone thickness calculation showed a mean deviation of 0.14±0.15mm for Accuitomo and 0.46±0.24mm for I-CAT. For bone level, the mean deviation was 0.76±0.57mm to Accuitomo and 2.10±1.58mm to I-CAT | i-CAT did not produce sufficient resolution for the thin bone |
Ritter et al., 2014 | From dental implant middle to bone surface, 4mm above apical of implant. | 2 | 2 | Mean deviation ranged from 0.06 to 2.61mm to CBCT and 0.12 to 0.43 to IR | CBCT provided usable information about bone dimension |
Sheridan et al., 2018 | At the implant platform and apex |
- | 1 | No statistical difference was found in images with and without implants | Dental implants to not hamper the measurements of bone thickness by CBCT |
Shiratori et al., 2012 | Dental implant to bone surface at apical and 5mm above | 1 | 3 | Mean difference for CBCT was 0.04±0.01mm for bone thickness and 0.13±0.86mm for bone level | CBCT is accurate |
Vanderstuyft et al., 2019 | 2,4, and 6mm to the implant shoulder | 1 | 1 | Bone thickness was underestimated by 0.3mm | CBCT underestimated bone thickness |
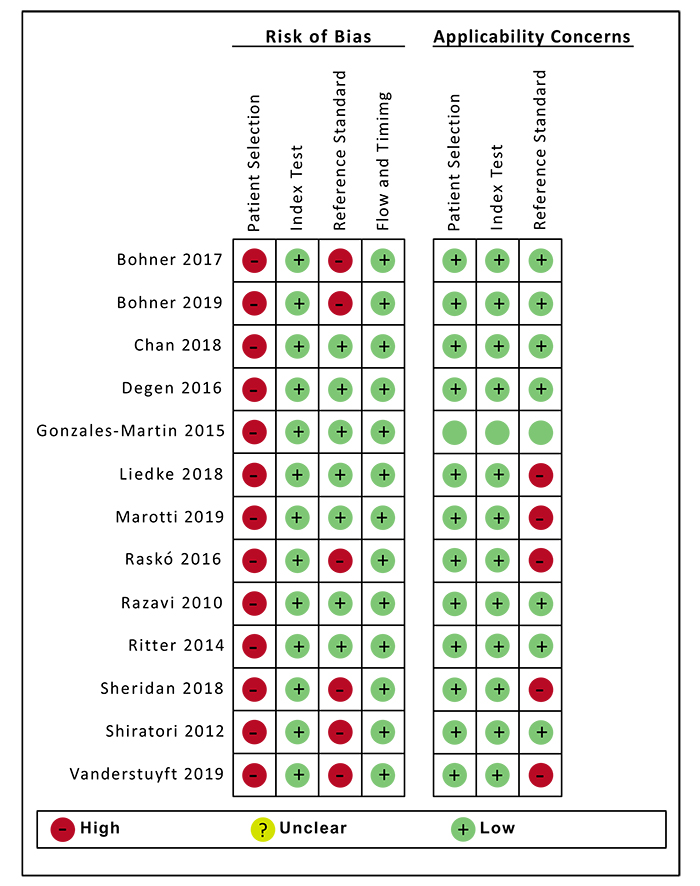
With regard to the test group, all studies presented a low risk of bias. It was unclear whether the reference standard was interpreted without knowledge of the index result. However, this was not considered a risk due to the limitations ofthe in-vitro study design.
Measurements of bone thickness, which were not performed on the physical bone samples, but cast models instead, were considered high risk. Furthermore, the studies that used digital images as gold standards were considered doubtfulforanswering the focused question of this review.
3.4. Results of Individual Studies
All studies evaluated the accuracy of CBCT with reference to standard values. In comparison with histological samples, Ritter et al. (2014) [12] showed a measurement error of 0.42mm for buccal bone thickness measured by CBCT. According to Degen et al. (2016) [27], CBCT showed a deviation of 18% when measurements were performed in the middle or center of the implant. Nonetheless, Razavi et al. (2010) [34] showed that measurement error ranged from 23% to 86% with different CBCT devices.
Shiratori et al. (2012) [32] showed an underestimation of up to 0.07mm, whereas Gonzales-Martín et al. (2015) [26]found that the measurement was underestimated in a range from 0.3mm to 0.5mm. No significant difference was found when comparing conventional with cone-beam computed tomography. The highest values were found by Bohner et al. (2017;2019) [29, 33], who showed a deviation of 0.78mm for measurements at the apex and 0.40mm for measurements 5mm abovethe apex.
When considering preoperative CBCT images, Raskó et al. (2016) [35] showed that bone thickness measurements ranged from 0.26mm to 1.65mm when CBCT images were taken without implants, and from 0mm to 1.46mm in CBCT images taken after implantation. Sheridan et al. (2018) [31] showed an underestimation of 0.04mm when measurements were taken at the implant platform and of 0.02mm when these were taken at the implant apex after placement of implants. Likewise, Vanderstuyft et al. (2019) [37] found a mean bone thickness deviation of up to 0.27mm when compared with CBCT images without implants. In comparison with optical imaging, Marotti et al. (2019) [30] showed a mean measurement error of 0.2mm for CBCT. This mean was in agreement with the study of Liedke et al. (2019) [36], , who showed that most measurement errors were under 0.5mm in comparison with measurements taken in digital photographs.
Ultrasound-based techniques were evaluated in four studies. Degen et al. (2016) [27] showed a mean error of 12.1% in comparison withthe gold standard. In comparison with optical images, the measurement error was 0.28mm. [30] Chan et al. (2018) [28] showed a mean difference of 0.3mm in comparison with direct measurements. Likewise, Bohner et al. (2019) [29] described a mean difference of 0.38mm when scanning with high-resolution ultrasound.
3.5. Synthesis of Results
I2 among studies was 11% (p=0.34). There was nostatisticallysignificant difference between the CBCT and the gold standard (p=0.81). Fig. (3) shows the mean values and standard deviation of the peri-implant buccal bone thickness measured by CBCT and by the Gold Standard.The mean difference between measurements of bone thickness between them was -0.0.3mm [95%CI -0.29;0.253mm].
4. DISCUSSION
The estimation of buccal bone thickness is an important parameter to predict the outcome of dental implant treatment. To date, the most reliable alternative to provide a non-invasive assessment of the buccal bone of the jawbone is CBCT. Nonetheless, novel imaging techniques have been investigated to overcome the limitations of a tomographic technique, such as ionizing radiation and artifacts. This research aimed to search the literature relative to the question about whether currently available techniques can provide accurate measurements of buccal bone during implant assessment.
According to the included studies, CBCT deviates from real measurements by less than 1mm, which is considered acceptable from a clinical point of view. Nonetheless, this accuracy seems to decrease as the bone becomes thinner. In this sense, Gonzales-Martín et al. (2015) [26] showed that in up to 63% of the cases, the buccal bone was not visible in CBCT imageswhen the thickness was lower than 1mm. This statement is in agreement with the findings of Shiratori et al. (2012) [32], who attributed the mean deviation range of -0.02mm to 0.07mm to the bone volume variation. Accordingly, Razavi et al. (2010) [34] claimed that an underestimation of bone thickness occurred when this was thinner than 0.8mm.
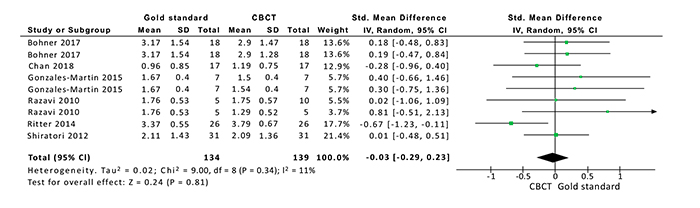
The inaccuracy of CBCT assessment is related to the appearance of metal artifacts, which may hamper the visualization of bone-implant contact [38]. Furthermore, exposure parameters and partial effect may affect the image accuracy [14]. In this sense, better accuracy is provided when the image resolution is increased by using a lower voxel size [28, 31].
With regard to ultrasound-based techniques, these provide real-time images without involving radiation, which may be considered an advantage of the technique for periodical assessment of peri-implant bone. Out of the included studies, 4 of them used a high-frequency US-transducer to scan hard tissue. Although studies reported that US can measure bone dimensions with an accuracy similar to CBCT, this is a sensitive technique, which can lead to distortions of the final image [18, 30, 39]. Thus, further studies are required to improve its use during clinical practice.
The included studies assessed only dental implants fully covered by bone. An important limitation of this study was that it did not take intoconsideration the presence of dehiscence or fenestrations, which could affect the results. Furthermore, the results described in this review are limited to in-vitro studies. The lack of clinical studies relative to this issue is possibly related to the need for a high number of cases to provide statistically significant results. Thus, there is a limitation to conducting this type of study design in agreement with the ALARA principle [16]. However, in a clinical situation, factors related to patient movements could also affect image resolution.
This review aimed to help clinicians to understand the advantages and limitations of the imaging methods currently available for the assessment of peri-implant bone. However, it must be emphasized that the choice of imaging technique dependson the patient`s need, professional preference and the additional benefits provided by the examination. Factors such as radiation dose, costs and individual needs must be taken into considerationin future researches [40].
CONCLUSION
- CBCT showed an acceptable accuracy for assessing peri-implant buccal bone thickness.
- US showed an accuracy similar to that ofCBCT in all evaluated studies.
- Due to the lack of studies in the literature, no conclusion could be drawn with respect to other techniques.
CONSENT FOR PUBLICATION
Not applicable.
STANDARD FOR REPORTING
PRISMA guidelines and methodology were followed.
FUNDING
None.
CONFLICT OF INTEREST
The authors declare no conflict of interest, financial or otherwise.
ACKNOWLEDGEMENTS
Declared none.
Author, Year | Exclusion criteria |
---|---|
Azevedo-Vaz et al 2013 | 3 |
Azevedo-Vaz et al 2013b | 3 |
Azevedo-Vaz et al 2013c | 3 |
Azevedo-Vaz et al 2016 | 3 |
Becker et al 2015 | 2 |
Benic et al 2012 | 4 |
Borg et al 2000 | 3 |
Bousquet et al 2007 | 2 |
Bousquet et al 2008 | 2 |
Bridcut et al 2001 | 2 |
Choi et al 2012 | 3 |
Dave et al 2012 | 3 |
Draenert et al 2007 | 2 |
Duttenhoefer et al 2015 | 2 |
Fienitz et al 2011 | 3 |
Fourmousis et al 1994 | 2 |
Gher et al 1995 | 3 |
Garcia-Garcia et al 2016 | 3 |
Golubovic et al 2012 | 3 |
Gonzales-Garcia et al 2013 | 2 |
Gonzáles-Martin et al 2016 | 3 |
Grondhal et al 1997 | 2 |
Kamburoglu et al 2014 | 3 |
Kavadella et al 2006 | 3 |
Kuhl et al 2015 | 3 |
King et al 2002 | 2 |
Linrachtamorn et al 2000 | 1 |
Ludlow et al 1995 | 2 |
Mangione et al 2013 | 2 |
Matsuda et al 2001 | 2 |
Meijer et al 1993 | 2 |
Meijer et al 1995 | 2 |
Mengel et al 2006 | 3 |
Merheb et al 2015 | 2 |
Miyamoto et al 2011 | 2 |
Ogusanglu et al 2012 | 2 |
Pasquet et al 2009 | 1 |
Pauwels et al 2014 | 2 |
Pinheiro et al 2015 | 3 |
Pinheiro 2015b | 3 |
Raes et al 2013 | 2 |
Rashedi et al 2003 | 2 |
Reddy et al 1994 | 2 |
Sanchez et al 2004 | 2 |
Schliephake et al 2013 | 3 |
Sennerby et al 2001 | 4 |
Sewerin et al 1997 | 3 |
Shokri et al 2015 | 2 |
Sirin et al 2012 | 3 |
Slak et al 2014 | 1 |
Sundén et al 1995 | 2 |
Svenson et al 1996 | 3 |
Van Oossterwyck, et al 2000 | 4 |
Vandeweghe et al 2013 | 4 |
Wang et al 2013 | 3 |
Wang et al 2013b | 1 |
Webber et al 1996 | 2 |
Zechner et al 2003 | 2 |
Dagassan-Berndt et al | 3 |
Tang et al | 3 |
Vidor et al | 3 |
Vidor et al | 3 |
Peterson et al | 3 |
Mercado et al | 3 |
Flügge et al | 3 |
Sheikhi et al | 3 |
Yen et al | 3 |
Chan et al | 3 |
Chan et al | 3 |
Salmon et al | 3 |