All published articles of this journal are available on ScienceDirect.
Masseter Muscle Activity in Track and Field Athletes: A Pilot Study
Abstract
Teeth clenching has been shown to improve remote muscle activity (by augmentation of the Hoffmann reflex), and joint fixation (by decreased reciprocal inhibition) in the entire body. Clenching could help maintain balance, improve systemic function, and enhance safety. Teeth clenching from a sports dentistry viewpoint was thought to be important and challenging. Therefore, it is quite important to investigate mastication muscles’ activity and function during sports events for clarifying a physiological role of the mastication muscle itself and involvement of mastication muscle function in whole body movement. Running is a basic motion of a lot of sports; however, a mastication muscles activity during this motion was not clarified. Throwing and jumping operation were in a same situation. The purpose of this study was to investigate the presence or absence of masseter muscle activity during track and field events. In total, 28 track and field athletes took part in the study. The Multichannel Telemetry system was used to monitor muscle activity, and the electromyograms obtained were synchronized with digital video imaging. The masseter muscle activity threshold was set 15% of maximum voluntary clenching. As results, with few exceptions, masseter muscle activity were observed during all analyzed phases of the 5 activities, and that phases in which most participants showed masseter muscle activity were characterized by initial acceleration, such as in the short sprint, from the commencement of throwing to release in both the javelin throw and shot put, and at the take-off and landing phases in both jumps.
INTRODUCTION
It is quite important to investigate mastication muscles’ activity and function during sports events for clarifying a physiological role of the mastication muscle itself and involvement of mastication muscle function in whole body movement.
Many researches have been done up to now for this issue. One problem that has been encountered in early studies on the masseter muscle activity during sports has been the restriction of movement by the wires of the electromyography used to perform measurements, making its application to the natural movements that occur during the performance of a sport impossible. However, in recent years, wireless and telemeter system electromyography has been developed, and the involvement of masseter muscles in a compound, synchronized physical activities can now be measured. The involvement of the masticatory muscles has also been investigated by a group in sports such as baseball [1], volleyball and handball [2], soccer [3]. All showed clear masticatory muscle activity during powerful batting and pitching [1], and spiking volleyballs, shooting handballs [2]. One-third of athletes studied showed clear masticatory muscle activity when kicking in soccer [3]. A positive correlation between masticatory muscle activity and kicking, spiking, shooting, batting, pitching, and strength activities were reported. It was concluded, in these earlier studies that the masticatory muscles might play an important role in all the sports studied. Furthermore, teeth clenching and mandibular fixation have been reported to affect performance in sports such as weight lifting, where competitors are required to exert maximum isometric muscle contraction throughout the body, and other athletic pursuits requiring slow, isotonic movement [4]. The effects of an occlusal status, clenching, and condylar position on simpler movements requiring back muscle strength [5], elbow flexion [6], and knee extension [7] have also been described.
Running is a basic motion of a lot of sports; however, a mastication muscles activity during this motion was not clarified. Throwing and jumping operation were in the same situation. The purpose of this study was to investigate the presence or absence of masseter muscle activity during track and field events.
MATERIAL AND METHODS
In total, 28 track and field athletes from Nippon Sport Science University, with an average age of 20.0 years and no stomatognathic abnormalities, took part in the study. The objective and techniques of the study were explained in detail to all the athletes before commencement of the experiment, and written consents were obtained. The study protocol was approved by the Tokyo Dental College Ethics Committee (Approval No. 238). The experimental method used and processing of the results adhered to the tenets of the Helsinki Declaration.
The athletes were required to perform the following activities: short sprint (5 males), javelin throw (4 males, 2 females), shot put (2 males, 2 females), long jump (5 males), and high jump (5 males). All phases of the 5 activities were subdivided for detailed analysis. Short Sprint was analyzed in 3 subdivisions: start position, just before the athlete began to raise his lower back from the crouch-start position until initiation of forward movement; start up, between the time the athlete lifted his legs after start-up until the time the legs touched the track; and during the initial acceleration run. Javelin was analyzed in 4 subdivisions: during the approach run; cross step, during the longer, flatter drive step of the leg that takes place after the athlete pushes off with the other leg; throwing step, during a step prior to throwing, when a leg is brought forward to bring the body into the power position; when the javelin is thrown; and reverse, when the other leg is brought forward for foul prevention. Shot put was analyzed in 4 subdivisions: gliding, between preparation and throw, when the athlete lowers the trunk, bends the supporting leg, and advances for the power position and throw; at the time of the throw; after the throw, before the reverse; and reverse, when the athlete straddles a leg for foul prevention. Long jump and high jump were analyzed in 3 subdivisions: during the approach run, during take-off, and at landing. Each athlete was also required to perform a trial activity and a maximum voluntary clenching 3 times before actual measurements.
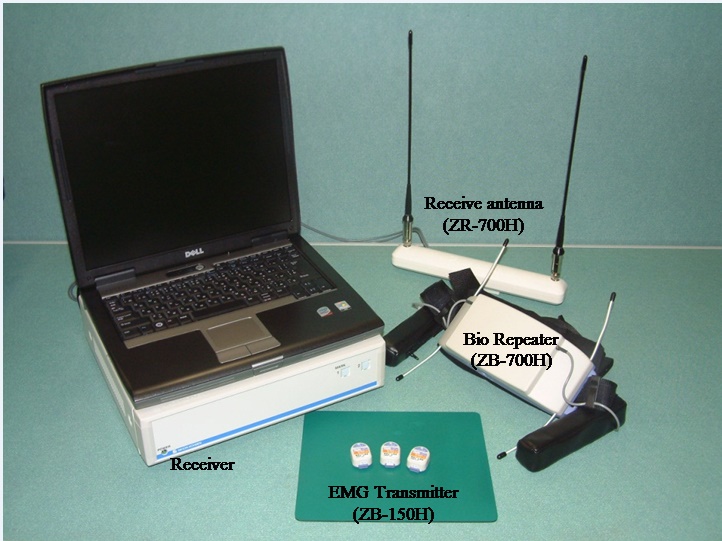
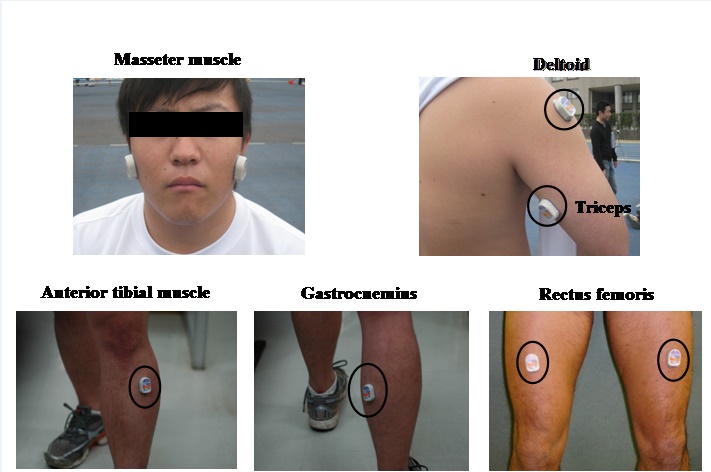
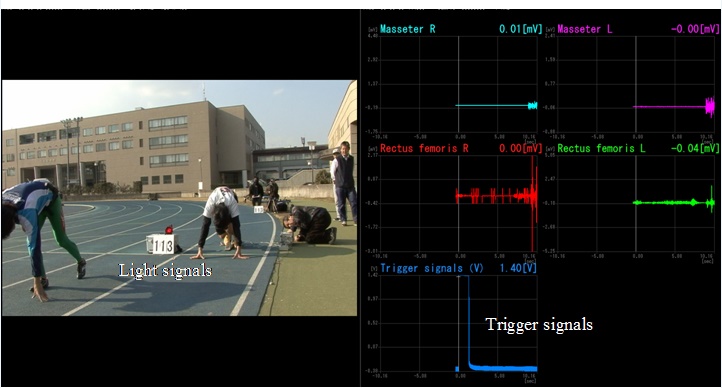
The WEB-7000 Multichannel Telemetry system (NIHON KOHDEN, Japan) was used to monitor muscle activity (Fig. 1). This system primarily consisted of EMG (ZB-150H), EMG transmitters (cordless telemetry electrodes), a BIO Repeater (ZB-700H), receiver antenna (ZR-700H), a receiver, and a personal computer. Electromyograms of the bilateral masseter muscles during and following all activities were obtained: during 60-m sprint, the bilateral rectus femoris; during jumping, the anterior tibial muscles; and during throwing, the deltoids and triceps of the dominant arm, the anterior tibial muscle of the pivotal foot, and contralateral gastrocnemius. Low region cutoff frequency was 30 Hz, high region cutoff frequency was 5 kHz and sampling frequency was 10 kHz (Fig. 2). The electromyograms were synchronized with digital video imaging. In the synchronous display with the Pixel Runner (TELLUSIMAGE, Japan), trigger signals were inputted into the WEB-7000 and light signals into the video image, both by direct current, to synchronize them (Fig. 3). Maximum muscle activity at each phase of activity and maximum voluntary clenching were obtained with the WEB-7000 system. The presence or absence of masseter muscle activity was investigated. Individual values for masseter muscle activity were also converted to a percentage of maximum clenching force to allow for comparison. Yokoyama et al. [8] have reported that the appropriate threshold value of clenching appearance during physical exercise was 15% of masseter muscle activity compared with the activity level at maximum voluntary clenching. Accordingly, we decided to record the presence or absence of masseter muscle activity using this threshold value during each phase of track and field events.
RESULTS
All results for masseter muscle activity are given as a percentage of the individual maximum values obtained as described above.
1. Masseter Muscle Activity in the Short Sprint
Masseter muscle activity was observed in 2 of the 5 athletes (58.33%–90.24%) on the right side and in 2 of 5 (50.63%–82.98%) on the left side, just before the athlete started to raise his/her lower back from the crouch-start position until initiation of forward movement (Fig. 4-1, Table 1) activity was observed in 3 of the 5 athletes (23.85%–97.56%) on the right side and in 3 of the 5 (29.29%–89.36%) on the left side (Fig. 4-2, Table 1) between the time the athlete lifted his/her legs after start-up until the time the legs touched the track; and activity was observed in 4 of the 5 athletes (20.83%–97.56%) on the right side and in 4 of the 5 (19.18%–97.57%) on the left side (Fig. 4-3, Table 1) during initial acceleration. Masseter muscle activity was not recognized after initial acceleration.
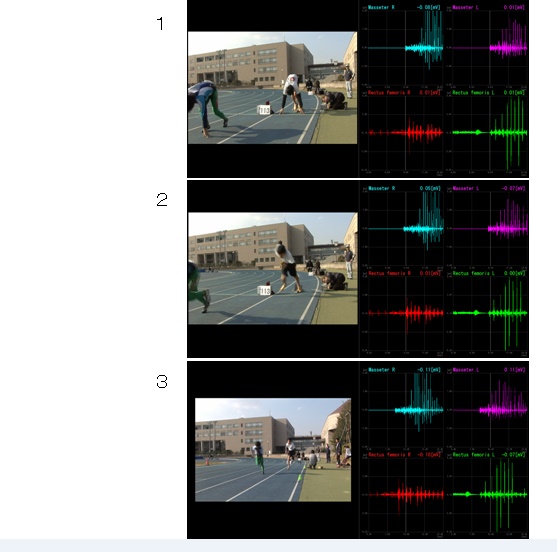
Sub. 1 | Sub. 2 | Sub. 3 | Sub. 4 | Sub. 5 | |||||||
---|---|---|---|---|---|---|---|---|---|---|---|
R/L | R | L | R | L | R | L | R | L | R | L | |
Max. MMA | 1.09 | 0.99 | 0.41 | 0.79 | 1.06 | 0.73 | 0.24 | 0.47 | 0.72 | 0.53 | |
Start position | MEAN | 0.27 | 0.26 | 0.37 | 0.4 | 0.04 | 0.03 | 0.14 | 0.39 | 0.03 | 0.02 |
SD | 0.16 | 0.11 | 0.12 | 0.02 | 0.01 | 0 | 0.04 | 0.03 | 0.01 | 0 | |
% | 14.68 | 11.11 | 90.24 | 50.63 | 3.77 | 4.11 | 58.33 | 82.98 | 4.17 | 3.77 | |
Start-up | MEAN | 0.26 | 0.29 | 0.4 | 0.37 | 0.04 | 0.07 | 0.15 | 0.42 | 0.03 | 0.02 |
SD | 0.05 | 0.05 | 0.11 | 0.04 | 0.01 | 0.05 | 0.03 | 0.05 | 0.01 | 0 | |
% | 23.85 | 29.29 | 97.56 | 46.84 | 3.77 | 9.58 | 62.5 | 89.36 | 4.17 | 3.77 | |
Initial acceleration | MEAN | 0.45 | 0.56 | 0.4 | 0.41 | 0.13 | 0.14 | 0.2 | 0.44 | 0.15 | 0.07 |
SD | 0.03 | 0.07 | 0.11 | 0.01 | 0.01 | 0.05 | 0.05 | 0.06 | 0.02 | 0.04 | |
% | 41.28 | 56.57 | 97.56 | 97.57 | 12.26 | 19.18 | 83.33 | 93.62 | 20.83 | 13.21 |
2. Masseter Muscle Activity in the Javelin Throw
Masseter muscle activity was observed in 2 of the 6 athletes (16.8%–18.8%) on the right side and in 5 of the 6 athletes (16.92%–63.22%) on the left side during the approach run (Fig. 5-1, Table 2). When the athletes stepped forward prior to the cross-step, it was observed in 3 of the 6 athletes (30.83%–78.13%) on the right side and in 3 of the 6 athletes (33.41%–97.07%) on the left side (Fig. 5-2, Table 2); when they stepped forward prior to throwing, it was observed in 5 of the 6 athletes (16.92%–103.13%) on the right side and in all athletes (41.78%–120.92%) on the left side (Fig. 5-3, Table 2). At the time of throwing the javelin, it was observed in all athletes (16.7%–121.88%) on the right side and in 5 of the 6 athletes (17.58%–107.3%) on the left side (Fig. 5-4, Table 2); at the time of reversing for foul prevention, it was observed in 3 of the 6 athletes (23.07%–56.25%) on the right side and in 3 of the 6 athletes (23.43%–30.96%) on the left side (Fig. 5-5, Table 2).
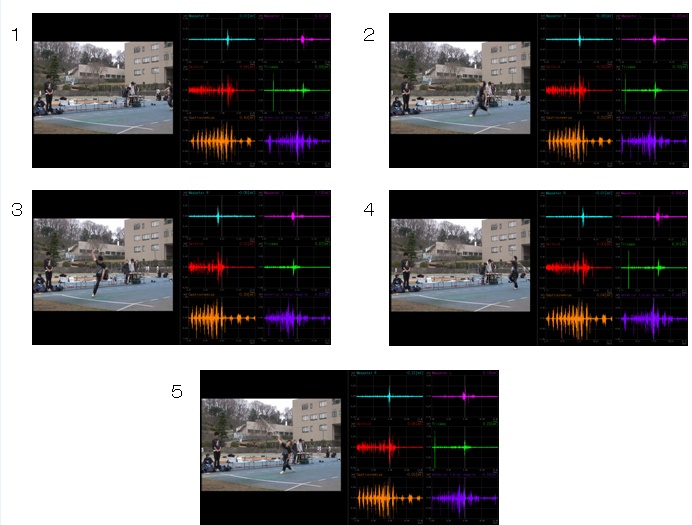
Sub. 1 (F) | Sub. 2 (F) | Sub. 3 (M) | Sub. 4 (M) | Sub. 5 (M) | Sub. 6 (M) | ||||||||
---|---|---|---|---|---|---|---|---|---|---|---|---|---|
R/L | R | L | R | L | R | L | R | L | R | L | R | L | |
Max MMA | 0.32 | 0.5 | 1.13 | 0.48 | 0.65 | 0.92 | 0.84 | 1.18 | 0.37 | 0.24 | 0.53 | 0.68 | |
Approach run | MEAN | 0.32 | 0.05 | 0.14 | 0.04 | 0.16 | 0.04 | 0.14 | 0.06 | 0.07 | 0.1 | 0.11 | |
SD | 0.1 | 0.02 | 0.01 | 0.01 | 0.03 | 0 | 0.02 | 0.06 | 0.04 | 0.02 | 0.03 | ||
% | 63.22 | 4.46 | 29.29 | 6.12 | 16.92 | 4.67 | 11.66 | 16.8 | 27.2 | 18.8 | 17.04 | ||
Cross-step | MEAN | 0.25 | 0.25 | 0.06 | 0.28 | 0.23 | 0.31 | 0.1 | 0.39 | 0.25 | 0.23 | 0.16 | 0.06 |
SD | 0.04 | 0.03 | 0.01 | 0.07 | 0.08 | 0.06 | 0.06 | 0.08 | 0.12 | 0.08 | 0.09 | 0.02 | |
% | 78.13 | 48.71 | 5.36 | 59.21 | 35.38 | 33.41 | 11.28 | 33.53 | 67.48 | 97.07 | 30.83 | 8.74 | |
Throwing step | MEAN | 0.33 | 0.39 | 0.05 | 0.32 | 0.11 | 0.44 | 0.38 | 0.74 | 0.37 | 0.29 | 0.1 | 0.28 |
SD | 0.07 | 0.05 | 0.01 | 0.08 | 0.07 | 0.05 | 0.13 | 0.15 | 0.17 | 0.06 | 0.04 | 0.02 | |
% | 103.13 | 78.33 | 4.59 | 66.95 | 16.92 | 48.35 | 45.1 | 63.32 | 99.19 | 120.92 | 19.55 | 41.78 | |
Throwing | MEAN | 0.39 | 0.54 | 0.19 | 0.04 | 0.37 | 0.16 | 0.5 | 0.56 | 0.44 | 0.22 | 0.32 | 0.27 |
SD | 0.04 | 0.1 | 0.05 | 0 | 0.1 | 0.02 | 0.15 | 0.15 | 0.11 | 0.02 | 0.08 | 0.09 | |
% | 121.88 | 107.3 | 16.7 | 8.37 | 56.92 | 17.58 | 59.81 | 47.57 | 119.51 | 90.8 | 60.53 | 40.44 | |
Reverse | MEAN | 0.18 | 0.14 | 0.17 | 0.11 | 0.15 | 0.07 | 0.08 | 0.09 | 0.09 | 0.07 | 0.07 | 0.04 |
SD | 0.1 | 0.09 | 0.09 | 0.02 | 0.06 | 0.03 | 0.02 | 0.07 | 0.05 | 0.01 | 0.06 | 0.01 | |
% | 56.25 | 27.04 | 14.82 | 23.43 | 23.07 | 7.57 | 9.81 | 8.18 | 23.39 | 30.96 | 12.97 | 6.61 |
3. Masseter Muscle Activity in the Shot Put
From the gliding movement, masseter muscle activity was observed in 4 of the 5 athletes (21.04%–65.58%) on the right side and in all athletes (15.09%–90.91%) on the left side (Fig. 6-1, Table 3). At the time of throwing, it was observed in all athletes on both the right (18.23%–93.29%) and left (36.25%–109.09%) sides (Fig. 6-2, Table 3); after the throw, it was observed in 3 of the 5 athletes (16.32%–23.87%) on the right side and in 2 of the 5 athletes (22.69%–24.53%) on the left side (Fig. 6-3, Table 3); at the time of reversing for foul prevention, it was observed in 2 of the 5 athletes (17.77%–30.27%) on the right side and in 1 of the 5 athletes (30.3%) on the left side (Fig. 6-4, Table 3).
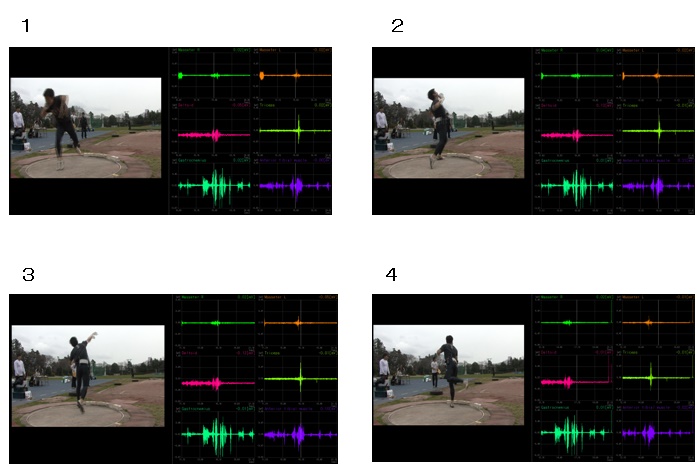
Sub. 1 (F) | Sub. 2 (F) | Sub. 3 (M) | Sub. 4 (M) | Sub. 5 (M) | |||||||
---|---|---|---|---|---|---|---|---|---|---|---|
R/L | R | L | R | L | R | L | R | L | R | L | |
Max MMA | 0.72 | 0.53 | 0.38 | 0.26 | 0.37 | 0.3 | 0.33 | 0.49 | 0.34 | 0.33 | |
Gliding | MEAN | 0.1 | 0.08 | 0.16 | 0.17 | 0.08 | 0.08 | 0.07 | 0.32 | 0.22 | 0.3 |
SD | 0.02 | 0.03 | 0.04 | 0.04 | 0.01 | 0.03 | 0.03 | 0.07 | 0.03 | 0.18 | |
% | 14.5 | 15.09 | 41.38 | 65.77 | 22.49 | 26.67 | 21.04 | 65.31 | 65.58 | 90.91 | |
Throw | MEAN | 0.13 | 0.24 | 0.15 | 0.1 | 0.15 | 0.11 | 0.31 | 0.16 | 0.22 | 0.36 |
SD | 0.04 | 0.01 | 0.01 | 0.01 | 0.01 | 0.04 | 0.05 | 0.1 | 0.14 | 0.1 | |
% | 18.23 | 45.28 | 39.26 | 38.46 | 39.84 | 36.67 | 93.29 | 32.65 | 65.58 | 109.09 | |
After throw | MEAN | 0.11 | 0.13 | 0.09 | 0.06 | 0.04 | 0.03 | 0.06 | 0.04 | 0.06 | 0.04 |
SD | 0.03 | 0.04 | 0.04 | 0.02 | 0.02 | 0.01 | 0.03 | 0.03 | 0.02 | 0.01 | |
% | 14.5 | 24.53 | 23.87 | 22.69 | 9.76 | 10 | 18.6 | 8.16 | 16.32 | 12.12 | |
Reverse | MEAN | 0.04 | 0.06 | 0.07 | 0.03 | 0.03 | 0.02 | 0.03 | 0.02 | 0.1 | 0.1 |
SD | 0.01 | 0.01 | 0.06 | 0.01 | 0.02 | 0 | 0 | 0.01 | 0.08 | 0.08 | |
% | 5.25 | 11.32 | 17.77 | 11.15 | 8.94 | 6.67 | 8.84 | 4.08 | 30.27 | 30.3 |
4. Masseter Muscle Activity in the Long Jump
Masseter muscle activity was observed in 3 of the 5 athletes (26.43%–120.59%) on the right side and in all athletes (16.32%–121%) on the left side during the approach run (Fig. 7-1, Table 4). At take-off, it was observed in all athletes on both the right (16.93%–123%) and left (36.49%–150.16%) sides (Fig. 7-2, Table 4). At landing, it was observed in 3 of the 5 athletes (19.72%–59.63%) on the right side and in all athletes (20.34%–51.41%) on the left side (Fig. 7-3, Table 4).
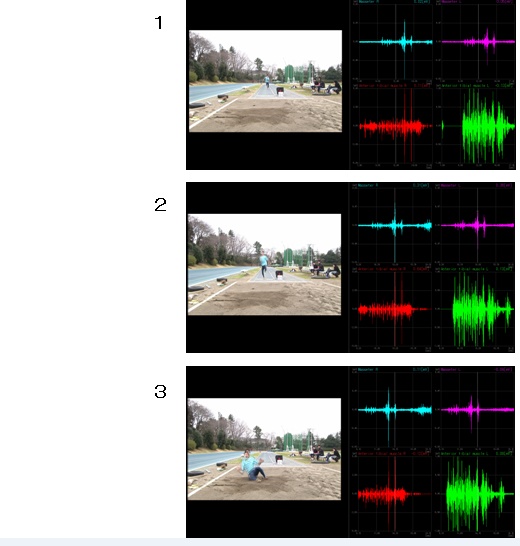
Sub. 1 | Sub. 2 | Sub. 3 | Sub. 4 | Sub. 5 | |||||||
---|---|---|---|---|---|---|---|---|---|---|---|
R/L | R | L | R | L | R | L | R | L | R | L | |
Max MMA | 1.05 | 0.77 | 1.04 | 1.01 | 1.4 | 0.64 | 0.37 | 0.32 | 0.65 | 0.63 | |
Approach run | MEAN | 0.11 | 0.13 | 0.28 | 0.34 | 0.22 | 0.22 | 0.45 | 0.39 | 0.17 | 0.15 |
SD | 0.03 | 0.02 | 0.04 | 0.09 | 0.02 | 0.06 | 0.04 | 0.03 | 0.05 | 0.06 | |
% | 10.08 | 16.32 | 26.68 | 33.63 | 14.43 | 34.38 | 120.59 | 121 | 26.43 | 23.48 | |
Take-off | MEAN | 0.69 | 0.51 | 0.58 | 0.37 | 0.24 | 0.29 | 0.46 | 0.48 | 0.18 | 0.29 |
SD | 0.12 | 0.17 | 0.11 | 0.01 | 0.06 | 0.05 | 0.18 | 0.02 | 0.06 | 0.15 | |
% | 65.21 | 66.45 | 55.47 | 36.49 | 16.93 | 45.31 | 123 | 150.16 | 27.2 | 45.85 | |
Landing | MEAN | 0.12 | 0.16 | 0.21 | 0.22 | 0.17 | 0.27 | 0.22 | 0.16 | 0.27 | 0.29 |
SD | 0.05 | 0.1 | 0.02 | 0.04 | 0.01 | 0.36 | 0.07 | 0.06 | 0.07 | 0.08 | |
% | 11.03 | 20.34 | 19.72 | 21.6 | 12.29 | 42.19 | 59.63 | 51.41 | 41.58 | 47.13 |
5. Masseter Muscle Activity in the High Jump
Masseter muscle activity was observed in 3 of the 5 athletes (19.87%–27.36%) on the right side and in 4 of the 5 athletes (15.75%–50%) on the left side during the approach run (Fig. 8-1, Table 5). At take-off, it was observed in all athletes on both the right (27.61%–108.8%) and left sides (27.86%–63.64%) (Fig. 8-2, Table 5). At landing, it was observed in all athletes on both the right (21.34%–51.63%) and left sides (23.14%–47.08%) (Fig. 8-3, Table 5).
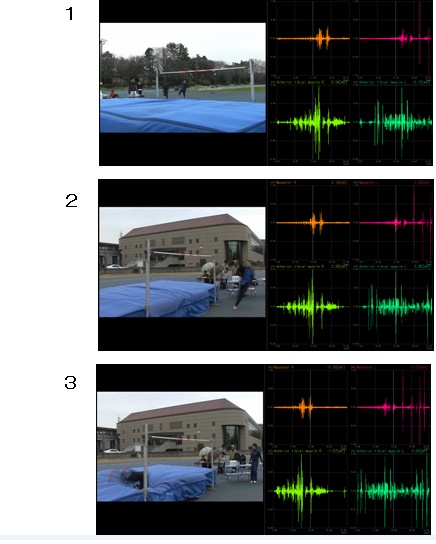
Sub. 1 | Sub. 2 | Sub. 3 | Sub. 4 | Sub. 5 | |||||||
---|---|---|---|---|---|---|---|---|---|---|---|
R/L | R | L | R | L | R | L | R | L | R | L | |
Max MMA | 1.05 | 0.77 | 1.04 | 1.01 | 1.4 | 0.64 | 0.37 | 0.32 | 0.65 | 0.63 | |
Approach run | MEAN | 0.11 | 0.13 | 0.28 | 0.34 | 0.22 | 0.22 | 0.45 | 0.39 | 0.17 | 0.15 |
SD | 0.03 | 0.02 | 0.04 | 0.09 | 0.02 | 0.06 | 0.04 | 0.03 | 0.05 | 0.06 | |
% | 10.08 | 16.32 | 26.68 | 33.63 | 14.43 | 34.38 | 120.59 | 121 | 26.43 | 23.48 | |
Take-off | MEAN | 0.69 | 0.51 | 0.58 | 0.37 | 0.24 | 0.29 | 0.46 | 0.48 | 0.18 | 0.29 |
SD | 0.12 | 0.17 | 0.11 | 0.01 | 0.06 | 0.05 | 0.18 | 0.02 | 0.06 | 0.15 | |
% | 65.21 | 66.45 | 55.47 | 36.49 | 16.93 | 45.31 | 123 | 150.16 | 27.2 | 45.85 | |
Landing | MEAN | 0.12 | 0.16 | 0.21 | 0.22 | 0.17 | 0.27 | 0.22 | 0.16 | 0.27 | 0.29 |
SD | 0.05 | 0.1 | 0.02 | 0.04 | 0.01 | 0.36 | 0.07 | 0.06 | 0.07 | 0.08 | |
% | 11.03 | 20.34 | 19.72 | 21.6 | 12.29 | 42.19 | 59.63 | 51.41 | 41.58 | 47.13 |
DISCUSSION
In sports, it is necessary to consider the effectiveness of skeletal muscle activity itself and the importance of balanced adjustment ability in equilibrium function. It is widely known that the ability of skeletal muscles to produce sufficient force at a high velocity is crucial for success in sprint [9] as well as strength and power performance [10]. Balance adjustment also plays an important role in orthostatic and dynamic postural control, influencing an individual’s abilities to perform activities of daily living [11-13], participate in sports [14-19]. In many types of physical activity for high performance requires the stability of static posture, the so-called “setup,” which is essential for accomplishing the dynamic motion required in terms of smoothness of movement, appropriate strength, and high speed. Therefore, attention has been focused on the effect of skeletal muscle activity and balance control in sports as and activities of daily living.
Therefore, several studies have investigated the effects of oral function on the performance in sports and whole body activities. Teeth clenching and mandibular fixation have been reported to affect performance in sports such as weight lifting, where competitors are required to exert maximum isometric muscle contraction throughout the body, and other athletic pursuits requiring slow, isotonic movement [4]. The effects of an occlusal status, clenching, and condylar position on simpler movements requiring back muscle strength [5], elbow flexion [6], and knee extension [7] have also been described. Similarly, the role of clenching is important in activities requiring maintenance of a static posture, such as archery and shooting [20, 21]. Voluntary teeth clenching contributes to the stabilization of a postural stance transiently disturbed by electrical stimulation [22] .
Here, we would like to think about possible meanings of masseter muscle activity during sports. Effects on an equilibrium function and a facilitation of remote muscle activity (the Jendrassik manoeuvre) are potencies. It has been suggested that an appropriate masseter muscle activity facilitate the maintenance of posture [1, 3, 8, 23]. To perform any kind of physical activity at a high level of efficiency, it is crucial that an athlete be able to maintain the requisite posture, whether static or dynamic, and move with the necessary degree of smoothness, speed, and strength. Both static and dynamic movements are required to act in concert to achieve the kinds of movements involved in physical exercise [24]. In the fixation of the head, stabilizations of sight line and balance of the body could be obtained. As a result, it is thought that accurate sports motion can be done [2]. And, the possible factors of effects on these functions are;1.Masseter muscle activity plays an important role in antigravity muscles [25]. 2.The masseter muscle activity is involved in, facilitating contraction of the extensor and flexor muscles, enhancing muscle activity in non-reciprocity and fixation of the joints [26-30]. Head stability depends largely on clenching or masticatory and neck muscles [31]. Takada [32] found that reciprocal Ia inhibition of the crural muscle was reduced during voluntary jaw clenching and suggested that jaw clenching contributes to stability by increasing the stiffness of the joint. 3. The Hoffmann reflex is an index that shows excitatory of motoneuron of spinal cord. This reflex seems to be involved in activity facilitation of remote muscle. Miyahara et al. [29] have reported that the soleus H reflex is facilitated in association with voluntary teeth clenching. This facilitation means strengthen masseter muscle activity made the Jendrassik manoeuvre strong and the excited condition of remote muscle strong. It seems that these factors take part in various sports events.
In this study, masseter muscle activity was observed during all the analyzed phases of the 5 activities in any subdivisions. We would like to see as an each event, and to add some consideration respectively.
Masseter muscle activity was observed in a short sprint in subdivision of during the before start, starting and initial acceleration. Stabilization of posture is important during the crouching start. Before the start, the center of gravity should be stabilized, and initial velocity could be gained when the athlete kicks away from the starting block strongly. A forward-bending posture and low center of gravity between starting and initial acceleration should be maintained, and further speed could be gained through a strong ground reaction. At this point, the force of the ground reaction increases in the horizontal as well as the vertical direction, when, at its maximum, it is believed to be 4–5 times the body weight of the athlete [33], and it is necessary to maintain an adequate posture against this force to achieve higher performance. This is particularly the case during initial acceleration, when a strong reaction force from the track surface is transmitted to the body. At this time, masseter muscle activity helps to stabilize the joints and posture, thus decreasing energy loss through the wobbling of the body. Masseter muscle activity disappeared after initial acceleration through the sprint, because, by this time, only smooth and relaxed movements are required. This result supports an opinion of masseter muscle activity is not as relevant in sports requiring relaxed and smooth movements, such as sprinting [34].
Masseter muscle activity was observed during the javelin throw, possibly because of the maintenance of the center of gravity and body axis during the approach run to stabilize the javelin at the point of release. This activation might be involved not only in gripping and the muscular tension in the arms, lower back, and legs during the throw but also in deceleration of each joint at the termination of the movement. These results are related to results of a strong handball shot with jump that a mastication muscle activity was started at a time of jump launches and completed backswing (these two motions occurred simultaneously), and it was disappeared at a time of ball release or following swing complete [2].
Masseter muscle activity was observed during the shot put, where the center of gravity is kept low during the gliding movement Masseter muscle activity here comes into play at the moment the athlete shifts his/her weight onto the pivotal leg to obtain projection and again after release, as in the javelin throw. These results are related to results of a volleyball strong spike that Mastication muscle activity was shown at a lowered body position for a preparation for jump and the strongest activity was shown momentarily of the jump [2]. The masseter muscle activity might be involved when a ground reaction is required to vertically redirect and convert force to obtain.
Masseter muscle activity was observed during the long jump, immediately before take-off. When the athlete jumps, horizontal speed gained during the approach run is converted into force in the vertical direction by ground reaction. Masseter muscle activity appears to reinforce the ground reaction required for this conversion [35]. Masseter muscle activity was also observed just before and during landing, whereas the upper body is maintained in a perpendicular orientation. This activity might enhance the skip distance by relaxing both knees and allowing the upper body to move sideways freely while landing, and may also act as a defensive reflex against shock. Moreover, masseter muscle activity while the athlete is in midair may help stabilize a balance and maintain the upper body in the perpendicular position until landing.
Masseter muscle activity during the approach run in the high jump is somewhat complicated, because a curve is drawn as the body axis is slanted inward. Activity occurs at the time of this inward-turning movement. At take-off, this activity enhances rotation of the body axis as the opposite-side knee is pulled inward. This rotation force is vertically redirected to decrease body sway and decrease loss of force [33]. This rotation force might activate muscles throughout the body, including the masseter muscle.
Voluntary masseter muscle activity observed in many events in many phases are thought to be represent a form of body control that is acquired through training (expected body control, which is a feed-forward mechanism of synergistic activity) to improve athletic performance [7]. Therefore, knowledge of the role of masseter muscle activity would be of considerable importance in improving sports performance. Hence, an elucidating the characteristics, effects, and meaning of masticatory muscle activity during various types of sports should be investigated.
Within the limitations of this study, it was not possible to analyze the complex movements involved in the targeted sports in detail. Further study is needed to clarify the characteristics, effectiveness, and significance of masticatory muscle activity during the performance of various sports. Moreover, such study must also take into account its involvement in other sports, individual differences, especially in athletes’ ability levels, and the effects of oral appliances. However, our results still have strength in clarifying, for the first time, the facts relative to the presence of masseter muscle activity during track and field events.
CONCLUSION
In this study, we investigated the presence or absence of masseter muscle activity during the performance of 5 track and field events. The selected events were the short sprint, javelin throw, shot put, long jump and high jump. The results showed that masseter muscle activity were observed during all analyzed phases of the 5 activities, even if not in all the athletes, and phases in which the majority of athletes showed masseter muscle activity included initial acceleration at the start of short sprints, from the commencement of throwing to release in both the javelin throw and the shot put, and at the take-off and landing phases in jumping.
CONFLICT OF INTEREST
The authors confirm that this article content has no conflict of interest.
ACKNOWLEDGEMENTS
The authors would like to thank all study participants for their kind cooperation in this study. Especially, Koji Otsu for his technical assistances.